Abstract
Objective
To assess the technical feasibility and complications of radiofrequency ablation (RFA) using a monopolar wet electrode for the treatment of inoperable non-small cell lung malignancies.
Materials and Methods
Sixteen patients with a non-small cell lung malignancy underwent RFA under CT guidance. All the patients were non-surgical candidates, with mean maximum tumor diameters ranging from 3 to 6 cm (mean: 4.6 ± 1.1 cm). A single 16-gauge open-perfused electrode with a 2 cm exposed tip was used for the procedure. A 0.9% NaCl saline solution was used as the perfusion liquid with the flow adjusted to 30 mL/h. The radiofrequency energy was applied for 10-40 minutes. The response to RFA was evaluated by performing contrast-enhanced CT immediately after RFA, one month after treatment and then every three months thereafter.
Results
Technical failure was observed in six (37.5%) of 16 patients: intractable pain (n = 2) and non-stop coughing (n = 4). The mean follow-up interval was 15 ± 8 months (range: 9-31 months). The mean maximum ablated diameter in the technically successful group of patients ranged from 3.5 to 7.5 cm (mean 5.1 ± 1.3 cm). Complete necrosis was attained for eight (80%) of 10 lesions, and partial necrosis was achieved for two lesions. There were two major complications (2/10, 20%) encountered: a hemothorax (n = 1) and a bronchopleural fistula (n = 1).
Radiofrequency ablation (RFA) can offer physicians an attractive alternative for the treatment of patients with an inoperable lung malignancy. The advantages of this technique include the ability to preserve more lung tissue than is possible with a surgical resection, and there is reduced morbidity compared with that associated with surgery. This is particularly important for patients suffering from lung cancer as they usually have accompanying chronic obstructive pulmonary disease. Some researchers (3-5) have suggested that RFA can be a minimally invasive method for treating patients with inoperable lung cancer. It has been reported that the incidence of complete coagulation necrosis of a lung malignancy after RFA as seen at the follow-up computed tomography (CT) was 38-76% and the rate of complications, such as a pneumothorax and hemoptysis, was 10-91% (1-9).
The major limitations for performing RFA for a lung malignancy have been the incapability of the technique to produce a coagulation area that is sufficiently large to include lung malignancies > 3 cm along with a reasonable safety margin (5-10 mm) of the normal tissue (1-6). Lee et al. (4) have reported that complete necrosis was attained 100% for tumors < 3 cm in size but complete necrosis was obtained in only six of 26 larger tumors. In an attempt to overcome this limitation of the ablated zone size, several studies have demonstrated that saline-enhanced RFA has showed superior performance in creating coagulation necrosis in the liver and lung (10-15).
However, there are few reports evaluating saline-enhanced RFA for a lung malignancy in humans. This report describes our initial experience using a CT-guided, transthoracic, RFA using a monopolar wet electrode for the treatment of inoperable non-small cell lung cancer (NSCLC). This study has focused on the technical success and complications associated with RFA using the monopolar wet electrode for the treatment of inoperable NSCLC.
Patients: The institutional ethics committee at our hospital approved this study, and written informed consent was obtained from every patient prior to treatment. From December 2003 to June 2004, 16 patients (mean age: 65.6 years, age range: 43-77 years) with NSCLC underwent RFA using a monopolar wet electrode under CT scan guidance. For all patients, the diagnosis of NSCLC was obtained following a percutaneous transbronchial needle biopsy and bronchoscopic biopsy. The patients were not candidates for surgery because of the advanced stage of their disease along with a poor cardiopulmonary status (n = 12) or coexistent medical problems (n = 2) or refusal to undergo surgery (n = 2). The patients that were selected for RF therapy met the following criteria: (a) they had histologically proven NSCLC, (b) no coagulation disorders, (c) tumor size was less than 6 cm, and (d) a single primary plmonary tumor was present.
The tumors ranged in diameter from 3 to 6 cm (mean, 4.6 ± 1.1 cm). There were two, nine, and five tumors < 3 cm, 3-5 cm, and > 5 cm, respectively. Two (12.5%) patients had stage IB lung cancer and 14 patients (87.5%) had unresectable stage III or IV lung cancer. Fourteen patients had a forced expiratory volume in 1 second that measured < 1 L. There were two separate justifications for performing RFA. In the first patient group, RFA was applied to achieve complete ablation in two patients. In this group, the patients had stage I lung cancer but despite our best medical advice that they undergo surgical resection, they adamantly refused surgery (n = 2) due to old age. In the second group, 14 patients underwent RFA to achieve volume reduction of the primary tumor to prevent complications or direct invasion due to the bulk mass. Nine of 14 patients received systemic chemotherapy after RFA regardless of technical failure due to the presence of metastatic lymph nodes or a distant metastasis. The remaining patients strongly refused systemic chemotherapy.
