Abstract
Objective
This study was designed to compare three different measures of the elderly human brain; the magnetization transfer ratio (MTR) histogram, the percentage of brain parenchymal volume, and the volume of T2 hyperintense areas in terms of correlations with the study subjects' neurocognitive performance.
Materials and Methods
Thirty-five healthy community-dwelling elderly volunteers aged 60-82 years underwent dual fast spin-echo (FSE) imaging and magnetization transfer imaging. A semi-automated technique was used to generate the MTR histogram, the brain parenchymal volume, and the T2 lesion volume. The subjects' neurocognitive performance was assessed by using the Korean-Mini Mental State Examination (K-MMSE) and additional tests. The peak height of the MTR (PHMTR), the percentage of brain parenchymal volume (PBV), and the normalized T2 lesion volume (T2LV) were compared between the normal group (Z score on the K-MMSE ≥ -2, n=23) and the mild cognitive impairment group (Z score on the K-MMSE < -2, n=12), and these parameters were correlated with age and various neurocognitive performance scores.
Results
The PHMTR was significantly lower in the cognitively impaired subjects than the PHMTR in the normal subjects (p = 0.005). The PBV scores were lower in the cognitively impaired subjects than in the normal subjects (p = 0.02). The T2LV scores were significantly higher in the cognitively impaired subjects (p = 0.01). An inverse correlation was found between the PHMTR and T2LV (r = -0.747, p < 0.001), and also between the PBV and T2LV (r = -0.823, p < 0.001). A positive correlation was observed between the PHMTR and the PBV (r = 0.846, p < 0.001). Scores on the various neurocognitive tests were positively correlated with the PHMTR (6 of 7 items) and the PBV (5 of 7 items), and they were negatively correlated with the T2LV (5 of 7 items).
MR imaging investigations of age-related cognition have typically involved white matter areas of T2 hyperintensity and the morphologic measures related to brain atrophy (1-3). However, conventional MR studies of age-related changes are only able to capture changes within the limits of macroscopic tissue changes. So, measures on conventional MR in normal subjects have frequently overlapped with those measures for patients having mild cognitive impairment (4-6).
Magnetization transfer (MT) MR imaging examinations have increased sensitivity for the detection of brain tissue damage that occurs at levels beyond the resolution capability of conventional imaging modalities (7). The magnetization transfer ratio (MTR), an index of MT imaging, reflects the efficiency of the magnetization exchange between the protons of water inside tissue and the protons bound to the macromolecules. These macromolecules decrease when brain tissue disorganization occurs owing to demyelination or axonal loss (8).
Over the past a few years, researchers have attempted to increase the use of MTR in cognitive disorders, and they have found that MTR of brain tissue correlated well with cognitive function and it had less overlap between controls and patients (9-11). They postulated that brain degeneration in neurodegenerative disorders would be more accurately reflected by MTR measures than morphologic changes, since the former could reveal microscopic tissue changes in the early stages. However, these studies were not performed in healthy elderly subjects for the assessment of superior methods by which age-related changes could be depicted more accurately for brain volume decreases, T2 lesion volumes and MTR, in terms of their correlation with cognitive performance. Our study was designed to compare three different measures [the peak height of the MTR histogram (PHMTR), the percentage of brain parenchymal volume (PBV), and normalized T2 lesion volume (T2LV)] with each other and between cognitive groups, and to correlate different measurements with cognitive performance in the healthy elderly human brain.
Thirty-five healthy community-dwelling elderly volunteers (20 women, 15 men; age range, 60-82 years; mean age, 69.8 years ± 5.8) were examined according to a rigid imaging protocol. The mean age of the female subjects (69.5 years ± 5.6) was not significantly different (p = 0.71) from the mean age of the male subjects (70.2 years ± 5.9). Participants who were enrolled in this study were screened by means of medical and neurological histories and physical examinations by an experienced neurologist. Exclusion criteria included a history of concussive head injury, long-term steroid use, brain mass, stroke, seizure, active psychiatric disorder, and active alcohol or substance abuse. All the participants were clinically stable and without any acute illness at the time of their entry to the study. Our institutional review board approved the study, and written informed consents were obtained from all participants.
