Abstract
Objective
To investigate the MR imaging and 1H-MR spectroscopic findings of acute fire-related post-traumatic stress disorder (PTSD).
Materials and Methods
Sixteen patients (M:F=10:6; mean age, 16 years) with fire-related PTSD underwent MR imaging and 1H-MR spectroscopy, and for control purposes, the procedures were repeated in eight age-matched normal volunteers. In all patients and controls, the regions of interest where data were acquired at MRS were the basal ganglia (BG), frontal periventricular white matter (FWM), and parietal periventricular white matter (PWM).
Results
In all patients with PTSD, MR images appeared normal. In contrast, MRS showed that in the BG, NAA/Cr ratios were significantly lower in patients than in volunteers. This decrease did not, however, show close correlation with the severity of the neuropsychiatric symptoms. In patients, neither NAA/Cr ratios in FWM nor PWM, nor Cho/Cr ratios in all three regions, were significantly different from those in the control group.
Following a serious fire in a public cafe in Korea in September 1999, more than 50 high-school students died and many survivors have suffered post-traumatic stress disorder (PTSD).
PTSD is an anxiety disorder that develops in persons who have experienced emotional or physical stress that for most individuals is extremely traumatic. Examples include combat experience, child sexual abuse, and natural and man-made disasters (1-3). Such individuals can re-experience their trauma through dreams or waking thoughts and have a variety of other emotional and cognitive symptoms. The findings of previous studies using MR imaging and PET in patients suffering from combat and noncombat-related PTSD suggest differences in hippocampal volume and increased blood flow in right-sided limbic structures, compared to age-matched controls (1-4).
The in-vivo 1H magnetic resonance spectroscopy (1H-MRS) technique has been developed as a noninvasive tool for biochemical assay. Localized 1H-MRS can detect alterations in brain metabolism in various diseases, and special attention has been paid to sub-clinical conditions such as early hepatic encephalopathy in which clinical symptoms and cerebral anatomic changes are not yet overt (5-12). Freeman et al. (13) recently reported 1H-MRS findings of significantly lower N-acetyl aspartate/creatine (NAA/Cr) ratios in the medial temporal lobe of patients with combat-related PTSD, and another recent study by de Bellis et al. (14) described similar low levels in the anterior cingulated gyrus of maltreated children and adolescents with PTSD.
To our knowledge, however, no previously published report has described the MR imaging and 1H-MR spectroscopic findings in patients with fire-related PTSD, especially during its acute stage. Moreover, no study has examined the relationship between these MR imaging and spectroscopic findings and the degree of neuropsychiatric symptoms in patients with PTSD. In this study, we evaluated the usefulness of combined 1H-MRS and MRI in patients with fire-related PTSD, and attempted to correlate the findings of MRS with the severity of the neuropsychiatric symptoms.
Sixteen consecutive patients [M:F=10:6; age, 15-17 (mean, 16) years] with fire-related PTSD underwent MR imaging and 1H-MR spectroscopy, and for the purpose of comparison, the procedures were repeated in eight healthy age-matched control subjects [M:F=6:2; age, 15-16 (mean, 15.7) years]. In neither patients nor controls did examinations involve the administration of any drug.
For the procedures involved, informed consent was obtained from all patients and the study protocol conformed to the ethical guidelines of the 1975 Declaration of Helsinki, a fact reflected in the a priori approval granted by our institutions' human research committees.
MR imaging and PROBE/SV examinations were performed with a 1.5-T whole-body GE MRI/MRS system (version 5.5; General Electric Medical Systems, Milwaukee, Wis., U.S.A.) equipped with actively shielded gradients. A quadrature head coil was used as a receiver and transducer. Before spectroscopy, spin-echo T1-weighted axial and sagittal images (TR/TE=484/8-9 msec, NEX=2, matrix size=256×192, slice thickness=7 mm, interslice gap=0 mm) and fast spin-echo T2-weighted axial images (TR/TE=4000/98 msec, NEX=2, matrix size=256×256, slice thickness=7 mm, interslice gap=0 mm) were obtained.
For all spectra, a STEAM (stimulated echo acquisition method) localization sequence incorporating a three-pulse chemical shift selective sequence to suppress the H2O signal was used, with the following acquisition parameters: TR=3.0 sec, TE=30 msec, mixing time=13.7 msec, spectral width=2500 Hz, size=2048 points, average=36, and two signals acquired. Voxel size was approximately 8 cm3 (2×2×2 cm).