Radiofrequency Technique: Before the procedure, all the patients fasted for six hours and blood coagulation parameters were checked. The most appropriate approach to the lesion was selected based on the tumor location determined by CT. The RFA procedure was performed in a hospital CT room by a single experienced chest radiologist, a technician and a nurse. RFA was always performed with the patient under analgesia, which was achieved with an intravenous administration of 50-100 µg of fentanyl citrate (Fentanyl, Myengmun, Seoul, Korea). Vital signs were monitored continuously during the procedure and for one hour afterward.
Two grounding pads were established near the electrode. All patients underwent a chest CT scan (Somatom Plus 4, Siemens, Erlangen, Germany) immediately before RFA. The selected axial images were obtained from within the area of interest. The image slices were 3 mm thick.
Radiofrequency ablation was planned in an attempt to avoid the blood vessels, bronchi, blebs and fissures. After cleaning the skin with iodine and alcohol, local anesthesia was achieved by a subcutaneous injection of 1% lidocaine from the skin down to the pleura along a predetermined puncture line. A 16-gauge single open-perfused electrode (Elektrotom HiTT 106, Berchtold Medizin-Eelektronik, Tuttlingen, Germany) was then carefully introduced into the tumor under CT guidance. In order to minimize the incidence of a pneumothorax, an attempt was made to limit the number of times the electrode passed through the pleura to a single insertion. If an additional ablation was required, the position of the needle within the tumor was changed by withdrawing it into the superficial tissue, changing the angle, and then inserting it back into the target without completely withdrawing the electrode out of the pleura.
The RFA system used for monopolar RFA consisted of a 375-kHz generator (Elektrotom HiTT 106, Berchtold Medizin-Eelektronik) that was set to 20-30 watts, and a single 16-gauge open-perfused electrode in the form of a needle with a 2 cm exposed tip. A 0.9% NaCl saline solution was used as the perfusion liquid with the flow adjusted to 30 mL/h. RF energy was applied for 5 minutes, and the RF was then turned off for 2-min intervals according to the manufacturer's recommendations. If the tumor size was below 3 cm, RFA was applied for 10-15 minutes. If the tumor size was 3-5 cm, RFA was applied for 15-30 minutes. If the tumor size was above 5 cm, RFA was applied for more than 30 minutes (15). The impedance was stabilized within a range of 100 to 350 Ω using a control mechanism. RFA was finished after an envelope of peripheral ground glass opacity appeared (at least > 5 mm) around the tumor as seen on the lung CT setting and when the temperature of the ablated zone exceeded 60℃.
Imaging and assessment of the treatment response: Technical success was defined as the completion of the RFA without stopping the procedure because of a side effect or complication and elimination of enhancing areas (more than 90%) as seen on the post-contrast-enhanced follow-up CT images compared with the post-contrast scan before RFA (16). Non-enhanced CT scans were obtained before and during the RFA procedures, and contrast-enhanced CT after injecting the contrast medium (Ultravist®, Schering, Berlin, Germany) was performed immediately after completing the RFA. The contrast-enhanced CT scans were compared with the pre-procedural contrast-enhanced CT scans, and the treatment response was then assessed. After administration of the contrast agent, the previously enhancing area that was now a non-enhancing area of the tumor was considered to represent necrosis induced by the RF energy. Any region in the tumor that showed continued enhancement was considered as residual tumor. Complete ablation was considered when the non-enhancing area at the site of the tumor had a diameter equal to or larger than the treated tumor, as noted on the immediate follow-up CT scan. Partial ablation was considered achieved when a residual enhancement zone was detected on the immediate follow-up image (1). If partial ablation occurred after the saline-enhanced RFA, we recommended additional therapy such as chemotherapy or radiation therapy. On immediately follow up CT, we evaluated shape (round or not) and the margin of the ablated zone (smooth or not). The types of complications encountered were also noted according to the Society of Interventional Radiology Clinical Practice Guideline (16). Follow-up CT was performed at 1-month and at 3-month intervals thereafter.