MR imaging was performed with a 1.5 Tesla Siemens scanner. Contiguous transverse dual fast spin-echo (FSE) proton density (PD)-weighted and T2-weighted images were obtained with 3400/18/90 (TR/TE1/TE2), 3 mm section thickness (contiguous, interleaved), 22 cm field of view, 256×192 matrix, > 50 sections, and an echo train length of eight. The MT images were acquired using a 3D gradient-echo sequence (TR/TE, 36/10; flip angle 12°) that was modified by an additional MT pulse with a 5 mm section thickness, 22 cm field of view, and 256×192 matrix. MT contrast was achieved by the application of a 19-ms sync-shaped RF pulse during each TR. Pairs of consecutive transverse images, one with and one without the MT saturation pulse, were obtained and interpolated to a 3 mm section thickness. More than 50 sections were obtained in each subject to cover the whole brain. To minimize variations in the volume calculations for the whole brain parenchyma among subjects, we included only certain sections from the MR image sets: those images starting from the section just before the image that showed the cerebellum at the bottom and ending with the last section that showed brain at the top. MR imaging of the brain was performed within 2 days of the neuropsychological examinations.
The first (PD-weighted) and second (T2-weighted) echoes of the FSE sequence and MT image pairs in each study were transferred electronically to our workstation for image processing. An automated segmentation algorithm was applied for brain MR images, and it was incorporated in a software system called IDL (Version 5.4 Win 32, Research Systems, Inc.).
T2 lesion volume calculations were performed with a validated semiautomatic computerized method based on the concept of fuzzy connectedness (12) by using PD- and T2-weighted image sets. This method has been described previously and validated in several studies (12-14). Parenchyma and CSF volumes also were determined with the same system (Fig. 1). To summarize, PD- and T2-weighted images were used for segmentation as follows: the skull was stripped from the images, and the whole brain was segmented into CSF and parenchyma using a k-means cluster analysis (15). Manual editing to exclude any residual extracranial components followed this automated process. To normalize for baseline differences in the intracranial volumes among subjects, an additional parameter, the PBV (%), was calculated by dividing the brain parenchymal volume by the sum of the brain parenchymal volume and CSF volume. Another parameter, the T2LV (%), also was achieved by dividing the T2 lesion volume by the brain parenchymal volume for interpersonal normalization.
MTR histographic analysis was performed using the method previously described by van Buchem et al. (16). Magnetization transfer images were segmented to remove the extracranial contents. Then, for each voxel, the MTR was calculated by using the following expression: MTR = (Mo-Ms)/Mo×100%, where Mo and Ms represent the signal intensity of the voxel with the saturation pulse off and on, respectively. The CSF was separated from other intracranial voxels using a threshold of MTR = 5%. The MTR histograms for brain parenchyma were then generated, and the peak height was normalized by using the number of voxels with a certain MTR value divided by the total number of voxels for the entire tissue to account for variations in head size among the subjects. Thus, the PHMTR of the histogram represents the largest normalized frequency (i.e., the largest normalized pixel value among the spectrum of individual MTRs). An experienced neuroradiologist performed the image processing and the calculations. In repeated studies, the coefficients of intra- and inter-observer variation were shown to be less than 1.5% for the PBV, the T2LV, and the PHMTR.
Neuropsychological assessments were performed by using the Korean-Mini Mental State Examination (K-MMSE) and additional tests of attention, visual memory, verbal memory, language, visuospatial function and executive function. The K-MMSE test used in this study was the revised one derived from the MMSE developed by Folstein et al. (17), with adjustment for the Korean language and cultural features (18). Z scores of the K-MMSE were obtained according to the age and educational level from the reference values for the Korean population (19). Subjects (n=12) with a Z score lower than 2 were considered as cognitively impaired. All of these patients also met the criteria for "mild cognitive impairment" - a definition combining the DSM-3 criteria (decreases in short- or long-term memory, preserved intellect and no impairment in living function on a day-to-day basis) with the criteria for age-associated cognitive decline. These are essentially the same criteria used in several other studies (9, 10). To develop a better discriminating neuropsychological subtest, in addition to the K-MMSE test, we used the following tests to develop composite scores in areas of attention, visual memory, verbal memory, language, visuospatial function and executive function: 1) digit span, 2) visual span, 3) Korean Boston Naming Test (K-BNT) (19), 4) Rey-Osterrieth Complex Figure Test-copy (RCFT-copy), 5) Korean-California Verbal Learning Test (K-CVLT) (20), 6) Delayed Rey-Osterrieth Complex Figure Test (RCFT-delayed recall), and 7) Controlled Oral Word Association Test (COWAT) (21). These composite scores were defined as follows: attention score = sum of the raw scores from two subtests (digit span scores + visual span scores); language score = raw scores from the short form of the K-BNT; visuospatial score = raw scores from the the RCFT-copy; verbal memory score = raw scores from the K-CVLT; visual memory = raw scores from the RCFT-delayed recall; and executive function score = sum of the raw scores from two COWAT subtests (phonetic scores + category scores).
