Abstract
Objective
The aim of our study was to compare the accuracy of CT and 18F-FDG PET for detecting peritoneal metastasis in patients with gastric carcinoma.
Materials and Methods
One-hundred-twelve patients who underwent a histologic confirmative exam or treatment (laparotomy, n = 107; diagnostic laparoscopy, n = 4; peritoneal washing cytology, n = 1) were retrospectively enrolled. All the patients underwent CT and 18F-FDG PET scanning for their preoperative evaluation. The sensitivities, specificities and accuracies of CT and 18F-FDG PET imaging for the detection of peritoneal metastasis were calculated and then compared using Fisher's exact probability test (p < 0.05), on the basis of the original preoperative reports. In addition, two board-certified radiologists and two board-certified nuclear medicine physicians independently reviewed the CT and PET scans, respectively. A receiver-operating characteristic curve analysis was performed to compare the diagnostic performance of CT and 18F-FDG PET imaging for detecting peritoneal metastasis.
Results
Based on the original preoperative reports, CT and 18F-FDG PET showed sensitivities of 76.5% and 35.3% (p = 0.037), specificities of 91.6% and 98.9% (p = 0.035), respectively, and equal accuracies of 89.3% (p = 1.0). The receptor operating characteristics curve analysis showed a significantly higher diagnostic performance for CT (Az = 0.878) than for PET (Az = 0.686) (p = 0.004). The interobserver agreement for detecting peritoneal metastasis was good (κ value = 0.684) for CT and moderate (κ value = 0.460) for PET.
Obtaining preoperative knowledge on peritoneal metastasis in gastric adenocarcinoma patients is important for planning the operative procedure. This allows the surgeon to decide whether the surgery is likely to be potentially curative or palliative in nature, and it helps avoid any unnecessary laparotomy procedures. However, detecting peritoneal metastasis is difficult because the imaging modalities can vary in their ability to detect tumor deposits, and laparoscopic techniques are limited by sampling error (1). CT is known to be the first choice as a preoperative modality for detecting peritoneal metastasis. However, it has been reported that CT has a limited capacity for demonstrating peritoneal metastases (2-4) and its sensitivity can vary: this depends on such factors as the size, site and morphology of tumor deposits, the presence of ascites, the paucity of intra-abdominal fat, the adequacy of bowel opacification and the concomitant use of peritoneography (5-7).
18F-FDG PET scan has recently been recognized as a useful diagnostic technique in clinical oncology (8, 9). However, experience with using 18F-FDG PET scanning for detecting peritoneal metastasis is very limited. A few studies have reported that 18F-FDG PET demonstrates superior sensitivity to CT for evaluating peritoneal metastasis in a various abdominal malignancies (10-12). However, there is scant comparison data regarding detecting peritoneal metastasis by CT and 18F-FDG PET for the preoperative evaluation of gastric adenocarcinoma. The purpose of our study was to compare the accuracy of CT and 18F-FDG PET for detecting peritoneal metastasis in the patients suffering with gastric carcinoma.
From January 2003 to January 2004, 124 patients were referred to our hospital because of their diagnosed gastric carcinoma, and they underwent CT and 18F-FDG PET scans for the pretreatment evaluation. The radiologic, surgical, pathologic and clinical reports on these 124 patients were retrieved from an institution-wide patient database system. Our institutional review board approved all aspects of this retrospective study and did not require an informed consent from any of the patients whose records were included in our study. Twelve of the 124 patients did not undergo surgery or confirmative histologic examination. These cases were excluded from this analysis because any positive or negative outcome about peritoneal metastasis could not be confirmatively determined. The remaining 112 patients (73 men and 39 women, mean age: 55.5 years, age range: 16-80 years), who underwent histologic confirmative exam or treatment (laparotomy: n = 107, diagnostic laparoscopy: n = 4, and peritoneal washing cytology: n = 1), were enrolled in our study. Through conducting a review of the surgical or pathologic reports for confirming the presence or absence of peritoneal metastasis, 95 patients were without peritoneal metastasis based on the laparotomy findings. However, peritoneal metastasis was histologically confirmed in 17 patients (10.7%) (12 men and 5 women, mean age: 51.4 years, age range: 32-74 years). In the cases with peritoneal metastasis, the types of cell differentiation of the primary lesions were as follows: moderate differentiation: n = 2, mixed type of moderate and poor differentiation: n = 2, signet cell differentiation: n = 4, poor differentiation: n = 9.
