Abstract
Objective
The purpose of this study was to describe the myocardial enhancement patterns in patients with myocardial infarction using two-phase contrast-enhanced multidetector-row computed tomography (MDCT).
Materials and Methods
Twenty-three patients with clinically proven myocardial infarction (17 acute myocardial infarction [AMI] and 6 chronic myocardial infarction [CMI]) were examined with two-phase contrast-enhanced ECG-gated MDCT. The presence, location, and patterns of myocardial enhancement on two-phase MDCT images were compared with infarcted myocardial territories determined by using electrocardiogram, echocardiography, thallium-201 single photon emission computed tomography, catheter and MDCT coronary angiography.
Results
After clinical assessment, the presence of myocardial infarctions were found in 27 territories (19 AMI and 8 CMI) of 23 patients. Early perfusion defects were observed in 30 territories of all 23 patients. Three territories not corresponding to a myocardial infarction were detected in three patients with AMI and were associated with artifacts. Fourteen of perfusion defects were in the left anterior descending artery territory, four in the left circumflex artery territory, and nine in the right coronary artery territory. Delayed enhancement was observed in 25 territories (17 AMI and 8 CMI) of 21 patients. Delayed enhancement patterns were variable. Transmural early perfusion defects (n =12) were closely associated with transmural late enhancement (n = 5) and subendocardial residual defect with subepicardial late enhancement (n = 5).
Conclusion
Myocardial infarction showed early perfusion defects and variable delayed enhancement patterns on two-phase contrast-enhanced MDCT. Delayed enhancement technique of MDCT could provide additional information of the location and extent of infarcted myocardium, and could be useful to plan appropriate therapeutic strategies in patients with AMI.
Myocardial infarction (MI) occurs if complete coronary arterial occlusion occurs for at least 15-20 minutes. A wave-front of myocardial necrosis begins in the endocardium and it spreads to the epicardium. The acute phase of MI, in which swelling of the infarcted cells and interstitial edema take place, is followed by infiltration of inflammatory cells within the first four days and there is also penetration of blood capillaries and connective tissue from the periphery. Up until the third week, the necrotic area is surrounded and progressively invaded by granulation tissue, and this leads to a fibrous scar (1-3).
A imaging tool that can provide detailed information on myocardial perfusion and viability at the same time will help in the prognostic assessment of the patient with MI, and may also be valuable for planning appropriate therapeutic strategies. MI imaging is generally performed by using echocardiography, nuclear imaging techniques, and contrast-enhanced magnetic resonance (MR) imaging. It was known that infarcted myocardium showed a slight decrease in attenuation as compared with normal myocardium on unenhanced computed tomography (CT) and hypoenhancement or paradoxical hyperenhancement on delayed scan 10 min or longer after the administration of contrast medium. However, single-slice nonspiral CT scan has not been widely used for diagnosing MI due to its low temporal and spatial resolution (4-6). In recent years, multidetector-row computed tomography (MDCT) has been validated as a useful non-invasive diagnostic tool for patients with ischemic heart disease because it can provides information on the coronary arteries and on the detailed cardiac morphology at the same time (7-10). Even though MDCT has shown the capability to detect MI, including information on an infarct's size and age, only a few scientific reports have been published regarding the myocardial enhancement of MI with using single or two-phase contrast-enhanced MDCT (11-15). Therefore, the aim of this study was to describe the myocardial enhancement patterns in MI patients with using two-phase contrast-enhanced MDCT.
Our hospital's institutional review board approved the research protocols, and all patients gave informed written consent. Thirty-eight patients who had undergone two-phase contrast-enhanced ECG-gated MDCT for ischemic heart disease between October 2004 and June 2005 were retrospectively identified. Among these 38 patients, there were 17 patients with acute myocardial infarction (AMI: infarction occurring less than one week before the CT study) and six patients with chronic myocardial infarction (CMI: infarction occurring more than one month before the CT study). The patient population was characterized as follows: 20 males and three females (mean age 63±11 years, range 34-82 years). A complete patient history, electrocardiography, echocardiography, and recent laboratory tests were available for all the patients. Among these 23 patients, six patients (5 AMI and 1 CMI) underwent 201Tl single photon emission computed tomography (SPECT). Catheter angiography was performed on 19 patients (17 AMI and 2 CMI) for diagnosis and percutaneous coronary intervention.
