Abstract
Objective
We wanted to estimate the practical improvements of 3T proton MR spectroscopy (1H MRS) as compared with 1.5T 1H MRS for the evaluation of human brain tumors.
Materials and Methods
Single voxel 1H MRS was performed at both 1.5T and 3T in 13 patients suffering with brain tumors. Using the same data acquisition parameters at both field strengths, the 1H MRS spectra were obtained with a short echo time (TE) (35 msec) and an intermediate TE (144 msec) with the voxel size ranging from 2.0 cm3 to 8.7 cm3. The signal to noise ratios (SNRs) of the metabolites (myoinositol [MI], choline compounds [Cho], creatine/phosphocreatine [Cr], N-acetyl-aspartate [NAA], lipid and lactate [LL]) and the metabolite ratios of MI/Cr, Cho/Cr, Cho/NAA and LL/Cr were compared at both TEs between the two field strengths in each brain tumor. The degrees of spectral resolution between the Cho and Cr peaks were qualitatively compared between the two field strengths in each brain tumor.
Results
The SNRs of the metabolites at 3T demonstrated 49-73% increase at a short TE (p < 0.01) and only 2-12% increase at an intermediate TE (p > 0.05) compared with those of 1.5T. The SNR of inverted lactate at an intermediate TE decreased down to 49% with poorer inversion at 3T (p < 0.05). There was no significant difference in the metabolite ratios between the two field strengths. The degrees of the spectral resolution at 3T were slightly superior to those of 1.5T at a short TE.
Conclusion
As compared with 1.5T, 3T 1H MRS demonstrated 49-73% SNR increase in the cerebral metabolites and slightly superior spectral resolution only at a short TE, but little at an intermediate TE, in the brain tumors. There was no significant difference in the metabolite ratios between the two field strengths.
In vivo proton magnetic resonance spectroscopy (1H MRS) provides completely different information on the basic metabolic processes such as cell proliferation or degradation, energy metabolism, neuronal integrity and necrotic transformation of the brain tumor tissues, and this stands in contrast to the methods that provide structural data, such as MRI, CT and angiography (1-3).
The spectroscopic studies on brain tumors have been attempted to characterize the different histologic types and predict the degree of malignancy of the gliomas. Noninvasive grading is especially important for the tumors in the eloquent areas or for the deeply located tumors. However, despite the expectations of noninvasively characterizing tumor by performing clinical 1H MRS, some of previous studies have yielded rather disappointing results that there was no reliable indicator for discriminating among the tumor types or among the malignant and benign neoplasms (2, 4, 5). This may be partially due to the limited spatial and spectral resolution obtained at the standard magnetic field of 1.5T (3).
With the integration of 3T MRI into clinical practice, there is growing interest in the practical improvement of 1H MRS at a 3T field strength with respect to the established magnetic field strength of 1.5T because both the spectral resolution and the spatial resolution depend, in a linear fashion, on the magnetic field (6-9).
Although single-voxel 1H MRS of the human brain has been performed at many field strengths from 0.5T to 7T, there have been few direct comparisons between the current standardized 1.5T and 3T in the same human brain or in phantoms with using the same methodology (6, 7, 9). According to those reports, the expected gains in the signal to noise ratio (SNR) and the spectral resolution were partially achieved in the normal human brain due to many factors at a higher field strength (6, 7). We tried to evaluate practical improvements of 1H MRS at 3T as compared with 1.5T in patients suffering with brain tumor in terms of the SNR and the spectral resolution.
Our study included 13 patients (6 men and 7 women, age range: 18-69 years, mean age: 45.9) with a previously untreated brain tumor. The postoperative diagnosis of brain tumors included three glioblastomas, two anaplastic astrocytomas, two anaplastic oligodendrogliomas, one oligodendroglioma, one metastasis, one lymphoma, one pineocytoma, one central neurocytoma and one meningioma. All the patients provided us with an informed consent for participation in the study, and this study was approved by our institutional review board.
All the 1H MRS studies were performed by using the automated single-voxel MRS package Proton Brain Examination/Single Voxel with both 1.5T and 3T scanning units (GE Medical Systems, Milwaukee, WI) that were equipped with circularly polarized head coils. In each patient, we obtained the spectra from voxels of the same size that contained the same area by using the point-resolved spectroscopy (PRESS) pulse sequences with the same repetition time (TR) and echo time (TE); there was a two week interval between the 1.5T and 3T examinations. The parameters of the short TE (2,000/35) (TR/TE) and intermediate TE (1,500/144) sequences were applied to the examinations with both fields.