All the patients included in the study underwent single-section spiral CT (HiSpeed CT/I, GE Medical Systems, Milwaukee, WI) or multi-detector CT scanning (four-detector row; Lightspeed Plus, GE Medical Systems, Milwaukee, WI or sixteen-detector row; Sensation 16, Siemens, Erlangen, Germany), according to an established protocol. Each patient fasted for six hours before undergoing CT, they drank 750-1000 mL of pure tap water immediately before scanning and they routinely underwent CT scans in a prone position for achieving gastric distention. Neither anticholinergic agents nor glucagon was administered. A 60% iodinated contrast material (Iopromide [Ultravist]; Schering, Berlin, diatrizoate meglumine [Hypaque] or iohexol [Omnipaque 300]; Nycomed Amersham, Princeton, NJ) was administered intravenously at a rate of 2-4 mL/sec by using an automatic power injector with a volume of 2 mL/kg up to a maximum volume of 150 mL/kg. CT scans were obtained at approximately 70 seconds (the parenchymal phase) after initiating the contrast material injection. The abdomen and pelvis, from the level of the hepatic dome to the ischial tuberosities, were scanned with 0.75-7.0 mm section collimation, a pitch of 1.0-1.5, and 3.0-7.0 mm of reconstruction thickness. The transverse images were reconstructed with a soft-tissue algorithm.
18F-FDG PET was performed in all the patients within a month of the CT study (range: 0-28 days, mean: 9.3 days). The patients fasted for at least four hours before the intravenous injection of 370-555 MBq of FDG. Scanning was initiated 60 min after administration of the tracer. The images were obtained on either a GE advance PET scanner (GE advance, GE Medical Systems, Milwaukee, WI) or a Philips Allegro PET system (Allegro, Philips-ADAC medical systems, Cleveland, OH). The intrinsic spatial resolution of these systems was approximately 5 mm (full-width at half-maximum) in the center of the field of view. Sequential emission scans were acquired from the neck to the proximal thighs. Transmission scans, with using Ge-68 for the GE advance system or Cs-137 point sources for the Allegro system, were obtained at the end of the emission scans in order to correct for non-uniform attenuation. The images were reconstructed using order subset expectation maximization (OSEM) for the GE advance system or a low action maximal likelihood algorithm (RAMLA) for the Allegro system.
The original preoperative CT and PET reports for the 112 patients were reviewed. Three experienced gastrointestinal radiologists were involved in the preoperative diagnoses using CT; the radiologists' experience ranged from three to 15 years. Two experienced nuclear medicine physicians were also involved in the preoperative diagnoses using PET; the physicians' experiences were five and 15 years, respectively. In the cases in which the interpreters identified or suspected peritoneal metastasis on the original preoperative reports, the study coordinator considered those cases to be positive for the presence of peritoneal metastasis. We compared the presence or absence of peritoneal metastasis, according to the CT and PET imaging, with the surgical and histopathologic standards of reference for each patient; we then determined the number of true-positive, true-negative, false-positive and false-negative imaging results. The sensitivity, specificity and accuracy of each imaging modality were calculated and compared using Fisher's exact probability test (p < 0.05).