In all patients and controls, the regions of interest for MRS data acquisition were the left basal ganglia (BG), right frontal periventricular white matter (FWM), and right parietal periventricular white matter (PWM) (Fig. 1). Voxel location was determined mainly through the use of axial MR images. To avoid tissue contamination from adjacent structures and to maintain consistent localization, all voxels were positioned by a single experienced neuroradiologist. Restricting the edge of voxels to positions approximately 5-10 mm from the inner table of the skull helped prevent contamination of the spectrum by strong signals originating from scalp fat, which can mask lactate resonance.
According to the consensus reached by two psychiatrists (J.S.L. and M.H.K.), all patients met the criteria for PTSD on the basis of the Korean version of the structured clinical interview for DSM-IV (SCID-RV) (15); the severity of neuropsychiatric symptoms was based on the results of the PTSD interview (PTSD-I) in DSM-III-R by Watson et al. (16). The PTSD-I was divided into five sections, A to E. The purpose of section A was to confirm significant trauma, while E related to the duration of symptoms. For section B-D (B: 're-experience'; C: 'avoidance'; D: 'hyperarousal'), scores of 1-7 were assigned, according to the severity of symptoms: 7 points for 'always, extreme', 1 point for 'no or never', and 4 points for 'frequently, moderate'. The cut-off point for symptoms was also recorded, and patients were assigned to one of two groups according to their total PTSD-I score.
Exposure to the fire was confirmed by official police records. Excluded from our study were individuals with current organic mental, bipolar, psychotic or neurological disorder; a history of major head trauma (defined as involving loss of consciousness for more than 10 minutes); or with alcohol or other substance dependence or abuse within the past year (determined according to SCID-RV). Subjects were free of psychotropic medications for at least 4 weeks prior to the onset of testing and for its duration.
Post-processing was carried out at a SUN SPARC 20 workstation using Spectral Analysis/General Electric (SA/GE) software incorporated with low frequency filtering of residual water signal removal, apodization by 0.5 Hz of exponential line broadening, zerofilling of 8k, Fourier transformation, and lorenzian to gaussian transformation, according to the method described by Kreis et al. (17). Metabolic peaks were fitted by lorenzian line shape at known frequencies of NAA at 2.02 ppm, Cr at 3.02 ppm, and Cho at 3.22 ppm. Results were expressed as relative ratios of metabolites to Cr.
We analyzed the MR imaging and spectroscopic findings in patients with PTSD, paying particular attention to spectral patterns different from those in the control group. In addition, the MRS findings were correlated with the severity of the neuropsychiatric symptoms.
Spectroscopic data were analyzed by Wilcoxon's rank sum test and Duncan's multiple range test to establish difference rankings between means at a statistically significant level, considered to be p<0.05.
PTSD symptoms were severe in nine patients and mild in seven (Table 1). In all 16, the interval between the fire and the time of MR examination was less than two months, and MR images appeared normal.
1H-MRS showed that in the BG, NAA/Cr ratios in patients (1.23±0.13) were significantly lower than in normal volunteers (1.46±0.11) (p<0.05), though there was sometimes overlap between these groups (Figs. 2A, B) (Table 2). In this same area, Cho/Cr ratios in patients and controls were 0.66±0.06 and 0.60±0.07, respectively, with no significant difference between the two groups. In FWM, NAA/Cr ratios in patients and controls were 1.52±0.22 and 1.55±0.16, respectively, and Cho/Cr ratios were 0.80±0.13 and 0.82±0.24, respectively (Figs. 2C, D), with no significant differences. In PWM, NAA/Cr ratios in patients and controls were 1.71±0.19 and 1.50±0.13, respectively, and Cho/Cr ratios were 0.76±0.14 and 0.71±0.17, respectively (Figs. 2E, F), again with no significant differences between the two groups (p>0.05) (Table 2).
In the basal ganglia, NAA/Cr ratios in patients with severe neuropsychiatric symptoms and those with mild symptoms were 1.22±0.13, and 1.27±0.13, respectively, with no significant differences between the groups (p>0.05). In addition, NAA/Cr ratios in FWM and PWM, as well as Cho/Cr ratios in all three regions, were not significantly different between patients with severe and mild neuropsychiatric symptoms (p>0.05) (Table 2).