A clinical neuropsychologist conducted all neuropsychological testing. The testing was conducted in a quiet room with minimal visual and auditory distractions. Periods of rest were provided between testing to minimize the effects of fatigue.
Statistics were calculated and compared for the different sex of subjects and between neurocognitive groups using t-tests. Relationships between age and the three MR measures were analyzed using Pearson product-moment correlations. Correlation between the PBV, the T2LV, the PHMTR and the scores of neurocognitive tests were analyzed using Spearman correlations. Two-tailed p-values of less than .05 were considered statistically significant.
Age was significantly correlated with all three measurements (PBV r = -0.44, p = 0.016; T2LV 0.46, p = 0.012; PHMTR (r = -0.45, p = 0.013). Differences in age and years of education between the genders were not significant. No significant gender difference was observed for the PBV, the T2LV, and the PHMTR histogram (Table 1).
The K-MMSE scores of the subjects ranged from 15 to 30. The 12 subjects with low K-MMSE scores (< -2) did not differ significantly from the 23 subjects with normal K-MMSE scores (≥ -2), with respect to age or education. Subjects with low K-MMSE scores, however, had a significantly lower PBV (p = 0.02) and PHMTR (p = 0.005), and a significantly higher T2LV (p = 0.01) (Fig. 2) (Table 2). An inverse correlation was found between the PHMTR and T2LV (r = -0.74, p < 0.001), and also between the PBV and T2LV (r = -0.62, p < 0.001). A positive correlation was observed between the PHMTR and the PBV (r = 0.74, p < 0.001). Scores of the various neurocognitive tests correlated positively with the PHMTR and the PBV, and these scores were negatively correlated with the T2LV, in which we controlled for age (Table 3).
In the past few years, there has been growing interest for using a MTR histogram to investigate the in vivo dynamics of brain aging. To our knowledge, however, there have been only a few studies for the effect of normal brain aging on MTR histogram measurements in which MTR measurements were found to be sensitive for the detection of age-related brain tissue changes (22-24). These previously performed studies did not address some important issues, such as the correlation among measurements derived from conventional MR and MTR histograms or the correlation between cognitive performances and various MR measurements.
For various neurodegenerative diseases, the superiority of the MTR histogram has been mentioned to evaluate the patients' disease burden (14, 25-29). Since the MTR can reflect microscopic tissue changes as well as macroscopic changes, calculation of the MTR gives us additional information that is unavailable with conventional MR.
In the early stages of the aging process, brain changes are too subtle to be detected on conventional MR, as they start at a cellular level (loss of macromolecules; i.e., myelin). Therefore, the MTR values are decreased when the brain tissues undergo degeneration by the aging process, even though there is no change recorded on a conventional MRI. In our study, all three measurements were found to have correlation with age and with each other, which was similar to previous reports (23, 24). As shown in Table 2, all three measurements presented significant differences between groups divided by the K-MMSE scores, in spite of a lack of age difference between the two groups. However, the PHMTR showed a smaller p-value than the PBV and T2LV. In addition, all three measurements were also correlated significantly with the K-MMSE and various other neurocognitive performance scores when there were no age effects. Among the three methods, the PHMTR has shown a larger value of the correlation coefficient for the neurocognitive tests scores than those of the PBV and T2LV. One possible implication of this interesting result is that subjects with low neurocognitive performance scores may have a more accelerated brain aging process than normal elderly subjects, and those results were reflected with more sensitivity on the MTR histogram. It is generally accepted that the PHMTR is one of the most sensitive values of MTR histograms, and so it can simply and clearly depict the characteristics of the MTR histogram (14, 23, 25). For this reason, among the several possible values, we have adopted the PHMTR as the most useful parameter for MTR histograms. Van der Flier et al. (30) have reported similar results in their study with normal aging, mild cognitive impairment, and Alzheimer's disease. They have suggested the relative peak height (RPH) for the whole brain as one of the potent MTR values that were found to be lower than those of controls subjects with mild cognitive impairment and Alzheimer's disease. The RPH is really about the same value as the PHMTR except for the normalization reference (the total number of segmented parenchymal voxels rather than the total number of voxels in the entire tissue).