We performed receiver-operating characteristic curve (ROC) analysis to compare the diagnostic performance of CT and PET imaging for detecting peritoneal metastasis. Among 17 patients (12 men and 5 women, mean age: 51.4 years, age range: 32-74 years) with histologically proven peritoneal metastasis, 16 patients were included in the positive group for the ROC analysis. One patient was excluded because the CT imaging data was not available. Furthermore, among the 95 patients who showed no peritoneal abnormalities on the surgical findings, the study coordinator selected 22 patients to be in the negative group (12 men and 10 women, mean age: 55.5 years, age range: 16-72 years) since they showed negative findings on the six month follow-up CT. The six month follow-up criteria were used to exclude the hidden peritoneal metastasis and to control the sample size for the ROC analysis. Two board-certified radiologists, who had subspecialist expertise in abdominal imaging, independently reviewed the CT images on a high-resolution computer screen; the reviewer's experiences were three and five years, respectively. The PET images were also reviewed by two board-certified nuclear medicine physicians; the reviewer's experiences were two and three years, respectively. All the reviewers were unaware of the patients' clinical or pathologic findings about peritoneal metastasis. They rated the likelihood of peritoneal metastases on a five point scale as follows: 1, definitely absent; 2, probably absent; 3, indeterminate; 4, probably present; and 5, definitely present. For the CT images, the reviewers used the same criteria for the identification of peritoneal metastasis, that is, the presence of a nodular, plaque-like or infiltrative soft-tissue lesion in the peritoneal fat or on the peritoneal surface (13, 14) (Figs. 1A, 2A, 3A). The presence of ascites, parietal peritoneal thickening or enhancement, and small-bowel wall thickening or distortion were also used as references since these findings have been described as ancillary signs of peritoneal malignancy (15, 16). On the PET images, the reviewers regarded certain focal or diffuse metabolic abnormalities in the abdomen or pelvis as signs of peritoneal metastasis (Fig. 1B). Discrete foci of increased FDG metabolism located either randomly and anteriorly within the abdomen or dependently within the pelvis, which were unrelated to solid viscera or nodal stations, were used as indicators of peritoneal metastasis (12) (Fig. 2B). The pattern of diffuse, low-grade glucose hyper-metabolism spreading uniformly throughout the abdomen and pelvis and obscuring the visceral outlines, and particularly the normal serpiginous pattern of the large and small bowel and the physiologic hepatic and splenic uptake, was also considered to be a sign of peritoneal metastasis (12, 17).
Before the ROC analysis, the interobserver agreement on the image interpretation for the CT and PET imaging was assessed with the weighted κ statistic for establishing their reliability in our study. The degrees of agreement were categorized as follows: κ values of 0.00-0.20 were considered to indicate poor agreement,κ values of 0.21-0.40 were fair agreement,κ values of 0.41-0.60 were moderate agreement, κ values of 0.61-0.80 were good agreement and κ values of 0.81-1.00 were excellent agreement (18).
To represent the performance of all the observers as a group, we plotted the receiver operating characteristic curves for the composite data assigned by each observer for the CT and PET imaging, as was demonstrated in previously published articles (19, 20). With the use of ROC evaluation software (MedCalc Software, Mariakerke Belgium), the diagnostic accuracy of each imaging technique was determined by calculating the area under the ROC curve (Az). The differences between the ROC curves were tested for their significance (p < 0.05) with using the two-tailed area test for paired data (a univariate z score test on the difference between the areas under the ROC curves with the null hypothesis being that the data sets arose from binomial ROC curves with equal areas beneath them).
The number of true-positive, true-negative, false-positive and false-negative cases, as well as the sensitivity, specificity and accuracy of CT and PET imaging for the diagnosis of peritoneal metastasis, are listed in Table 1. The sensitivities of CT and PET for detecting peritoneal metastasis were 76.5% (13 of 17 patients, 95% confidence interval: 53 and 90) and 35.3% (6 of 17 patients, 95% confidence interval: 17 and 59) (p = 0.037), respectively (Fig. 3). The specificities of CT and PET were 91.6% (87 of 95 patients, 95% confidence interval: 84 and 96) and 98.9% (94 of 95 patients, 95% confidence interval: 94 and 100) (p = 0.035), respectively (Fig. 4). The accuracies of CT and PET were equal (89.3% each) (p = 1.0).