All the CT images were archived using a picture archiving and communication system (PACS, m-view TM; Marotech, Korea). The maximum diameter of each ablated zone (ablated tumor with enveloped ground glass opacity) was calculated for all the sections and the mean value was calculated after measuring the size of the zone on each section on the CT scans (lung setting) that had been performed before and immediately after RFA. The diameters of the ablated zone before and after RFA were compared using a paired-sample t tests using SPSS 9.0 software (SPSS Inc., Chicago, IL).
Table 1 summarizes the results of RF treatment according to the patient characteristics and tumor size. The mean follow-up interval of this study was 15 ± 8 months (range: 9-31 months). Technical failure was observed in six (37.5%) of 16 patients due to side effects during the procedure: cough (n = 4) and uncontrolled pain (n = 2). The mean number of needle electrode insertions into the tumor mass was 1.5 (range: 1-2). The duration of the ablation therapy ranged from 10 to 40 minutes (mean 22.5-7.7 minutes).
The changes in the tumor size were highly significant for predicting treatment response. Table 2 summarizes the results of RF therapy according to tumor size. In the technically successful group of patients (n = 10), the mean maximum diameter of the tumor before RFA and the mean maximum diameter of the ablated zones after RFA were 43.6 ± 11.3 and 51 ± 13.5 mm, respectively. There were statistically significant differences between the mean maximal diameter of the tumor before RFA and of the ablated zone after RFA (p < 0.05). Complete ablation was attained in eight (80%) of 10 lesions (Fig. 1), and partial ablation was achieved in two (20%) of 10 lesions (Fig. 2). Complete ablation (100%) was achieved for all tumors with a mean diameter < 3 cm. The mean maximum diameter of the tumor before RFA and the mean maximum diameter of the ablated zones after RFA as seen on the last follow-up CT were 4.3 cm ± 1.1 and 3.7 cm ± 2.3, respectively. On the immediate follow-up CT, it was believed that two patients achieved partial ablation after RFA. One patient had a recurrence after 24 months, but one patient showed a decreased size of the primary lung mass on the 31-month follow-up CT, and it was thought that this patient had achieved complete ablation. On the follow-up CT, two of ten patients had a recurrence, and the remainder of the patients showed no change or a decreased size of the primary lung mass. On the immediate CT, a round shape of the ablated zone was seen in nine (90%) of 10 patients and a smooth margin of the ablated zone was also seen in nine (90%) of 10 patients.
Table 3 summarizes the complications after RF therapy. There were two major complications (2/10, 20%): a hemothorax (n = 1) and a bronchopleural fistula (n = 1). There were five minor complications (5/10, 50%): pneumothoraces that required no treatment (n = 2), pleural effusion (n = 2), and mild hemoptysis (n = 1). The bronchopleural fistula occurred for a subpleural tumor that was treated with two electrode insertions. Two patients (20%) underwent a thoracostomy and surgery after RFA. The complications in the remaining patient required no further treatment even though hospital discharge was delayed. Side effects included pain (n = 6) and cough (n = 6).
Until a few years ago, conventional RF treatment performed with a monopolar or expandable electrode was capable of producing thermal necrosis in lung lesions < 3 cm in diameter. Although lesions > 3 cm in diameter can be treated with multiple overlapping zones of necrosis, this task demands a repeated procedure, which can create more complications. Moreover, the likelihood of a local recurrence is directly related to the treatment of larger tumors. Consequently, the maximum size of the tissue area that can be ablated with a single treatment is of paramount importance because it directly determines the maximum tumor size that can be treated during a single RF application, the number of RF applications required, and the likelihood of achieving a complete tumor treatment (1-9).
Monopolar, dry type RFA and bipolar multiprobe array systems have been used. However, these techniques are not very satisfactory, even though using a power output above 60-70 W resulted in a larger ablated zone. Moreover, it is often difficult to ensure a proper probe configuration when inserting multiple probes into tumors > 3 cm in diameter. As a result, regions of histological viable residual tumors have sometimes been observed after RF treatment of lesions > 3 cm in diameter. In this study, RFA using a monopolar wet electrode was able to produce a coagulation necrosis zone with a maximum diameter of 5 - 7 cm after one or two electrode insertions if the procedure was technically successful. With this technique, it was found that a saline injection could enlarge the effective surface area of the RF electrode. The monopolar wet electrode is easier to handle than multiple probes for achieving coverage of the ablated zone (1, 3-5, 11-23).