Acute myocardial infarction was diagnosed if the patient had 1) clinical symptoms, 2) electrocardiographic changes indicative of MI, and 3) characteristic elevation of the serum enzyme levels. CMI was diagnosed if the patient had 1) a history of previous MI, 2) diagnostic Q waves on the electrocardiogram or ECG registration of the acute event, and 3) normal enzyme levels during the observation period. The patients with ST segment elevation MI (n = 10) were treated with thrombolytics before their CT scans. Contrast-enhanced MDCT was performed before the cardiac catheterization. The average time between two-phase contrast-enhanced MDCT and catheter angiography was 4±3 days.
Each patient's heart rate (HR) was measured prior to the examination. Patients with a pre-scan HR≥65 beats per minute (bpm) were given 25-50 mg of atenolol (Tenormin, Hyundai) per os 1 h before the scan. Two-phase contrast-enhanced ECG-gated MDCT was performed using a Sensation 16 scanner (Siemens, Forchheim, Germany). The acquisition protocol included both early-phase acquisition for analysis of the coronary arteries and myocardial perfusion of the left ventricle, and late-phase acquisition that was performed 7 minutes after this first-pass acquisition for analysis of the delayed enhancement patterns. The following parameters were employed: 16×0.75 mm collimation, 0.37s rotation time, table feed 6.8 mm/rotation, 120 kV, and 620 mAs, resulting in a total scan time of about 20s to cover the entire heart, and the scan was acquired a single breath hold with using retrospective ECG gating. A contrast injection protocol with injection of 100 ml of non-ionic contrast material (Ultravist 370®, Schering, Berlin, Germany) at a flow rate of 3.5 ml/s followed by a saline chaser bolus of 50 ml at the same flow rate was used. CT scanning started with real-time bolus tracking (CARE bolus, Siemens, Forchheim, Germany) using a region of interest in the ascending aorta for monitoring a threshold of +100 HU above the baseline attenuation. A late-phase acquisition was obtained using the same protocol, except for reducing the mAs to 310. MDCT evaluation was done on a workstation (Syngo, Wizard; Simens Medical solutions). MDCT coronary angiography was used for detecting coronary stenosis in four CMI patients who did not have catheter angiography performed. A series of maximum intensity projection images with a 4-mm thick slab were generated in the short-axis, horizontal long-axis and vertical long-axis plane of the heart from early-phase axial images. The presence of significant coronary stenosis was visually defined as more than 50% luminal narrowing. The contrast enhancement patterns were assessed with the multiplanar vertical long-axis and shot-axis views by using 5-mm-thick slab sections of the heart from the early and late-phase axial images taken at mid-diastole.
The two-phase multiplanar reformation (MPR) images of each patient were analyzed by two experienced radiologists in consensus and unaware of any clinical information and the results of both the catheter and MDCT coronary angiography. Assessment of myocardial enhancement patterns was based on those used in a previous study with using two-phase contrast-enhanced MDCT (14) and as follows: the presence of a hypoenhanced area on the early-phase images as an early perfusion defect, the presence of persistent hypoenhanced area in the subendocardium surrounded by partially enhanced myocardium on the latephase images as a residual perfusion defect, and the presence of a hyperenhanced area on the late-phase images as late enhancement. Regions of interest of 2-5 mm2 in size were placed over the center of the area of each finding and the surrounding normal myocardium. For all the perfusion defects and delayed enhancement detected on the two-phase MPR images, the location of each detected MI within the left ventricular myocardium was defined with using a 17-segment model in the cardiac short axis, as recently suggested by the American Heart Association (AHA) (16). The depth of myocardial involvement was classified into three regions as subendocardial: involvement of less than 1/2 of the left inner ventricle wall thickness, subepicardial: involvement of less than 1/2 of the outer wall thickness, and transmural: involvement of more than 50% of the wall thickness. The readers visually adjusted the window parameters on an individual basis. The typical window width and level settings ranged between 150-350 and 60-200 HU, respectively.