The calculated Az values from each reviewer for CT and PET are shown in Table 2. The composite ROC curves formed on the basis of the pooled data from the two observers for the CT and PET, respectively, are shown in Figure 5. The accuracy of CT (Az = 0.878) was significantly superior compared with the accuracy of PET (Az = 0.686) for detecting peritoneal metastasis (p = 0.004). The interobserver agreement for detecting peritoneal metastasis was good (κ value = 0.684) for CT and moderate (κ value = 0.460) for PET. The Kappa value tended to be higher for the CT images.
Our present findings, on the basis of analyzing the original preoperative reports, showed that CT had a higher sensitivity (76.5% vs 35.3%), a lower specificity (91.6% vs 98.9%), and an equal accuracy (89.3%) when compared with the PET imaging. In the clinical setting of peritoneal carcinomatosis, the higher sensitivity of CT will help physicians avoid unnecessary surgery in the patients with peritoneal metastasis. The higher specificity of PET suggests that PET may be helpful in the equivocal cases on CT, as this will preclude missing the chance for performing curative surgery. However, CT imaging also appeared to be superior to PET imaging when comparing the diagnostic performance of the two modalities (91.6% vs 71.4%, respectively).
Although the role of 18F-FDG PET in the evaluation of peritoneal metastasis has not yet been established, several studies have shown promising results. Turlakow et al. have reported the superior sensitivity of PET to CT for their patients who had various malignancies, including gastric malignancy (12). Tanaka et al. have also demonstrated PET sensitivities far superior to CT for the evaluation of colorectal tumor peritoneal recurrence (11). The results for the evaluation of primary serous peritoneal carcinoma or bile duct cancer appear similarly promising (10, 21). However, our results suggests that 18F-FDG PET is not significantly better than CT for the detection of peritoneal metastasis in the patients with gastric carcinoma, and this finding stands in contrast to the previous reports (10-12, 21) on patients with various abdominal malignancies.
Open laparotomy with peritoneal biopsy is the gold standard for the diagnosis of peritoneal metastasis because the peritoneum may be assessed visually and it can also be carefully examined by hand. Although a greater number of diagnostic biopsies may be performed with open laparotomy and the ascitic fluid easily sampled, this technique is invasive (12). CT remains as the preoperative modality of choice for the diagnosis of peritoneal metastasis because it is widely available at most medical centers, less expensive than the other imaging modalities and less invasive than surgical procedures. However, so far, peritoneal metastasis is often detected and confirmed intraoperatively due to the limited capability of CT (15). Some authors have described the relatively lower sensitivity (17-54%) on the basis of their results using conventional CT scanning with a relatively thick section thickness (5, 7, 22). Metastases can vary in size and they can be as small as a few millimeters. If the section thickness exceeds the size of the lesion, then the lesions may not be optimally seen due to partial volume averaging. Therefore, with using conventional CT scanners, it was technically not possible to obtain thin sections through the relatively large volume of the abdomen and pelvis. In most studies, 10-mm thick sections were obtained either contiguously or at 10-mm intervals. As a result, there was a high false-negative rate for detecting peritoneal metastases in patients. The acquisition of very thin sections has been made possible by the recent advances in scanner technology (single or multi-detector CT). Several reports have suggested that thin sections may improve the accuracy of CT for detecting peritoneal metastasis in ovarian cancer (13, 23). The CT examinations in our study were performed with various CT scanners (single or multi-detector CT) and with using various section thicknesses; however, all the section thicknesses were 7 mm or smaller (3-7 mm). Our relatively higher sensitivity (76.5%) may be a result of the thinner section thickness rather than those used by the previous published reports (5, 7, 22).