MR imaging with the T2-weighted fast spin echo sequences (4,000-5,000/104-121, 24-cm field of view, 256×224 matrix and 5-mm sections) in the axial or coronal planes preceded 1H MRS in order to define the volume of interest (VOI). The VOIs varied from 2.0 cm3 to 8.7 cm3 depending on the tumor size. The size and location of the voxel were carefully adjusted to include as much of either the nonenhancing or homogeneously enhancing solid portion as possible, as was determined from the previously obtained MR images. We intended to avoid inclusion of the voxels of the obvious necrosis, cyst, hemorrhage, edema, calcification and normal appearing brain. All the 1H MRS examinations were performed without administration of contrast agent.
All spectra were processed using Mrdx (CAD Impact, Inc., Seoul, Korea) based on IDL (Research Systems, Inc., Boulder, CO). Typical full widths at half maximum of 3 to 6 Hz were achieved on most examinations. The water signal was suppressed by a frequency-selective saturation pulse at the water resonance. A sweep width of 1,000 Hz was used with a data size of 1,024 points. Only the second half of the echo was acquired. Following the zero-filling of 8,192 points in all the free induction-decay data, an exponential line broadening (center: 0 milliseconds, half time: 150 milliseconds) was done before Fourier transformation. Zero-order phase correction was applied to all spectra.
For the semiquantitative evaluation, we estimated the levels of myoinositol (MI), choline compounds (Cho), creatine/phosphocreatine (Cr), and N-acetyl-aspartate (NAA) as the heights of the peaks at 3.6, 3.2, 3.0, and 2.0 ppm in respect to both TEs of the two field strengths. We defined and estimated the sum of the peak heights between 0.9 and 1.3 ppm as lipid and lactate (LL) at the short TE. We defined and estimated the sum of the upright lipid peak at around 0.9-1.3 ppm and also the absolute value of the inverted lactate peak at 1.3 ppm as the LL at the intermediate TE.
In order to compare the degree of inversion of lactate at the intermediate TE between the two fields, we estimated the absolute value of the inverted lactate peak at 1.3 ppm on both fields in seven patients who showed lactate inversion at the intermediate TE on 1.5T. The SNRs of all the metabolites were estimated by taking the ratios of the metabolite peak height to the standard deviation of the noise (δ noise), which was estimated from the signal-free 0 to -5 ppm region.
The metabolite ratios of MI/Cr, Cho/Cr, Cho/NAA and LL/Cr were also calculated. For the qualitative evaluation of spectral resolution, the degree of baseline Cho-Cr separation was also visually evaluated. The 3T/1.5T ratios of each metabolite's SNR and the metabolite ratios at both fields were described as means±standard deviations (SD).
SPSS (version 11.0; SPSS Inc, Chicago, lL) was used for statistical analysis.
Nonparametric tests were applied throughout, as the number of cases was not sufficiently high to assume a normal distribution. The Wilcoxson signed rank tests for matched pairs were performed to compare the SNRs of the metabolites and the metabolite ratios at both TEs between the 1.5T and 3T. P values less than 0.05 were considered to indicate a statistically significant difference.
The 3T/1.5T SNR ratios of the metabolites at both TEs are given in Figure 1 and Table 1. At a short TE, the SNRs of the metabolites at a 3T strength increased, i.e., 49.4% for NAA and 72.6% for LL (p < 0.01) and the SNRs were better in all the patients as compared with the SNRs at 1.5T (Fig. 2). However, at an intermediate TE, the SNRs of the metabolites at 3T increased only 2.4% and 11.6% for NAA, Cr, respectively (p > 0.05). There were rather worse SNRs at the intermediate TE of 3T in six patients whose tumors were located near the calvarium and/or they had portions of hemorrhage (Fig. 3).
In seven patients who showed lactate inversion at an intermediate TE on 1.5T, the SNRs of the inverted lactate decreased down to 49.2±18.5% at 3T. Out of the seven patients, the inverted lactate peaks were indiscernible in four patients and they were smaller in three patients at 3T (Figs. 2 and 3).
The mean metabolite ratios at both TE sequences at 3T and 1.5T are given in the Table 2. There was no statistical difference in any of the metabolite ratios between both fields.
For the qualitative assessment of spectral resolution at 3T, seven out of 13 patients showed better Cho-Cr separation at a short TE and three patients with brain tumor showed at an intermediate TE as compared with 1.5T (Fig. 2).
In the present study, the theoretical gain of twofolds for the SNR of each metabolite was not fully realized with 3T. A partial gain (49.4-72.6%) was obtained at a short TE with 3T as compared with 1.5T. At an intermediate TE, there was little significant SNR gain with 3T. The spectral resolution was also partly improved, and particularly at a short TE with 3T.