Livraghi et al. (24) suggested that a continuous infusion of 1 mL/min saline during ablation resulted in necrotic lesions up to a maximum of 3.9 cm in diameter. Continuous saline infusion through a monopolar electrode increases the efficacy of RF ablation. Three possible effects might explain the increase in the volume of necrosis achieved with continuous saline infusion. These effects include (a) enlargement of the effective surface area of the RF electrode, (b) improved patient tolerance of the increased generator output due to tissue cooling or decreased tissue impedance, and (c) diffusion of boiling saline into the tissues (24, 25). In this study, an attempt was made to infuse 0.9% saline at a rate of 0.5 ml/min during ablation, which resulted in an ablated zone up to a maximum of 7.5 cm in diameter after inserting the electrode twice. It is suggested that increased coagulation can be achieved in fewer treatment sessions with continuous saline infusion as compared with the use of conventional RFA. The areas of coagulation were sometimes irregular using this infused saline technique. Livraghi et al. (24) suggested that the irregular shape of the ablation zones created with a continuous saline infusion might be due to two factors: the uneven diffusion of saline and the differential diffusion of heated saline through the tissue, which leads to necrosis along the diffusion path. However, in our study, we found different results for the shape of ablation zone. A regular round shape and smooth ablated margin was seen in nine of 10 patients that had undergone RFA with technical success. We suggest that slow perfusion, such as the infusion of 0.9% saline at a rate of 0.5 ml/min during ablation does not cause an irregular shape of the ablated zone.
The rates of major complications were acceptable, and similar to the rates associated with the dry type RFA. Two major complications (2/10, 20%) were observed. One hemothorax occurred in a patient with a central tumor and one bronchopleural fistula occurred in another patient. These problems were due to the repeated insertions near the pleura. Although the reported rate of pneumothorax in a larger series varied from between 5% and 30% after a percutaneous needle biopsy, incidences as high as 60% have been reported (26, 27). Lee et al. (4) reported pneumothoraces in 30% of patients (9/30) after RF ablation when using a dry-type monopolar electrode. In this study, pneumothoraces was observed in 20% (2/10) of patients after RF ablation. The lower incidence of a pneumothorax after performing RFA using the monopolar wet electrode was related to the number of electrode insertions.
Although the incidence of a pneumothorax was low, side effects such as pain and cough during RFA were common. Six (37.5%) of 16 patients complained of pain despite the administration of analgesia. These patients had a peripheral tumor within 3 cm from the parietal pleura. For this reason, it was determined that a power output < 30 W was adequate for the peripheral tumors during RFA. Two of these patients stopped the RFA procedure before adequate treatment had been completed because of uncontrolled pain despite the power being set below 30 W. It is suggested that saline-enhanced RFA should not be performed on a subpleural mass because a low power output needs to be selected because of intolerable pain. Lee et al. (28) reported that pleural effusion might occur because of spillage of hot NaCl solution into the pleural cavity while instilling the solution. We thought that the intractable pain might be caused by back flow of hot saline toward the pleura. Six (37.5%) of 16 patients suffered from cough when the rate of saline infusion was approximately 30 ml/hour. In this situation, we hesitated in deciding whether to continue RFA or to stop the procedure. As a result, two patients underwent successful RFA after repositioning the electrode. However, four patients stopped the RFA due to nonstop coughing. These patients were in the prone position and the tumors had cavitations or there was an open bronchus. We suggest that infused saline might have flowed from the cavitary lung mass or open bronchus to the proximal bronchus and this situation may occur with persistent coughing during saline-enhanced RFA. Hence, the use of RFA with a monopolar wet electrode is not recommended for lung cancer with cavitations or if it is near an open bronchus.
The study has some limitations. First, there is the lack of histopathological proof of the completeness of coagulation necrosis, as other researchers have provided (3-5). However, it has been clearly demonstrated in previous studies that a contrast-enhanced CT scan could effectively reveal the extent of the coagulation necrosis induced by RF ablation. In addition, most of the complete ablation on the immediately follow-up CT showed no change or a decreased size of the ablated zone on the last follow-up CT (1-8). A second limitation is the number of study participants was small and we thought that the number of participants should be increased to evaluate further the therapeutic response in the future.
In conclusion, despite the use of a lower power output, RFA using a monopolar wet electrode can achieve larger ablated zones and a lower incidence of pneumothorax. However, this technique showed a high rate of technical failure due to side effects such as pain and cough during the RFA procedure. There were also limitations in achieving complete necrosis due to the irregular shape of the created ablation zones, as shown on the follow-up CT.
