Abstract
Objective
We wanted to assess the feasibility and diagnostic accuracy of whole heart coronary magnetic resonance angiography (MRA) with using 3D balanced turbo-field-echo (b-TFE) with SENSE and the half-Fourier acquisition technique for identifying stenoses of the coronary artery.
Materials and Methods
Twenty-one patients who underwent both whole heart coronary MRA examinations and conventional catheter coronary angiography examinations were enrolled in the study. The whole heart coronary MRA images were acquired using a navigator gated 3D b-TFE sequence with SENSE and the half-Fourier acquisition technique to reduce the acquisition time. The imaging slab covered the whole heart (80 contiguous slices with a reconstructed slice thickness of 1.5 mm) along the transverse axis. The quality of the images was evaluated by using a 5-point scale (0 -uninterpretable, 1 - poor, 2 - fair, 3 - good, 4 - excellent). Ten coronary segments of the heart were evaluated in each case; the left main coronary artery (LM), and the proximal, middle and distal segments of the left anterior descending (LAD), the left circumflex (LCX) and the right coronary artery (RCA). The diagnostic accuracy of whole heart coronary MRA for detecting significant coronary artery stenosis was determined on the segment-by-segment basis, and it was compared with the results obtained by conventional catheter angiography, which is the gold standard.
Results
The mean image quality was 3.7 in the LM, 3.2 in the LAD, 2.5 in the LCX, and 3.3 in the RCA, respectively (the overall image quality was 3.0±0.1). 168 (84%) of the 201 segments had an acceptable image quality (≥grade 2). The sensitivity, specificity, accuracy, negative predictive value and positive predictive value of the whole heart coronary MRA images for detecting significant stenosis were 81.3%, 92.1%, 91.1%, 97.9%, and 52.0%, respectively. The mean coronary MRA acquisition time was 9 min 22 sec (±125 sec).
Three-dimensional (3D) coronary magnetic resonance angiography (MRA) with using the navigator-echo technique has been reported to be a sufficiently accurate method for identifying significant stenoses of the major epicardial coronary artery (1-4). Various coronary MRA methods and targeted double-oblique volume acquisition techniques have been developed with using the 3D balanced gradient echo sequence, and they are widely used for the diagnosis of this disease (5-10). However, these techniques fail visualize the side branches that are not included in the 3D imaging slab, and they are time-consuming because every targeted volume acquisition technique requires repeated scans to cover each coronary artery.
In this study, we used the free-breathing 3D balanced turbo-field-echo (b-TFE) sequence, in which the 3D slab covered the whole heart (the slab thickness was 120 mm); the SENSE (sensitivity encoding) and the Halfscan (half-Fourier acquisition) techniques were used to reduce the scan time. The aim of this study was to assess the clinical feasibility and diagnostic accuracy of this whole heart coronary MRA for recognizing stenoses of the coronary artery in comparison to conventional catheter coronary angiography.
From May to August 2004, we performed the whole heart coronary MRA on 42 patients with various cardiac diseases, and these patients were referred for cardiac MRI. Twenty-one of these patients (15 males and 6 females, the mean age 54.2 years, the age range was 26-79 years), who underwent the conventional catheter coronary angiography within one week after the whole heart coronary MRA examination, were enrolled in this study. The underlying diseases were coronary artery obstructive disease (n = 11), hypertrophic cardiomyopathy (n = 3), dilated cardiomyopathy (n = 2), valvular heart disease (n = 3) and congenital heart disease (n = 2). The whole heart coronary MRA failed to achieve acceptable results in two (4.8%) of the 42 patients because of arrhythmia in one and inability to breathe regularly in the other patient.
MRI was performed using a 1.5-T imaging unit (Gyroscan Intera, Philips Medical Systems, Best, Netherlands) that was equipped with a dedicated cardiac software package, a synergy cardiac coil and a vectorcardiogram.