The presence, location, and patterns of myocardial enhancement on two-phase MDCT images were compared with infarcted myocardial territories determined by using electrocardiogram, echocardiography, 201Tl SPECT, catheter and MDCT coronary angiography. The continuous variables are presented as mean±standard deviation. Comparison of the CT attenuation numbers of the normal and abnormally enhanced myocardial segments on the two-phase MDCT images were done with using paired Student's t-tests. A p value of 0.05 or less was considered to indicate a statistically significant difference. For statistical analysis, a commercially available Windows-based software product was used (SPSS 12.0.1, 2003).
Fifteen of 23 (65%) patients received a beta-blocker regimen before their scan (5 patients had an HR < 65 bpm and 3 patients had a blood pressure < 100/65 mmHg). The mean HR was 67.7±6.6 bpm and it ranged from 57 to 78 bpm. Beta-blocker (25-50 mg of atenolol) decreased the HR 12 bpm in our study. Of the 23 patients who were analyzed, 21 had significant coronary stenoses on catheter or MDCT coronary angiography.
By clinical assessment, a total of 27 infarct territories (19 AMI and 8 CMI) were identified in 23 patients. In the 19 patients who underwent catheter angiography, 21 infarcted myocardial territories were clinically identified and 17 infarct-related arteries were detected. Ten of these infarct-related arteries were in the left anterior descending coronary artery (LAD), two in the left circumflex coronary artery (LCX) and five in the right coronary artery (RCA). Two patients with AMI had double anterior and inferior MIs in which the infarct-related arteries were assigned to the LAD because each RCA was totally occluded along with the presence of collaterals from the LAD. Two infarcted territories (1 LAD and 1 LCX) that were not associated with significant coronary stenoses were noted in two patients with reperfused AMI. All 23 patients showed 30 hypoenhanced territories of the left ventricle myocardium on the early-phase MDCT scan. Twenty-seven of 30 territories corresponded to clinically assessed MI territories (Table 1). In three (1 LAD and 2 LCX) of 30 territories, the areas of decreased myocardial attenuation were not corresponded to infarcted myocardial territories and this discrepancy was caused by artifacts that were seen as transverse dark bands crossing the left ventricle on the short-axis images. Early perfusion defects were noted in the 19 territories of 17 patients with AMI (Figs. 2A, 3A, 4A) and 8 territories of six patients with CMI (Fig. 1A). The attenuation of the early perfusion defects (40.3 HU± 13.2) was significantly lower than that of the noninfarcted areas (120.3 HU±12.8, p < 0.001). Among the 27 territories of the early perfusion defects, 15 were located in the subendocardium (Figs. 1A, 4A) and 12 were located in the transmural myocardium (Figs. 2A, 3A) (Table 2). A total of 25 of the 27 (93%) territories with early perfusion defects were consistent with the territories of significant coronary stenoses, as observed on the catheter or MDCT coronary angiography.
Delayed enhancement was observed in 16 of 17 (94 %) AMI patients and in all six (100 %) CMI patients. A total of 25 territories (17 AMI and 8 CMI) with delayed enhancement were identified on the MDCT scans, which corresponded to clinically assessed infarct territories. Late enhancement (n = 19, 98.8 HU±10.1) had higher attenuation compared with the residual perfusion defects (n = 12, 40.5 HU±11.7, p < 0.001) and the remote noninfarcted areas (80.5 HU±5.7; p < 0.001) that were observed on the late-phase images. Two territories (LAD and RCA) with subendocardial perfusion defects in one AMI patient did not show delayed enhancement, but they showed fixed perfusion defects on 201Tl SPECT. The delayed enhancement patterns were variable (Table 3): 1) five territories of subendocardial late enhancement (Fig. 1B), 2) eight territories of transmural late enhancement (Figs. 2B, 4B), 3) six territories of subendocardial residual perfusion defect, 4) six territories of subendocardial residual perfusion defect with subepicardial late enhancement (Fig. 3B). For the association of the delayed enhancement patterns with the depth of myocardial involvement of the early perfusion defect, the subendocardial perfusion defects (n = 15)showed various delayed enhancement patterns, but the transmural perfusion defects (n = 12) mainly showed transmural late enhancement (n = 5) and subendocardial residual defect with subepicardial late enhancement (n = 5) (Table 4).