Barker et al. (7) reported that the spectra of the normal human brains at 3T demonstrated a 20% improvement of the SNR compared to 1.5T at a short TE (20 msec); however the spectra at a long TE (272 msec) exhibited a similar SNR at both field strengths with using the stimulated-echo acquisition mode (STEAM) sequences. Despite the expected advantages that should be obtained with using high strength fields, the limited SNR improvements might be explained by many factors. They include increased magnetic field inhomogeneity, poorer radiofrequency coil efficiency, poorer shimming and different relaxation times (6, 7, 9, 10). Therefore, in order to realize the theoretical improvements, these factors should be overcome and adjusted for at a field strength of 3T.
The T1 relaxation times increase at higher fields, leading to increased signal saturation for a given TR. To maintain the same level of saturation, the acquisition TR should be lengthened with increasing the total acquisition times or decreasing the signal averaging in the same total acquisition time. As the T2 relaxation times decrease at higher fields, the TE should be shortened. However the optimal TR and TE at 3T have not yet been well established.
Barker et al. (7) explained that the similar SNR at a long TE on both fields was because the long TE sequence was more influenced by the decreased T2 relaxation time in the high field than it being influenced by the short TE sequence.
The SNR gain seen at a short TE in the present study was larger than that of Barker's study. This was presumably because for PRESS, there was much less signal loss of the coupled spin signals than for STEAM (11, 12).
Gonen et al. (6) reported that there was a 23-46% SNR gain at a TE of 135 ms with 3T versus 1.5T in the 3D chemical shift imaging with using the PRESS sequence in the normal human brain, and this is in contrast to the results of the small SNR gain at an intermediate TE in the present study with brain tumor patients. It is postulated that the tumor's own heterogeneity might offset the expected gain of the SNR as compared with the normal human brain at the intermediate or long TE sequences. Tumors are inherently heterogeneous and their spectra have been described as a combination of viable tumor with both necrotic and cystic tissue, as well as the infiltration of the normal brain tissue. The involvement of paramagnetic material in tumor tissue such as intratumoral microscopic hemorrhage may also cause a susceptibility effect and so cause T2 shortening. Blood vessels and cerebrospinal fluid increase the magnetic susceptibility effect by pulsation as well (12, 13).
As for the inverted lactate peak at 1.3 ppm of the intermediate TE spectrum, the SNR on 3T was rather much smaller than that of 1.5T in the present study. This might be related to the increased susceptibility and the T2 shortening effect with 3T.
For lactate inversion, the intermediate TE value used at 1.5T might not be appropriate at 3T. Because there was no significant difference in the metabolite ratios (MI/Cr, Cho/Cr, Cho/NAA and LL/Cr) between the two fields, we may well apply the established methods of 1.5T concerning the metabolite ratios for the evaluation of brain tumors at 3T.
For the 3T 1H MRS of brain tumors, there were 49-73% SNR increases of the major cerebral metabolites and also better spectral resolution (n = 7/13) at a short TE (35 msec): however, little at an intermediate TE (144 msec), compared with 1.5T. The SNR of inverted lactate at an intermediate TE decreased to 49% at 3T. There was no significant difference in the metabolite ratios of MI/Cr, Cho/Cr, Cho/NAA and LL/Cr between 1.5T and 3T.
Figures and Tables
Fig. 1
Standard error graph of the 3T/1.5T signal to noise ratios of each metabolite in the patients with brain tumor (n = 13). The small square is the mean, and the upper and lower transverse lines show the 95% values of the data. The 3T/1.5T signal to noise ratios of all the metabolites are significantly increased at a short echo time: however, they were not at an intermediate echo time.
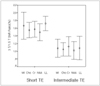
Fig. 2
A 40-year-old woman with anaplastic astrocytoma.
A. The axial T2-weighted image reveals a mass of high signal intensity with a square voxel (7.1 cm3) at the left high frontal lobe.
B. The 1H MR spectrum obtained at a short TE on 1.5T shows markedly increased Cho/Cr, decreased NAA/Cr and increased lipid and lactate.
C. The 1H MR spectrum obtained at an intermediate TE on 1.5T shows an inverted lactate peak at 1.3 ppm in addition to increased Cho/Cr.
D. The 1H MR spectrum obtained at a short TE on 3T shows a better SNR and better spectral resolution between Cho and Cr than on 1.5T (B), and it is otherwise similar to 1.5T (B).