The survey images were taken using the b-TFE sequence to determine the position of the heart. A SENSE reference image was then obtained. High temporal resolution cine imaging (50 phases per cardiac cycle) in the four-chamber view was performed then with using a balanced fast-field-echo (b-FFE) sequence to determine the subject-specific trigger delay time, when the motion of the right coronary artery was minimal. To compensate for the respiratory motion, a prospective diaphragmatic navigator gating was used in the craniocaudal direction with the 5 mm-gating window. The 3D imaging slab was positioned from the lower one third of the main pulmonary artery to the diaphragmatic surface of the heart with using the coronal survey image, and then the source images of MRA were acquired along a transverse axis.
The coronary MRA sequence began with a flow-insensitive T2 preparation pulse for the myocardial signal suppression; this was followed by the spectrally selective fat saturation pulse (SPIR), a navigator pulse and one more SPIR, after which the 3D b-TFE was acquired. An imaging volume of 12 cm was covered (80 contiguous slices with a reconstructed slice thickness of 1.5 mm). The other imaging parameters were as follows: a TR of 5.0 msec, a TE of 2.5 msec, a flip angle of 110°, an acquisition matrix 272×272, a reconstruction matrix 512×512, and a field of view of 270×270 mm. SENSE (factor 2) and the half-Fourier acquisition technique (Halfscan) were used to reduce the acquisition time.
Conventional catheter coronary angiography was performed, and its results were analyzed by two experienced cardiologists. The coronary vasculature was subdivided into 27 numbered anatomic segments with using the standardized nomenclature of the coronary artery surgery trial (11). The diameters of the narrowest portions of the lesions and of the adjacent healthy segment were manually measured in the diastolic phase with using a digital micrometer, and the severity of the coronary artery stenosis was expressed as a percentage reduction in the luminal diameter. A stenosis of > 50% in the vessel diameter was considered as significant.
The source images (e.g. Figs.1A, 1B, 2A, 2B) and the reconstructed MRA images (volume rendering and multiplanar reformation) (e.g. Figs. 1C, 1D, 2C) were evaluated by consensus between the two cardiac radiologists who were blinded to the catheter coronary angiography results. 3D image reformation was performed using VoxelPlus (Mevisys, Daejeon, Korea).
Ten coronary segments were evaluated in each heart: the left main coronary artery (LM) and the proximal, middle and distal segments of the left anterior descending coronary artery (LAD), the left circumflex coronary artery (LCX) and the right coronary artery (RCA). The ten segments corresponded to segments 11, 12, 13, 14, 18, 19, 19a, 1, 2, and 3 of the catheter coronary angiogram, respectively. For each segment, the image quality was visually graded as 0 - indicating uninterpretable (coronary artery nearly invisible); 1 - poor (coronary artery visible, but with markedly blurred vessel edges); 2- fair (moderately blurred vessel edges); 3 - good (mildly blurred vessel edges); 4 - excellent (sharply defined vessel edges); this scale is similar to the scales used by other researchers (1, 5, 12). The overall image quality of each coronary artery (LAD, LCX and RCA) was obtained by averaging the segment results. The segments with an acceptable image quality (grades≥2) were used to diagnose coronary artery stenosis. Segments with an artifact caused by a metallic coronary stent were excluded from the analysis. The degree of stenosis was qualitatively defined as significant or insignificant (normal or minimal) stenosis. Focal areas of marked signal loss, signal reduction or stenosis of > 50% in the vessel diameter were used as the criteria for significant stenosis (12, 13). No quantitative measurement of stenosis was performed.
The mean image quality of each coronary artery (LM, LAD, LCX and RCA) was calculated. Statistical differences in the image quality of the four coronary arteries were obtained by ANOVA. The sensitivity, specificity, accuracy and the positive and negative predictive values of the whole heart coronary MRA for the detection of significant coronary artery stenosis were determined, and then they were compared with the conventional X-ray coronary angiogram as the gold standard.
The mean acquisition time of the whole heart coronary MRA was 9 minutes 22 seconds (±125 seconds), and this was dependent on the heart rate and the navigator efficiency. The mean heart rate of the patients was 66.2 (±14.6)/min.
A total of 210 segments were obtained in these 21 patients. Of these, 9 segments that contained a metallic stent were excluded from the analysis.