There are several well known limitations of PET imaging that could be associated with the relatively lower sensitivity or diagnostic performance for the preoperative detection of peritoneal metastasis in the patients with gastric cancer. The first is the low spatial resolution of PET imaging. Because of the physical characteristics of the PET scanner and specifically the spatial resolution of 4-5 mm or higher, small seeded peritoneal nodules may be missed with 18F-PET imaging. Secondly, the 18F-FDG uptake by gastric cancer shows variable results according to the cell differentiation. Stahl et al. have reported that mucinous and signet ring cell carcinomas show significantly lower 18F-FDG uptake than do the other histologic types of gastric cancer (24). Yoshioka et al. have reported that poorly differentiated adenocarcinomas and signet ring cell carcinomas tend to show low 18F-FDG accumulation in the primary lesions, and this is probably because of the low concentration of cancer cells with a lot of stromal tissue, in spite of their aggressiveness (25). The same problems might be expected to occur with peritoneal metastasis, although it is well known that the differentiation of primary and metastatic gastric cancer lesions can be very different. In our study, most of the cell differentiation in the patients with peritoneal metastasis was poor or of the signet type (13/17). These larger proportions of poor or signet cell differentiation correspond with the previous studies showing that diffuse type cancers (poor, signet ring cell and mucinous differentiation) were more correlated with peritoneal metastasis, rather than the intestinal type metastasis (26-28). These cell differentiations might be related to the lower sensitivity of PET imaging. Third, there is marked interobserver variability for the physiological peritoneal 18F-FDG uptake owing to the differences in involuntary muscle activity (ex. intestine) (29). In our study, the κ values for PET imaging also showed a lower tendency than those of the CT imaging (PET: 0.460 and CT: 0.684). Fourth, PET imaging cannot detect ascitic fluid, which is the most common finding of peritoneal metastasis and this could easily be detected with CT.
Our present study contained several significant limitations. First, the study population was small. Further studies with a larger sample size are needed to statistically confirm our results. In addition, for the determination of sensitivity, specificity and accuracy with using the original preoperative reports, we regarded both the definitely identified and suspected peritoneal metastases as positive cases. This process may result in statistical error. Second, the cases that did not undergo surgery or histologic confirmative examination were excluded from our study. This exclusion may have resulted in a potentially important source of selection bias. However, this selection bias cannot be overcome in the clinical setting. Third, we performed our analysis on a per-patient basis, rather than on a per-lesion basis, because we cannot determine the site-by-site correlations of CT, PET and the surgical findings for the depiction of peritoneal implants. However, the diagnosis of peritoneal spread is a critical observation and the distribution of the peritoneal implants is of lesser importance for most cancers. Forth, this study included CT scans that were obtained by using a variety of CT techniques (various CT scanners; single or multi-detector CT scanners or various section collimation, i.e., 0.75-7 mm). However, our CT protocol used similar scanning levels, including the entire abdomen and pelvis, and all the reconstruction thicknesses were 7 mm or smaller. Finally, our study was performed to evaluate the accuracy of each CT and PET modality. A new imaging technique combining PET and CT equipment has recently been introduced for clinical use. A comparative trial with this combined PET and CT equipment for the detection of peritoneal metastasis should be undertaken.
In conclusion, for the patients with gastric adenocarcinoma, CT was more sensitive and showed a higher diagnostic performance than did PET for the detection of peritoneal metastasis, although CT had a relatively lower specificity than did PET. Adequate surveillance of peritoneal metastasis in gastric cancer patients based on PET alone is not possible. CT remains as the most important investigative modality for the preoperative evaluation of peritoneal metastases from gastric cancer.
Figures and Tables
Fig. 1
A 62-year-old man with poorly differentiated adenocarcinoma of the stomach.
A. The transverse contrast enhanced CT scan shows multifocal peritoneal implants in the omentum (arrows).
B. The PET image shows the diffuse FDG uptake that obscures the visceral outlines in the peritoneal cavity (arrows). Peritoneal metastasis was confirmed by diagnostic laparoscopy.
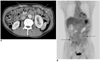
Fig. 2
A 60-year-old man with poorly differentiated adenocarcinoma of the stomach.
A. The transverse contrast enhanced CT scan shows a peritoneal implant (arrow) in the right paracolic gutter.
B. The PET image shows a discrete focus (arrow) of increased FDG metabolism in the same location. Peritoneal metastasis was confirmed by laparotomy.