Some inconsistencies, however, exist between the measurements and individual neurocognitive tests. The PBV and T2LV, respectively, had two neurocognitive performance items that did not correlate with statistical significance, and the PHMTR had one. The attention test did not correlate with any of the measurements, and the reason for this inconsistency is uncertain. A major explanation for the differences observed across these studies could be due to the use of different tests and norms to evaluate neurocognitive performances.
The methodological problems in our study are mostly related to the small sample size. Although this study included subjects randomly selected from a community-dwelling population, the time-consuming and complicated tests could have discouraged some subjects to enroll in our study. Another possible limitation may be related to the instrument used to evaluate a cognitive domain. The K-MMSE is a quick and easy measure of cognitive function that has been widely used in clinical evaluation and research involving patients with dementia. It assesses orientation, attention, immediate and short-term recall, language, visuospatial function, and the ability to follow simple verbal and written commands. The K-MMSE provides a total score that places the individual on a scale of cognitive function. The K-MMSE, however, is unable to characterize the specific nature of cognitive decline in the whole brain. Unfortunately, the other neurocognitive tests used in this study are also not free from this limitation. So, the need for an adequately powered neuropsychological assessment method to reflect and validly delineate cognitive decline with age is apparent.
In conclusion, our findings of a correlation among the PBV, the T2LV, and the PHMTR suggest that MTR histograms and the PBV and T2LV can be used as a reliable method and valid statistical tools, respectively, for quantifying the total lesion burden in an aging brain.
Figures and Tables
Fig. 1
Segmented volume image of the intracranium (A), cerebrospinal fluid (B), and brain parenchyma (C) from proton density-weighted and T2-weighted images.
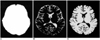
Fig. 2
Magnetization transfer ratio (MTR) histograms from normal subjects (dotted line) and cognitive impaired subjects (solid line). Normalized peak height of the MTR Histogram is lower for the cognitive impairment group compared with that of the normal groups.
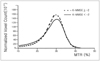
References
1. Salonen O, Autti T, Raininko R, Ylikoski A, Erkinjuntti T. MRI of the brain in neurologically healthy middle-aged and elderly individuals. Neuroradiology. 1997. 39:537–545.
2. DeCarli C, Murphy DGM, Trahn M, et al. The effect of white matter hyperintensity volume on brain structure, cognitive performance and cerebral metabolism of glucose in 51 healthy adults. Neurology. 1995. 45:2077–2084.
3. Smith CD, Snowdon DA, Wang H, Markesberry WR. White matter volumes and periventricular white matter hyperintensities in aging and dementia. Neurology. 2000. 54:838–842.
4. Rossor MN, Freeborough PA, Roques PK. Slowing the progression of Alzheimer's disease: Monitoring progression. Alzheimer Dis Assoc Disord. 1997. 11:Suppl. 5. S6–S9.
5. Golomb J, de Leon MJ, Kluger A, George AE, Tarshish C, Ferris SH. Hippocampal atrophy in normal aging. An association with recent memory impairment. Arch Neurol. 1993. 50:967–973.
6. Fein G, van Dyke C, Davenport L, et al. Preservation of normal cognitive functioning in elderly subjects with extensive white-matter lesions of long duration. Arch Gen Psychiatry. 1990. 47:220–223.
7. Filippi M, Grossman RI, Comi G. Magnetization transfer in multiple sclerosis. Neurology. 1999. 53:Suppl 3. S18–S22.
8. Brochet B, Dousset V. Pathological correlates of magnetization transfer imaging abnormalities in animal models and humans with multiple sclerosis. Neurology. 1999. 53:Suppl 3. S12–S17.
9. Kabani NJ, Sled JG, Chertkow H. Magnetization transfer ratio in mild cognitive impairment and dementia of Alzheimer's type. Neuroimage. 2002. 15:604–610.
10. Kabani NJ, Sled JG, Shuper A, Chertkow H. Regional magnetization transfer ratio changes in mild cognitive impairment. Magn Reson Med. 2002. 47:143–148.
11. Tanabe JL, Ezekiel F, Jagusta WJ, et al. Magnetization transfer ratio of white matter hyperintensities in subcortical ischemic vascular dementia. AJNR Am J Neuroradiol. 1999. 20:839–844.