Using the devised 5-point scale, the overall image quality was 3.0±0.1. Acceptable image quality was acquired for most of the proximal and middle coronary artery segments, and 168 (84%) of the 201 segments had an image quality of ≥ grade 2 (fair). The mean image quality was 3.7 in the LM, 3.2 in the LAD, 2.5 in the LCX, and 3.3 in the RCA. Of all the individual coronary arteries, the image quality of the LCX was significantly lower than that of other arteries (p < 0.05). The distal segments of the LAD and LCX had a significantly poorer image quality versus the other segments (p < 0.05); however, the distal segment of the RCA showed a relatively good image quality. The image quality of the coronary artery and the arterial segments is summarized in Table 1.
One hundred and sixty-eight of 201 segments in 21 patients with an image quality > grade 2 (fair) were assessed.
On the whole heart coronary MRA, 143 segments were normal or insignificantly narrowed, and 140 of these cases showed no signs of significant stenosis by the catheter coronary angiography (negative predictive value, 97.9%) (Fig. 1). According to the catheter coronary angiography, significant stenosis was identified in 16 segments. Of these 16 segments, 13 segments were correctly diagnosed to have significant stenosis by the whole heart coronary MRA (sensitivity: 81.3%) (Fig. 2). The specificity, accuracy and the positive predictive value of the whole heart coronary MRA were 92.1%, 91.1% and 52.0%, respectively. The values of the accuracy in the different coronary arteries were not significantly different (85.7% in the LM, 84.1% in the LAD, 90.7% in the LCX and 90.6% in the RCA). The data on the coronary arteries are summarized in Table 2.
Coronary MRA has definite advantages, namely, the absence of radiation exposure and the nephrotoxicity that is due to the iodine contrast material. However, its long scan time and the sophistication of the techniques, in comparision with CT coronary angiography with using multi-detector CT, have impeded its application in routine practice.
The weak points of evaluating the coronary arteries by MRI are associated with the cardiac motion, the respiratory motion and the small size and tortuous courses of the vessels (14, 15). In order to overcome the cardiac and respiratory motion, vectorcardiogram triggering and respiratory navigators have been generally applied (16-19). Because of the higher signal-to-noise ratio (SNR), a better ability to reduce the partial volume effect, the higher spatial resolution and the possibility of multiplanar reformatting, the 3D acquisition techniques are more frequently used than the 2D techniques (20, 21).
The free breathing 3D gradient echo technique with targeted double-oblique volume acquisition is widely used in diagnostic practice (5-10). This technique usually uses a 3 cm thick slab along the course of each major coronary artery, and it provides only one view of the major coronary artery. Therefore, the side branches, which are not included in the slab, cannot be imaged, and scans should be performed at least three times to image the three major coronary vessels.
In the present study, we attempted to increase the slab thickness to 12 cm to cover all the coronary arteries in one scan, including the small branches. In the 3D MR technique, when a larger volume (imaging slab thickness) is covered, then the SNR is increased, but the scan time is extended (21) as a result. We presumed that the SNR would be increased, and all the coronary arteries and their branches would be included if we increased the thickness of the 3D imaging slab to cover the whole heart. In addition, we used the parallel imaging technique SENSE and the half-Fourier acquisition (Halfscan) method to reduce the scan time. SENSE is an imaging technique that allows faster scanning by using signals from multiple receiver coils to reduce the number of the projections that are measured (22, 23), and usually it changes neither the imaging sequence itself nor the resulting image contrast. However, due to a reduced overall acquisition time, the SNR is reduced by the square root of the SENSE reduction factor (22). Half-Fourier acquisition is a method whereby approximately only one half of the acquisition matrix in the phase encoding direction is acquired; thus, the scan time is reduced. Moreover, half-Fourier acquisition does not affect the fine resolution of the image, but it does reduce the SNR by the square root of 2. We presumed that widening the slab thickness would compensate for the presumed loss of the SNR that's caused by the use of SENSE and half-Fourier acquisition. As expected, the mean scan time (9.4 minutes) proved adequate, and the image quality and the diagnostic accuracy of the whole heart coronary MRA were also acceptable in the current study, as compared with previous reports that used other MRA techniques (1-3, 5, 24). Kim et al. (1) reported a prospective multicenter study using 3D coronary MRA with the targeted volume acquisition technique, in which the sensitivity, specificity, accuracy and the negative predictive value for patients with disease of the LM or three vessel disease were 100%, 85%, 87% and 81%, respectively. Recently, Sakuma et al. (25) have reported a prospective study using whole heart coronary MRA that was similar to our technique. In their study, the sensitivity and specificity of the coronary MRA for detecting significant stenosis were 82% and 91%, respectively. They used SENSE (factor 3) to reduce the scan time, and the average imaging time was 13.8 minutes. We also used the half-Fourier acquisition technique, as well as the SENSE technique (factor 2); therefore we could reduce the mean scan time to 9.4 minutes. We presumed that other methods could be used to reduce the scan time, e.g., control of the acquisition window in the RR interval, or the scan time could be reduced by improving the navigator efficiency.