Single-slice nonspiral CT has not been widely used for evaluating MI because of the low temporal and spatial resolution even though this modality was able to detect and characterize MI in both animal models and human subjects (4-6). Electron beam CT could assess regional myocardial perfusion, non-invasively detect coronary artery disease, and assess the risk of future coronary events by quantifying calcification of the coronary arteries. But this modality failed to gain popularity because of its high cost, limited availability, high radiation exposure and the limited extra information compared to the other established imaging modalities (17-19). In recent years,MDCT has been proposed as a useful non-invasive imaging method for evaluating both coronary artery stenosis and the cardiac morphology at the same time. In addition, MDCT can provide information about myocardial perfusion because MDCT coronary angiographic scanning is performed during maximal enhancement of the coronary artery. Koyama et al. (14, 15) reported that the early perfusion defects seen on two-phase contrast-enhanced MDCT might reflect a decrease in the myocardial blood flow, and so this might correspond to myocardial necrosis with extensive capillary disorder, or to myocardial necrosis in those patients with reperfused AMI. On the cardiac MR image, early perfusion defect indicates myocardial microcirculatory occlusion and edema in an area of MI (20-24).
In our study, all 23 patients showed 30 hypoenhanced territories of the left ventricle myocardium on the early-phase MDCT scan. In 3 of the 30 territories, artifacts were responsible for the false-positive territories. These artifacts were seen as transverse dark bands crossing the left ventricle on short-axis images and they might be associated with arrythmia and higher HR. Two territories with perfusion defects were noted in two patients with reperfused AMI who did not have a significant coronary stenosis; this possibly corresponded to resolution of thrombus in the coronary artery.
In one study using an animal model to evaluate the usefulness of MDCT for reperfused MI, the areas showing hyperenhancement on the delayed CT scans were significantly correlated with the histopathologic results (11). On MR studies, delayed hyperenhancement obtained within 5 to 10 minutes after injection of contrast medium mainly reflects the volume of the interstitial space and this correlates with the localization and extent of the myocardial infarction. Persistent hypoenhancement is caused by microvascular obstruction and this is associated with non-viability (2, 23, 25-28). Koyama et al. (14, 15) classified patients with reperfused AMI into 3 groups according to the myocardial enhancement patterns with using two-phase contrast-enhanced MDCT. In group 3 that had early perfusion defect, residual defect and late enhancement, the wall thickness of the chronic infarcted stage was significantly thinner than that of the acute infarcted stage, and the deterioration of left ventricular function was more severe than that observed in group 2, which did not have residual defect. That study's results demonstrated that the variability in the enhancement patterns indicated variability in the extent of microvascular damage, and so assessment of myocardial enhancement patterns could serve as a predictor of the changes in wall motion and thickness, the left ventricular function, and the myocardial viability in patients with reperfused AMI. In our study, transmural early perfusion defects were closely associated with transmural late enhancement and subendocardial residual defect with subepicardial late enhancement. Delayed enhancement was not present in 2 of the 19 territories of early perfusion defects in the AMI patients. These 2 territories of subendocardial perfusion defects showed fixed perfusion defects on 201Tl SPECT. The false negative results on the late-phase MDCT images were probably due to weak contrast between the infarcted myocardium and the noninfarcted myocardium. Residual perfusion defects were noted in 12 territories of 11 MI patients. Residual defects are usually observed in large areas of infarcted myocardium as smaller dark subendocardial regions surrounded by a partially hyperenhanced zone and might correspond to myocardial necrosis with extensive capillary damage. Among the 12 territories of the subendocardial residual defects, 6 territories were accompanied with subepicardial late enhancement but the other 6 territories were not. The difference between these enhancement patterns was possibly due to the poor tissue contrast resolution that was insufficient to differentiate the subtle differences in Hounsfield units and an increase in the noise resulted from decreasing the mAs to 310. The time after injecting the contrast medium may be important for the detecting delayed enhancement. We adopted a standard delay of 7 minutes after injection, based on previous studies (14, 15). In an animal model, even though the contrast between the infarcted myocardium and the normal myocardium was equally prominent at both 5 min and 10 min on the delayed-phase MDCT images (11), a different time interval between the injection of contrast medium and MDCT acquisition may cause different outcomes, and further studies are needed to determine the optimal delay time. Paul et al. (29) lowered the tube voltage to 80 kV for delayed acquisition and they obtained better contrast enhancement on the delayed contrast-enhanced MDCT and decreased radiation exposure to the patients. Also, the noise was reduced by using 8-mm-thick MPRs, and making consensual assessment of myocardial enhancement was possible in all cases.