E. The 1H MR spectrum obtained at an intermediate TE on 3T shows an inverted lactate peak at 1.3 ppm that is significantly smaller than that of 1.5T (C).
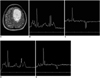
Fig. 3
A 49-year-old man with anaplastic oligodendroglioma.
A. The axial T2-weighted image reveals a heterogenous mass with probable hemorrhagic portion in the right frontal lobe. A rectangular voxel (2.3 cm3) contains the solid tumor portion, including a small possibly hemorrhagic area, and it is located close to the calvarium.
B. The 1H MR spectrum obtained at a short TE on 1.5T shows slightly elevated Cho/Cr and decreased NAA/Cr with many noisy signals.
C. The 1H MR spectrum obtained at an intermediate TE on 1.5T shows elevated Cho/Cr, decreased NAA/Cr and an inverted lactate peak (1.3 ppm).
D. The 1H MR spectrum obtained at a short TE on 3T shows slightly elevated Cho/Cr and decreased NAA/Cr with a slightly better SNR and shimming compared with the 1.5T (B).
E. The 1H MR spectrum obtained at an intermediate TE on 3T shows much more noise than that of the 1.5T (C) and there is no discernible inverted lactate peak at 1.3 ppm, and this is presumably due to the high susceptibility effect secondary to probable intratumoral hemorrhage and the close proximity to the calvarium.
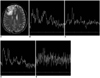
References
1. Moller-Hartmann W, Herminghaus S, Krings T, Marquardt G, Lanfermann H, Pilatus U, et al. Clinical application of proton magnetic resonance spectroscopy in the diagnosis of intracranial mass lesions. Neuroradiology. 2002. 44:371–381.
2. Shimizu H, Kumabe T, Tominaga T, Kayama T, Hara K, Ono Y, et al. Noninvasive evaluation of malignancy of brain tumors with proton MR spectroscopy. AJNR Am J Neuroradiol. 1996. 17:737–747.
3. Rabinov JD, Lee PL, Barker FG, Louis DN, Harsh GR, Cosgrove GR, et al. In vivo 3-T MR spectroscopy in the distinction of recurrent glioma versus radiation effects: initial experience. Radiology. 2002. 225:871–879.
4. Ott D, Hennig J, Ernst T. Human brain tumor: assessment with in vivo proton MR spectroscopy. Radiology. 1993. 186:745–752.
5. Barker PB, Glickson JD, Bryan RN. In vivo magnetic resonance spectroscopy of human brain tumors. Top Magn Reson Imaging. 1993. 5:32–45.
6. Gonen O, Gruber S, Li BS, Mlynarik V, Moser E. Multivoxel 3D proton spectroscopy in the brain at 1.5 versus 3.0T: signal-to-noise ratio and resolution comparison. AJNR Am J Neuroradiol. 2001. 22:1727–1731.
7. Barker PB, Hearshen DO, Boska MD. Single-voxel proton MRS of the human brain at 1.5T and 3.0T. Magn Reson Med. 2001. 45:765–769.
8. Gruber S, Mlynarik V, Moser E. High-resolution 3D proton spectroscopic imaging of the human brain at 3T: SNR issues and application for anatomy-matched voxel sizes. Magn Reson Med. 2003. 49:299–306.
9. Kantarci K, Reynolds G, Petersen RC, Boeve BF, Knopman DS, Edland SD, et al. Proton MR spectroscopy in mild cognitive impairment and Alzheimer disease: comparison of 1.5 and 3T. AJNR Am J Neuroradiol. 2003. 24:843–849.
10. Bartha R, Drost DJ, Menon RS, Williamson PC. Comparison of the quantification precision of human short echo time 1H spectroscopy at 1.5 and 4.0 tesla. Magn Reson Med. 2000. 44:185–192.
11. Kaminogo M, Ishimaru H, Morikawa M, Ochi M, Ushijima R, Tani M, et al. Diagnostic potential of short echo time MR spectroscopy of gliomas with single-voxel and point-resolved spatially localised proton spectroscopy of brain. Neuroradiology. 2001. 43:353–363.
12. Howe FA, Barton SJ, Cudlip SA, Stubbs M, Saunders DE, Murphy M, et al. Metabolic profiles of human brain tumors using quantitative in vivo 1H magnetic resonance spectroscopy. Magn Reson Med. 2003. 49:223–232.
13. Isobe T, Matsumura A, Anno I, Yoshizawa T, Nagatomo Y, Itai Y, et al. Quantification of cerebral metabolites in glioma patients with proton MR spectroscopy using T2 relaxation time correction. Magn Reson Imaging. 2002. 20:343–349.