In the current study, the image quality of the LCX was significantly poorer than the image quality of the other arteries (LM, LAD and RCA). Among the segments, the distal segments of the LCX and LAD displayed poorer quality than the other segments. When the distal segments were excluded, the image quality between the coronary arteries was not statistically different. We believe that the poor quality of the LCX was caused by the small diameter of its distal segment.
The advantages of the whole heart coronary MRA are as follows. The technique enables all the coronary arteries and side branches to be covered by one scan within a reasonable scan time without compromising the spatial resolution and SNR. Although the spatial resolution was not sufficient to evaluate stenoses of the small side branches, we were able to obtain anatomical information on the side branches. The reduced scan time allows this MRA sequence to be additionally used in patients who are examined by cardiac MRI for other purposes; therefore, the purely diagnostic use of conventional catheter coronary angiography can be significantly reduced. Moreover, various post-processing reformation techniques (e.g. volume rendering, multiplanar reformation and maximum intensity projection) can be applied like in multi-detector CT coronary angiography.
The first drawback of this technique is that the trigger delay time is determined by one coronary artery, as opposed to the coronary MRA with using the targeted volume acquisition technique, in which the trigger delay time is determined by each targeted coronary artery. We determined the trigger delay time based on the minimal RCA motion, because the RCA, which is located along the atrioventricular groove, shows the widest positional change during the cardiac cycle (26). In our study, the image quality was not significantly different between the different coronary arteries, except for the LCX, and this was attributed to the vessel's size rather than to the trigger delay time. The second drawback concerns the spatial resolution. Some of the distal segments and side branches of the coronary arteries were not clearly visualized. We used a 1.5 mm slice thickness because of the time limitation; for example, if we had used a 0.75 mm slice thickness for a better spatial resolution, the scan time would have been longer than 20 minutes. We believe that the application of high field strength MRI or a highly efficient navigator technique may overcome this limitation, and a recent report has demonstrated such a possibility (27-29). The third drawback is that low navigator efficiency (due to irregular breathing, patient's non-cooperation, sleep during scanning, etc.) or arrhythmia can deteriorate the overall image quality, as compared with multi-detector CT coronary angiography, in which the image is degraded only in the portion taken during the arrhythmic heart beat. In our study, the coronary MRA failed in two patients because of arrhythmia in one patient and the inability to breathe regularly in the other patient.
Our study shows that using whole heart coronary MRA for diagnosing coronary artery stenosis has a high negative predictive value and diagnostic accuracy, and we suggest that the technique may play a certain role in excluding clinically significant coronary disease, especially for the intermediate to low risk patients.
A drawback of our study is the small sample size, especially the size of the positive population; therefore, statistical evaluation of the sensitivity and positive predictive value is limited. Further studies are required to determine the diagnostic value of whole heart coronary MRA. Another limitation of this study is that we failed to evaluate the degree of stenoses quantitatively because the blurred vessel margin and signal loss in the cases of severe stenosis made it difficult to quantify the degree of stenosis. We expect that better spatial resolution with using a more efficient motion-adapted navigator gating, high Tesla equipment or intravascular contrast agent could resolve this limitation.