The delayed enhancement technique of MDCT technique requires a second scan that increases the radiation dose to the patient and prolongs the examination times. This is why two-phase MDCT has been not commonly used for assessing MI. In our study, the effective radiation dose for both studies was around 20 mSv even though we lowered the mAs to 310 for the delayed acquisition. When one considers the important information obtained from the delayed enhancement technique, particularly if the functional imaging techniques are not available, the radiation dose could be acceptable for AMI patients.
This study has some important limitations. The standard reference modalities for the assessment of myocardial perfusion and delayed enhancement were in short. Only six patients underwent 201Tl SPECT. Comparing the two-phase contrast-enhanced MDCT with cardiac MR imaging or nuclear imaging techniques would be of great interest. We had lowered the mAs to 310 for the late-phase acquisition to reduce the radiation dose, which caused an increase in noise. In our study population, with 15 of 23 (65%) patients receiving a beta-blocker regimen before the scan, the mean HR was 67.7±6.6 bpm and it ranged from 57 to 78 bpm. A higher HR (> 70 bpm) might cause cardiac motion artifacts that hamper the evaluation of abnormal myocardial enhancement. Interobserver variability was not assessed because the weak contrast resolution, the increase in noise, and the artifacts may have caused difficulty in assessing the myocardial enhancement patterns. Finally, the number of patients investigated in this study was rather small.
In conclusion, myocardial infarction showed early perfusion defect, late enhancement, residual perfusion defect, or subendocardial residual defect with subepicardial late enhancement on two-phase contrast-enhanced MDCT. The delayed enhancement imaging of MDCT could provide additional information on the location and residual perfusion defect of the infarcted myocardium, and it could be useful when planning appropriate therapeutic strategies for patients with AMI.
Figures and Tables
Fig. 1
Images obtained in a 57-year-old man with chronic myocardial infarction.
A. The short-axis multiplanar reformation image obtained with early-phase multidetector CT shows a subendocardial hypoenhancement involving the mid-inferolateral myocardium with mild wall thinning (arrow), which corresponds to chronic myocardial infarction of the left circumflex coronary artery territory.
B. The short-axis multiplanar reformation image obtained with late-phase multidetector CT shows subendocardial late enhancement in the same area (arrow).
C. The volume rendered image shows occlusion of the left circumflex artery (large arrow) and significant stenosis of the obtuse marginal artery (small arrow). A T-graft with the radial artery (open arrow) from the left internal mammary artery to the obtuse marginal artery and the distal anastomosis are open.
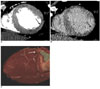
Fig. 2
Images obtained in a 73-year-old man with reperfused acute myocardial infarction.
A. The short-axis multiplanar reformation image obtained with early-phase multidetector CT shows an early perfusion defect involving the whole thickness of the mid-anterolateral myocardium (arrow), which corresponds to significant stenosis of the left circumflex coronary artery.
B. The short-axis multiplanar reformation image obtained with late-phase multidetector CT shows transmural late enhancement in the same area (arrow). Note the total absence of residual perfusion defect within the hyperenhanced area.
C. Right oblique caudal projection of the left coronary angiogram shows total occlusion with bridge collateral flow at the proximal segment of the left circumflex coronary artery (arrow).
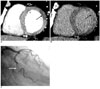
Fig. 3
Images obtained in a 72-year-old man with reperfused acute myocardial infarction.
A. The short-axis multiplanar reformation image obtained with early phase multidetector CT shows a transmural perfusion defect involving the mid-inferior myocardium (arrow), and this corresponds to significant stenosis of the right coronary artery.
B. The short-axis multiplanar reformation image obtained with late phase multidetector CT shows subendocardial residual perfusion defect (arrow) with subepicardial late enhancement (arrowhead) in the same area.