12. Udupa JK, Samarasekera S. Fuzzy connectedness and object definition: Theory, algorithms and applications in image segmentation. Graph Models Image Process. 1996. 58:246–261.
13. Udupa J, Wei L, Samarasekera S, Miki Y, van Buchem MA, Grossman RI. Multiple sclerosis lesion quantification using fuzzy connectedness principles. IEEE Trans Med Imaging. 1997. 16:598–609.
14. Phillips MD, Grossman RI, Miki Y, et al. Comparison of T2 lesion volume and magnetization transfer ratio histogram analysis and of atrophy and measures of lesion burden in patients with multiple sclerosis. AJNR Am J Neuroradiol. 1998. 19:1055–1060.
15. Selim SZ, Ismail MA. K-means-type algorithms. IEEE Trans Pattern Anal Mach Intell. 1984. 6:81–87.
16. Van Buchem MA, Udupa JK, McGowan JC, et al. Global volumetric estimation of disease burden in multiple sclerosis based on magnetization transfer imaging. AJNR Am J Neuroradiol. 1997. 18:1287–1290.
17. Folstein MF, Folstein SE, Mc Hugh PR. Mini-mental state - a practical method grading the cognitive state of patients for the clinician. J Psychiatr Res. 1975. 12:189–198.
18. Kang YW, Na DL, Hahn SH. A validity study on the Korean mini-mental state examination (K-MMSE) in dementia patients. J Korean Neurol Assoc. 1997. 15:300–307.
19. Kang YW, Kim HJ, Na DL. Parallel short form for the Korean-Boston Naming Test (K-BNT). Korean J Clinical Psy. 2000. 18:144–150.
20. Kang YW, Kim JK. Korean-California Verbal Learning Test (K-CVLT): A normative study. Korean J Clinical Psy. 1997. 16:379–396.
21. Lee JH, Kang YW, Jin JH, Na DL, Park JS. A normative study of the Korean version of Controlled Oral Word Association Test (COWAT) in the elderly. Korean J clinical Psy. 2000. 19:385–392.
22. Hofman PAM, Kemerink GJ, Jolles J, Wilmonk JT. Quantitative analysis of magnetization transfer images of the brain: effect of closed head injury, age and sex on white matter. Magn Reson Med. 1999. 42:803–806.
23. Ge Y, Grossman RI, Babb JS, Rabin ML, Mannon LJ, Kolson DL. Age-related total gray matter and white matter changes in normal adult brain II: Quantitative magnetization transfer ratio histogram analysis. AJNR Am J Neuroradiol. 2002. 23:1334–1341.
24. Rovaris M, Iannucci G, Cercignani M, et al. Age-related changes in conventional, magnetization transfer, and diffusion-tensor MR imaging findings: study with whole-brain tissue histogram analysis. Radiology. 2003. 227:731–738.
25. Ge Y, Kolson DL, Babb JS, Mannon LJ, Grossman RI. Whole brain imaging of HIV-infected patients: quantitative analysis of magnetization transfer ratio histogram and fractional brain volume. AJNR Am J Neuroradiol. 2003. 24:82–87.
26. Ge Y, Grossman RI, Udupa JK, Babb JS, Mannon LJ, McGowan JC. Magnetization transfer ratio histogram analysis of normal-appearing gray matter and normal-appearing white matter in multiple sclerosis. J Comput Assist Tomogr. 2002. 26:62–68.
27. Hofman PA, Verhey FR, Wilmink JT, Rozendaal N, Jolles J. Brain lesions in patients visiting a memory clinic with postconcussional sequelae after mild to moderate brain injury. J Neuropsychiatry Clin Neurosci. 2002. 14:176–184.
28. Dehmeshki J, Van Buchem MA, Bosma GP, Huizinga TW, Tofts PS. Systemic lupus erythematosus: diagnostic application of magnetization transfer ratio histograms in patients with neuropsychiatric symptoms--initial results. Radiology. 2002. 222:722–728.
29. Dehmeshki J, Barker GJ, Tofts PS. Classification of disease subgroup and correlation with disease severity using magnetic resonance imaging whole-brain histograms: application to magnetization transfer ratios and multiple sclerosis. IEEE Trans Med Imaging. 2002. 21:320–331.
30. Van Der Flier WM, Van Den Heuvel DM, Weverling-Rijnsburger AW, et al. Magnetization transfer imaging in normal aging, mild cognitive impairment, and Alzheimer's disease. Ann Neurol. 2002. 52:62–67.