Figures and Tables
![]() | Fig. 1A 48-year-old woman who was diagnosed with hypertrophic cardiomyopathy.
Source images (A, B), the multiplanar reformatted image (C) and the volume rendering image (D) of whole heart coronary MRA show normal major epicardial coronary arteries.
|
![]() | Fig. 2A 53-year-old man who presented with chest pain.
A, B. Source images of whole heart coronary MRA demonstrate a signal loss (arrow in B) at the middle segment of the right coronary artery, which is indicative of significant stenosis.
C. planar reformatted image along the right coronary artery course shows an abrupt signal loss at the middle segment of the right coronary artery (arrow) and multifocal signal reduction (arrowheads) at the proximal portion.
D. Conventional catheter coronary angiography shows a total occlusion of the middle segment of the right coronary artery and multifocal mild stenosis of the proximal and middle segments.
|
Table 1
Quality of the Images of Whole Heart Coronary MRA
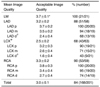
Note.-Image quality was graded as 0 = uninterpretable, 1 = poor, 2 = fair, 3 = good or 4 = excellent; *= standard error of the mean; †= Image quality of the LCX was significantly lower (p < 0.05) than that of the other arteries; ‡= Image quality of the distal segments of the LAD and LCX was significantly lower (p < 0.05) than that of the other segments; p = proximal; m = middle; d = distal
References
1. Kim WY, Danias PG, Stuber M, Flamm SD, Plein S, Nagel E, et al. Coronary magnetic resonance angiography for the detection of coronary stenoses. N Engl J Med. 2001. 345:1863–1869.
2. Weber C, Steiner P, Sinkus R, Dill T, Bornert P, Adam G. Correlation of 3D MR coronary angiography with selective coronary angiography: feasibility of the motion-adapted gating technique. Eur Radiol. 2002. 12:718–726.
3. Sardanelli F, Molinari G, Zandrino F, Balbi M. Three-dimensional, navigator-echo MR coronary angiography in detecting stenoses of the major epicardial vessels, with conventional coronary angiography as the standard of reference. Radiology. 2000. 214:808–814.
4. Sandstede JJ, Pabst T, Wacker C, Wiesmann F, Hoffmann V, Beer M, et al. Breath-hold 3D MR coronary angiography with a new intravascular contrast agent (feruglose)-first clinical experiences. Magn Reson Imaging. 2001. 19:201–205.
5. Giorgi B, Dymarkowski S, Maes F, Kouwenhoven M, Bogaert J. Improved visualization of coronary arteries using a new three-dimensional submillimeter MR coronary angiography sequence with balanced gradients. AJR Am J Roentgenol. 2002. 179:901–910.
6. Maintz D, Aepfelbacher FC, Kissinger KV, Botnar RM, Danias PG, Heindel W, et al. Coronary MR angiography: comparison of quantitative and qualitative data from four techniques. AJR Am J Roentgenol. 2004. 182:515–521.
7. Stehning C, Bornert P, Nehrke K, Eggers H, Dossel O. Fast isotropic volumetric coronary MR angiography using free-breathing 3D radial balanced FFE acquisition. Magn Reson Med. 2004. 52:197–203.
8. Bunce NH, Lorenz CH, Keegan J, Lesser J, Reyes EM, Firmin DN, et al. Coronary artery anomalies: assessment with free-breathing three-dimensional coronary MR angiography. Radiology. 2003. 227:201–208.
9. Spuentrup E, Buecker A, Stuber M, Botnar R, Nguyen TH, Bornert P, et al. Navigator-gated coronary magnetic resonance angiography using steady-state-free-precession: comparison to standard T2-prepared gradient-echo and spiral imaging. Invest Radiol. 2003. 38:263–268.
10. Spuentrup E, Bornert P, Botnar RM, Groen JP, Manning WJ, Stuber M. Navigator-gated free-breathing three-dimensional balanced fast field echo (TrueFISP) coronary magnetic resonance angiography. Invest Radiol. 2002. 37:637–642.