C. The left oblique projection of the right coronary angiogram shows multiple stenoses in the proximal and middle (arrows) right coronary artery and total occlusion at the distal segment (arrowhead) of the right coronary artery.
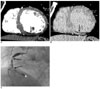
Fig. 4
The images obtained in a 63-year-old woman with reperfused acute myocardial infarction.
A. The short-axis multiplanar reformation image obtained with early phase multidetector CT shows a subendocardial perfusion defect involving the mid-anterior and anterolateral myocardium (arrow), and this corresponds to significant stenosis of the left circumflex coronary artery.
B. The short-axis multiplanar reformation image obtained with late phase multidetector CT shows transmural late enhancement in the same area (arrow).
C. The right anterior oblique caudal projection of the left coronary angiogram shows minimal lesion at the middle segment of the left circumflex coronary artery (arrow).
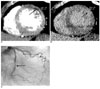
Table 1
Distribution Territories of Myocardial Infarctions and Early Perfusion Defects
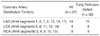
Note.-Data are the number of early perfusion defect territories.
MI = myocardial infarction, LAD = left anterior descending coronary artery, LCX = left circumflex coronary artery, RCA = right coronary artery. The 3 (1 LAD and 2 LCX) perfusion defect territories that did not correspond to a MI were caused by artifacts, AHA = American Heart Association
References
1. Kloner RA, Jennings RB. Consequences of brief ischemia: stunning, preconditioning, and their clinical implications: part 1. Circulation. 2001. 104:2981–2989.
2. Sandstede JJ. Assessment of myocardial viability by MR imaging. Eur Radiol. 2003. 13:52–61.
3. Richard V, Murry CE, Reimer KA. Healing of myocardial infarcts in dogs: effects of late reperfusion. Circulation. 1995. 92:1891–1901.
4. Lipton MJ, Higgins CB. Evaluation of ischemic heart disease by computerized transmission tomography. Radiol Clin North Am. 1980. 18:557–576.
5. Godwin JD, Moore AV, Ideker RE, Califf RM. Prospective demonstration of myocardial infarction by CT. AJR Am J Roentgenol. 1984. 143:985–986.
6. Kramer PH, Goldstein JA, Herkens RJ, Lipton MJ, Brundage BH. Imaging of acute myocardial infarction in man with contrast-enhanced computed transmission tomography. Am Heart J. 1984. 108:1514–1523.
7. Nieman K, Cademartiri F, Lemos PA, Raaijmarkers R, Pattynama PM, de Feyter PJ. Reliable noninvasive coronary angiography with fast submillimeter multislice spiral computed tomography. Circulation. 2002. 106:2051–2054.
8. Ropers D, Baum U, Pohle K, Anders K, Ulzheimer S, Ohnesorge B, et al. Detection of coronary artery stenoses with thin-slice multi-detector row spiral computed tomography and multiplanar reconstruction. Circulation. 2003. 107:664–666.
9. Nieman K, Oudkerk M, Rensing BJ, van Ooijen P, Munne A, van Geuns RJ, et al. Coronary angiography with multi-slice computed tomography. Lancet. 2001. 357:599–603.
10. Juergens KU, Grude M, Fallenberg EM, Opitz C, Wichter T, Heindel W, et al. Using ECG-gated multidetector CT to evaluate global left ventricular myocardial function in patients with coronary artery disease. AJR Am J Roentgenol. 2002. 179:1545–1550.
11. Park JM, Choe YH, Chang S, Sung YM, Kang SS, Kim MJ, et al. Usefulness of multidetector-row CT in the evaluation of reperfused myocardial infarction in a rabbit model. Korean J Radiol. 2004. 5:19–24.
12. Nikolaou K, Knez A, Sagmeister S, Wintersperger BJ, Boekstegers P, Steinbeck G, et al. Assessment of myocardial infarctions using multidetector-row computed tomography. J Comput Assist Tomogr. 2004. 28:286–292.
13. Nikolaou K, Sanz J, Poon M, Wintersperger BJ, Ohnesorge B, Rius T, et al. Assessment of myocardial perfusion and viability from routine contrast-enhanced 16-detector-row computed tomography of the heart: preliminary results. Eur Radiol. 2005. 15:864–871.