Figures and Tables
Fig. 1
73-year-old woman with lung cancer (adenocarcinoma) in the right middle lobe.
A. Before radiofrequency ablation, contrast-enhanced CT scans showed 3-cm, triangular-shaped, enhanced mass in right middle lobe of lung.
B. Monopolar electrode was inserted once within lung mass.
C. Immediately after radiofrequency ablation, ablated zone showed almost no enhancement on contrast-enhanced CT scans and this was judged as complete ablation.
D. Two years later, contrast-enhanced CT scans revealed small subpleural nodule, such as fibrotic nodule, at previous ablated zone.
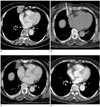
Fig. 2
40-year-old man with lung cancer (adenocarcinoma) in right upper lobe. After undergoing radiofrequency ablation, patient received repeated chemotherapy and radiotherapy as treatment response was only partial ablation.
A. Before radiofrequency ablation, contrast-enhanced CT scans showed 6 cm, lobulated contoured, enhanced mass in right upper lobe of lung.
B. During radiofrequency ablation, monopolar electrode was inserted twice into center of mass.
C. Immediately after radiofrequency ablation, contrast-enhanced CT scans showed peripheral, mildly enhanced rim outside ablated zone of lung cancer (arrow).
D. Ten months later, size of ablated zone decreased in diameter (3 cm) and radiation pneumonia in right upper lobe was observed on contrast-enhanced CT. However, patient died from acute respiratory distress syndrome.
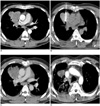
Table 1
Clinical Characteristics of 16 Patients with Non-Small Cell Lung Cancer Treated with Saline-enhanced Radiofrequency Ablation Using Monopolar Wet Electrode
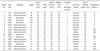
References
1. Jin GY, Lee JM, Lee YC, Han YM, Lim YS. Primary and secondary lung malignancies treated with percutaneous radiofrequency ablation: evaluation with follow-up helical CT. AJR Am J Roentgenol. 2004. 183:1013–1020.
2. Jin GY, Lee JM, Lee YC, Han YM. Acute cerebral infarction after radiofrequency ablation of an atypical carcinoid pulmonary tumor. AJR Am J Roentgenol. 2004. 182:990–992.
3. Belfiore G, Moggio G, Tedeschi E, Greco M, Cioffi R, Cincotti F, et al. CT-guided radiofrequency ablation: a potential complementary therapy for patients with unresectable primary lung cancer - a preliminary report of 33 patients. AJR Am J Roentgenol. 2004. 183:1003–1011.
4. Lee JM, Jin GY, Goldberg SN, Lee YC, Chung GH, Han YM, et al. Percutaneous radiofrequency ablation for inoperable non-small cell lung cancer and metastases: preliminary report. Radiology. 2004. 230:125–134.
5. Steinke K, King J, Glenn DW, Morris DL. Percutaneous radiofrequency ablation of lung tumors with expandable needle electrodes: tips from preliminary experience. AJR Am J Roentgenol. 2004. 183:605–611.
6. Gadaleta C, Mattioli V, Colucci G, Cramarossa A, Lorusso V, Canniello E, et al. Radiofrequency ablation of 40 lung neoplasms: preliminary results. AJR Am J Roentgenol. 2004. 183:361–368.
7. Diederich S, Hosten N. Percutaneous ablation of pulmonary tumors: state-of-the-art 2004. Radiologe. 2004. 44:658–662.
8. Yasui K, Kanazawa S, Sano Y, Fujiwara T, Kagawa S, Mimura H, et al. Thoracic tumors treated with CT-guided radiofrequency ablation: initial experience. Radiology. 2004. 231:850–857.
9. Steinke K, Glenn D, King J, Clark W, Zhao J, Clingan P, et al. Percutaneous imaging-guided radiofrequency ablation in patients with colorectal pulmonary metastases: 1-year follow-up. Ann Surg Oncol. 2004. 11:207–212.
10. Kang S, Luo R, Liao W, Wu H, Zhang X, Meng Y. Single group study to evaluate the feasibility and complications of radiofrequency ablation and usefulness of post treatment position emission tomography in lung tumours. World J Surg Oncol. 2004. 2:30.
11. Burdio F, Guemes A, Burdio JM, Navarro A, Sousa R, Castiella T, et al. Bipolar saline-enhanced electrode for radiofrequency ablation: results of experimental study of in vivo porcine liver. Radiology. 2003. 229:447–456.