11. Scanlon PJ, Faxon DP, Audet AM, Carabello B, Dehmer GJ, Eagle KA, et al. ACC/AHA guidelines for coronary angiography. A report of the American College of Cardiology/American Heart Association Task Force on practice guidelines (Committee on Coronary Angiography). Developed in collaboration with the Society for Cardiac Angiography and Interventions. J Am Coll Cardiol. 1999. 33:1756–1824.
12. Duerinckx AJ, Urman MK. Two-dimensional coronary MR angiography: analysis of initial clinical results. Radiology. 1994. 193:731–738.
13. Manning WJ, Li W, Edelman RR. A preliminary report comparing magnetic resonance coronary angiography with conventional angiography. N Engl J Med. 1993. 328:828–832.
14. Yang PC, McConnell MV, Nishimura DG, Hu BS. Magnetic resonance coronary angiography. Curr Cardiol Rep. 2003. 5:55–62.
15. Flamm SD, Muthupillai R. Coronary artery magnetic resonance angiography. J Magn Reson Imaging. 2004. 19:686–709.
16. Chia JM, Fischer SE, Wickline SA, Lorenz CH. Performance of QRS detection for cardiac magnetic resonance imaging with a novel vectorcardiographic triggering method. J Magn Reson Imaging. 2000. 12:678–688.
17. Fischer SE, Wickline SA, Lorenz CH. Novel real-time R-wave detection algorithm based on the vectorcardiogram for accurate gated magnetic resonance acquisitions. Magn Reson Med. 1999. 42:361–370.
18. Nagel E, Bornstedt A, Schnackenburg B, Hug J, Oswald H, Fleck E. Optimization of realtime adaptive navigator correction for 3D magnetic resonance coronary angiography. Magn Reson Med. 1999. 42:408–411.
19. Liu YL, Riederer SJ, Rossman PJ, Grimm RC, Debbins JP, Ehman RL. A monitoring, feedback, and triggering system for reproducible breath-hold MR imaging. Magn Reson Med. 1993. 30:507–511.
20. Hofman MB, Paschal CB, Li D, Haacke EM, van Rossum AC, Sprenger M. MRI of coronary arteries: 2D breath-hold vs 3D respiratory-gated acquisition. J Comput Assist Tomogr. 1995. 19:56–62.
21. Li D, Paschal CB, Haacke EM, Adler LP. Coronary arteries: three-dimensional MR imaging with fat saturation and magnetization transfer contrast. Radiology. 1993. 187:401–406.
22. Pruessmann KP, Weiger M, Scheidegger MB, Boesiger P. SENSE: sensitivity encoding for fast MRI. Magn Reson Med. 1999. 42:952–962.
23. Sodickson DK. Tailored SMASH image reconstructions for robust in vivo parallel MR imaging. Magn Reson Med. 2000. 44:243–251.
24. Ikonen AE, Manninen HI, Vainio P, Hirvonen TP, Vanninen RL, Matsi PJ, et al. Three-dimensional respiratory-gated coronary MR angiography with reference to X-ray coronary angiography. Acta Radiol. 2003. 44:583–589.
25. Sakuma H, Ichikawa Y, Suzawa N, Hirano T, Makino K, Koyama N, et al. Assessment of coronary arteries with total study time of less than 30 minutes by using whole-heart coronary MR angiography. Radiology. 2005. 237:316–321.
26. Achenbach S, Ropers D, Holle J, Muschiol G, Daniel WG, Moshage W. In-plane coronary arterial motion velocity: measurement with electron-beam CT. Radiology. 2000. 216:457–463.
27. Huber ME, Kozerke S, Pruessmann KP, Smink J, Boesiger P. Sensitivity-encoded coronary MRA at 3T. Magn Reson Med. 2004. 52:221–227.
28. Weber OM, Martin AJ, Higgins CB. Whole-heart steady-state free precession coronary artery magnetic resonance angiography. Magn Reson Med. 2003. 50:1223–1228.
29. Du YP. Prospective navigator gating with a dual acceptance window technique to reduce respiratory motion artifacts in 3D MR coronary angiography. Int J Cardiovasc Imaging. 2003. 19:157–162.