14. Koyama Y, Mochizuki T, Higaki J. Computed tomography assessment of myocardial perfusion, viability, and function. J Magn Reson Imaging. 2004. 19:800–815.
15. Koyama Y, Matsuoka H, Mochizuki T, Higashino H, Kawakami H, Nakata S, et al. Assessment of reperfused acute myocardial infarction with two-phase contrast-enhanced helical CT: prediction of left ventricular function and wall thickness. Radiology. 2005. 235:804–811.
16. Cerqueira MD, Weissman NJ, Dilsizian V, Jacobs AK, Kaul S, Laskey WK, et al. Standardized myocardial segmentation and nomenclature for tomographic imaging of the heart: a statement for healthcare professionals from the Cardiac Imaging Committee of the Council on Clinical Cardiology of the American Heart Association. Circulation. 2002. 105:539–542.
17. Gould RG. Perfusion quantitation by ultrafast computed tomography. Invest Radiol. 1992. 27:Suppl 2. S18–S21.
18. Budoff MJ, Georgiou D, Brody A, Agatston AS, Kennedy J, Wolfkiel C, et al. Ultrafast computed tomography as a diagnostic modality in the detection of coronary artery disease: a multicenter study. Circulation. 1996. 93:898–904.
19. Knollmann FD, Muschick P, Krause W, Hausmann H, Hetzer R, Felix R. Detection of myocardial ischemia by electron beam CT. Acta Radiol. 2001. 42:386–392.
20. Kim RJ, Chen EL, Lima JA, Judd RM. Myocardial Gd-DTPA kinetics determine MRI contrast enhancement and reflect the extent and severity of myocardial injury after acute reperfused infarction. Circulation. 1996. 94:3318–3326.
21. Fieno DS, Kim RJ, Chen EL, Lomasney JW, Klocke FJ, Judd RM. Contrast-enhanced magnetic resonance imaging of myocardium at risk: distinction between reversible and irreversible injury throughout infarct healing. J Am Coll Cardiol. 2000. 36:1985–1991.
22. Rogers WJ Jr, Kramer CM, Geskin G, Hu YL, Theobald TM, Vido DA, et al. Early contrast-enhanced MRI predicts late functional recovery after reperfused myocardial infarction. Circulation. 1999. 99:744–750.
23. Poon M, Fuster V, Fayad Z. Cardiac magnetic resonance imaging: a "one-stop-shop" evaluation of myocardial dysfunction. Curr Opin Cardiol. 2002. 17:663–670.
24. Sandstede JJ, Lipke C, Beer M, Harre K, Pabst T, Kenn W, et al. Analysis of first-pass and delayed contrast-enhancement patterns of dysfunctional myocardium on MR imaging: use in the prediction of myocardial viability. AJR Am J Roentgenol. 2000. 174:1737–1740.
25. Gould KL, Lipscomb K. Effects of coronary stenoses on coronary flow reserve and resistance. Am J Cardiol. 1974. 34:48–55.
26. Kurata A, Mochizuki T, Koyama Y, Haraikawa T, Suzuki J, Shigematsu Y, et al. Myocardial perfusion imaging using adenosine triphosphate stress multi-slice spiral computed tomography: alternative to stress myocardial perfusion scintigraphy. Circ J. 2005. 69:550–557.
27. Kim RJ, Wu E, Rafael A, Chen EL, Parker MA, Simonetti O, et al. The use of contrast-enhanced magnetic resonance imaging to identify reversible myocardial dysfunction. N Engl J Med. 2000. 343:1445–1453.
28. Shan K, Constantine G, Sivananthan M, Flamm SD. Role of cardiac magnetic resonance imaging in the assessment of myocardial viability. Circulation. 2004. 109:1328–1334.
29. Paul JF, Wartski M, Caussin C, Sigal-Cinqualbre A, Lancelin B, Angel C, et al. Late defect on delayed contrast-enhanced multi-detector row CT scans in the prediction of SPECT infarct size after reperfused acute myocardial infarction: initial experience. Radiology. 2005. 236:485–489.