12. Lee JM, Han JK, Kim SH, Sohn KL, Lee KH, Ah SK, et al. A comparative experimental study of the in-vitro efficiency of hypertonic saline-enhanced hepatic bipolar and monopolar radiofrequency ablation. Korean J Radiol. 2003. 4:163–169.
13. Burdio F, Guemes A, Burdio JM, Castiella T, De Gregorio MA, Lozano R, et al. Hepatic lesion ablation with bipolar saline-enhanced radiofrequency in the audible spectrum. Acad Radiol. 1999. 6:680–686.
14. Lee JM, Kim SW, Li CA, Youk JH, Kim YK, Jin Z, et al. Saline-enhanced radiofrequency thermal ablation of the lung: a feasibility study in rabbits. Korean J Radiol. 2002. 3:245–253.
15. Kim TS, Lim HK, Kim H. Excessive hyperthermic necrosis of a pulmonary lobe after hypertonic saline-enhanced monopolar radiofrequency ablation. Cardiovasc Intervent Radiol. 2006. 29:160–163.
16. Sacks D, McClenny TE, Cardella JF, Lewis CA. Society of Interventional Radiology clinical practice guidelines. J Vasc Interv Radiol. 2003. 14:S199–S202.
17. Denys AL, De Baere T, Kuoch V, Dupas B, Chevallier P, Madoff DC, et al. Radio-frequency tissue ablation of the liver: in vivo and ex vivo experiments with four different systems. Eur Radiol. 2003. 13:2346–2352.
18. Burdio F, Burdio JM, Navarro A, Ros P, Guemes A, Sousa R, et al. Electric influence of NaCl concentration into the tissue in radiofrequency ablation. Radiology. 2004. 232:932.
19. Goldberg SN, Stein MC, Gazelle GS, Sheiman RG, Kruskal JB, Clouse ME. Percutaneous radiofrequency tissue ablation: optimization of pulsed-radiofrequency technique to increase coagulation necrosis. J Vasc Interv Radiol. 1999. 10:907–916.
20. Goldberg SN, Solbiati L, Hahn PF, Cosman E, Conrad JE, Fogle R, et al. Large volume tissue ablation with radiofrequency by using a clustered, internally cooled electrode technique: laboratory and clinical experience in liver metastases. Radiology. 1998. 209:371–379.
21. Solbiati L, Ierace T, Goldberg SN, Sironi S, Livraghi T, Fiocca R, et al. Percutaneous US-guided radiofrequency tissue ablation of liver metastases: treatment and follow up in 16 patients. Radiology. 1997. 202:195–203.
22. Rossi S, Garbagnati F, Lencioni R, Allgaier HP, Marchiano A, Fornari F, et al. Percutaneous radio-frequency thermal ablation of non-resectable hepatocellular carcinoma after occlusion of tumor blood supply. Radiology. 2000. 217:119–126.
23. Berber E, Flesher NL, Siperstein AE. Initial clinical evaluation of the RITA 5-centimeter radiofrequency thermal ablation catheter in the treatment of liver tumors. Cancer J Sci Am. 2000. 6:S319–S329.
24. Livraghi T, Goldberg SN, Monti F, Bizzini A, Lazzaroni S, Meloni F, et al. Saline-enhanced radio-frequency tissue ablation in the treatment of liver metastases. Radiology. 1997. 202:205–210.
25. Hoey MF, Mulier P, Shake JG. Intramural ablation using radiofrequency energy via screw-tip catheter and saline electrode. Pacing Clin Electrophysiol. 1995. 18:917.
26. Ymagami T, Iida S, Kato T, Tanaka O, Nishimura T. Combining fine-needle aspiration and core biopsy under CT fluoroscopy guidance: a better way to treat patients with lung nodules? AJR Am J Roentgenol. 2003. 180:811–815.
27. Ohno Y, Hatabu H, Takenaka D, Higashino T, Watanabe H, Ohbayashi C, et al. CT-guided transthoracic needle aspiration biopsy of small (< or = 20 mm) solitary pulmonary nodules. AJR Am J Roentgenol. 2003. 180:1665–1669.
28. Lee JM, Youk JH, Kim YK, Han YM, Chung GH, Lee SY, et al. Radio-frequency thermal ablation with hypertonic saline solution injection of the lung: ex vivo and in vivo feasibility studies. Eur Radiol. 2003. 13:2540–2547.