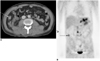
Fig. 3
A 31-year-old man with poorly differentiated adenocarcinoma of the stomach.
A. The transverse contrast enhanced CT scan shows multifocal peritoneal implants in the omentum (arrows).
B. The transverse CT scan also shows ascitic fluid in the right paracolic gutter (arrows). The CT reviewers interpreted these findings as being highly suggestive of peritoneal metastasis.
C. The PET image does not show significantly increased FDG uptake in the peritoneal cavity, except for the normal physiologic colon uptake in the ascending colon. Peritoneal metastasis was confirmed by laparotomy.
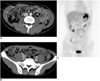
Fig. 4
A 72-year-old man with moderately differentiated adenocarcinoma of the stomach.
A. Multiple tiny soft tissue infiltrations are suspected in the right paracolic gutter on the CT image (arrows). The CT reviewers interpreted this finding as being peritoneal metastasis.
B. The PET image does not show significantly increased FDG uptake in the peritoneal cavity. The absence of peritoneal metastasis was identified by laparotomy.
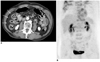
Fig. 5
Plot showing the receiver operating characteristic curves for detecting peritoneal metastasis with using CT (solid line) and PET (dotted line).
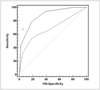
Acknowledgement
This work was supported by Yonsei University Research Fund of 2004. The authors thank Seong Ho Park for his help on the statistical analysis.
References
1. Champault G, Barrat C. Laparoscopy in the staging of cancer of the stomach. J Chir (Paris). 1999. 136:150–155.
2. Davies J, Chalmers AG, Sue-Ling HM, May J, Miller GV, Martin IG, et al. Spiral computed tomography and operative staging of gastric carcinoma: a comparison with histopathological staging. Gut. 1997. 41:314–319.
3. Dux M, Richter GM, Hansmann J, Kuntz C, Kauffmann GW. Helical hydro-CT for diagnosis and staging of gastric carcinoma. J Comput Assist Tomogr. 1999. 23:913–922.
4. Sendler A, Dittler HJ, Feussner H, Nekarda H, Bollschweiler E, Fink U, et al. Preoperative staging of gastric cancer as precondition for multimodal treatment. World J Surg. 1995. 19:501–508.
5. Low RN, Barone RM, Lacey C, Sigeti JS, Alzate GD, Sebrechts CP. Peritoneal tumor: MR imaging with dilute oral barium and intravenous gadolinium-containing contrast agents compared with unenhanced MR imaging and CT. Radiology. 1997. 204:513–520.
6. Gryspeerdt S, Clabout L, Van Hoe L, Berteloot P, Vergote IB. Intraperitoneal contrast material combined with CT for detection of peritoneal metastases of ovarian cancer. Eur J Gynaecol Oncol. 1998. 19:434–437.
7. Boudiaf M, Bedda S, Soyer P, Panis Y, Zidi S, Kardache M, et al. Preoperative evaluation of gastric adenocarcinomas: comparison of CT results with surgical and patholgic results. Ann Chir. 1999. 53:115–122.
8. Rohren EM, Turkington TG, Coleman RE. Clinical applications of PET in oncology. Radiology. 2004. 231:305–332.
9. Park DH, Kim KH, Park SY, Lee BH, Choi CW, Chin SY. Diagnosis of recurrent uterine cervical cancer: computed tomography versus positron emission tomography. Korean J Radiol. 2000. 1:51–55.
10. Kluge R, Schmidt F, Caca K, Barthel H, Hesse S, Georgi P, et al. Positron emission tomography with [(18)F]fluoro-2-deoxy-D-glucose for diagnosis and staging of bile duct cancer. Hepatology. 2001. 33:1029–1035.
11. Tanaka T, Kawai Y, Kanai M, Taki Y, Nakamoto Y, Takabayashi A. Usefulness of FDG-positron emission tomography in diagnosing peritoneal recurrence of colorectal cancer. Am J Surg. 2002. 184:433–436.
12. Turlakow A, Yeung HW, Salmon AS, Macapinlac HA, Larson SM. Peritoneal carcinomatosis: role of (18)F-FDG PET. J Nucl Med. 2003. 44:1407–1412.
13. Buy JN, Moss AA, Ghossain MA, Sciot C, Malbec L, Vadrot D, et al. Peritoneal implants from ovarian tumors: CT findings. Radiology. 1988. 169:691–694.
14. Halvorsen RA Jr, Panushka C, Oakley GJ, Letourneau JG, Adcock LL. Intraperitoneal contrast material improves the CT detection of peritoneal metastases. AJR Am J Roentgenol. 1991. 157:37–40.
15. Walkey MM, Friedman AC, Sohotra P, Radecki PD. CT manifestations of peritoneal carcinomatosis. AJR Am J Roentgenol. 1988. 150:1035–1041.
16. Coakley FV, Choi PH, Gougoutas CA, Pothuri B, Venkatraman E, Chi D, et al. Peritoneal metastases: detection with spiral CT in patients with ovarian cancer. Radiology. 2002. 223:495–499.
17. Lin EC, Lear J, Quaife RA. Metastatic peritoneal seeding patterns demonstrated by FDG positron emission tomographic imaging. Clin Nucl Med. 2001. 26:249–250.
18. Fleiss J. Statistical methods for rates and proportions. 1981. 2nd ed. New York: Wiley;212–236.
19. Tang Y, Yamashita Y, Arakawa A, Namimoto T, Mitsuzaki K, Abe Y, et al. Detection of hepatocellular carcinoma arising in cirrhotic livers: comparison of gadolinium- and ferumoxides-enhanced MR imaging. AJR Am J Roentgenol. 1999. 172:1547–1554.
20. Blakeborough A, Ward J, Wilson D, Griffiths M, Kajiya Y, Guthrie JA, et al. Hepatic lesion detection at MR imaging: a comparative study with four sequences. Radiology. 1997. 203:759–765.
21. Wang PH, Liu RS, Li YF, Ng HT, Yuan CC. Whole-body PET with (fluorine-18)-2-deoxyglucose for detecting recurrent primary serous peritoneal carcinoma: An initial report. Gynecol Oncol. 2000. 77:44–47.
22. Sussman SK, Halvorsen RA Jr, Illescas FF, Cohan RH, Saeed M, Silverman PM, et al. Gastric adenocarcinoma: CT versus surgical staging. Radiology. 1988. 167:335–340.
23. Prayer L, Kainz C, Kramer J, Stiglbauer R, Schurawitzki H, Baldt M, et al. CT and MR accuracy in the detection of tumor recurrence in patients treated for ovarian cancer. J Comput Assist Tomogr. 1993. 17:626–632.
24. Stahl A, Ott K, Weber WA, Becker K, Link T, Siewert JR, et al. FDG PET imaging of locally advanced gastric carcinomas: correlation with endoscopic and histopathological findings. Eur J Nucl Med Mol Imaging. 2003. 30:288–295.
25. Yoshioka T, Yamaguchi K, Kubota K, Saginoya T, Yamazaki T, Ido T, et al. Evaluation of 18F-FDG PET in patients with a, metastatic, or recurrent gastric cancer. J Nucl Med. 2003. 44:690–699.
26. Ming SC. Gastric carcinoma. A pathobiological classification. Cancer. 1977. 39:2475–2285.
27. Noda S, Soejima K, Inokuchi K. Clinicopathological analysis of the intestinal type and diffuse type of gastric carcinoma. Jpn J Surg. 1980. 10:277–283.
28. Sugano H, Nakamura K, Kato Y. Pathological studies of human gastric cancer. Acta Pathol Jpn. 1982. 32:Suppl 2. 329–347.
29. De Potter T, Flamen P, Van Cutsem E, Penninckx F, Filez L, Bormans G, et al. Whole-body PET with FDG for the diagnosis of recurrent gastric cancer. Eur J Nucl Med Mol Imaging. 2002. 29:525–529.