In this study, the characteristic 1H-MR spectroscopic finding in patients with fire-related PTSD was a significantly decreased NAA/Cr ratio in the BG only. Other metabolite ratios showed no significant change.
PTSD is a unique psychological disease arising after extreme emotional and physical stress. Although a few previous MR imaging and PET studies in patients with PTSD have revealed hippocampal volume decrease or changes in blood flow in the limbic or paralimbic system, routinely obtained MR images were normal, as in other psychological diseases. This is because the anatomical or morphological changes which occur are very subtle and believed to be intimately related to changes in the biophysical or chemical metabolism (1-4). Whereas MR imaging is primarily concerned with gross morphological change accompanying the disease process, MRS takes advantage of the magnetic resonance phenomenon to provide access to living chemistry in situ. MRS is the only physical technique routinely used in clinical research that allows the assessment of in-vivo metabolism at the molecular level, which usually takes place prior to morphological change (5-12, 18-20). Accordingly, MRS might be more sensitive in detecting early cerebral change occurring in patients with PTSD, and can be employed in various neurological and psychological diseases where the findings of conventional imaging are normal, or only subtle change is demonstrated.
In this study, MR imaging revealed morphological change in neither patients nor controls, and the choice of region of interest (ROI) at MRS was thus very difficult. Because an ROI should involve an area where metabolic change might precede morphological change, this choice is, however, important. In a previous study by Freeman et al.(13) using 1H-MRS in patients with combat-related PTSD, the N-acetyl aspartate/creatine (NAA/Cr) ratio in the right medial temporal lobe was significantly lower than that on the left side, even in the same veterans. This was also the case when patients were compared with controls, though choline/creatine (Cho/Cr) ratios in the left medial temporal lobe were also lower. Instead of comparing right and left medial temporal lobes, as in their study, we located ROIs in the BG and periventricular white matter where metabolism might be active. In contrast to that previous study, which included patients with neuropsychiatric symptoms which had continued for years, ours involved those whose symptoms had existed for a relatively short period, after experiencing a serious fire at the same time and same place, and the identification of acute metabolic changes within the brain was thus facilitated. The voxel size of the ROIs employed in our study and in the earlier one was more than 8ml, too large to evaluate the hippocampus only, and contamination by adjacent brain parenchyma and CSF, or by the surrounding scalp fat, was inevitable. The BG periventricular white matter, on the other hand, are both large enough for proper MRS study without contamination by other structures. This is the second reason for the choice of ROIs in the basal ganglia and white matter.
After total parenteral nutrition and in patients with hepatocellular degeneration or manganese intoxication, the BG region is thought to be one of the areas most vulnerable to hypoxic brain damage and is also subject to deposition of various paramagnetic trace metals. Interestingly, our results show that in PTSD, this region appears to be one of the most metabolically active areas of the brain, though the anatomic changes occurring are not seen at MRI. Although the precise biochemical mechanism explaining the morphologic disturbance depicted by MR images in PTSD patients is still unknown, the results of this study support the hypothesis that ongoing or early metabolic change might occur in the BG of such patients. In as earlier 1H-MRS study involving patients with neuropsychiatric systemic lupus erythematosus, Lim at al. (21) drew similar conclusions. In our investigation, a further reason for choosing the white matter region rather than that of gray matter is the susceptibility of the former to small vascular lesions. We found, however, that in PTSD patients, the metabolites shown to be present in the periventricular white matter at 1H-MRS did not significantly change, and thus believe that to evaluate the early biochemical changes accompanying fire-related PTSD, the most suitable site for 1H-MRS is the basal ganglia.
In a research-based study, the ideal condition is homogeneity. In combat-related and sexual abuse-related PTSD, the causes, types, intensities, and duration of psychic traumas are, however, individually different, and in such patients it is thus difficult to categorize the type and degree of injury. In this study, on the other hand, conditions such as cause, stage, and age were the same or similar, and for that reason our results make a meaningful contribution to research into PTSD as well as to other psychological diseases.
Our findings showed that in patients with fire-related PTSD, NAA levels in the BG diminished. This decrease, known to be a neuronal marker, indicates not only a loss of neurons or neuronal activity but also neuronal dysfunction or impairment as a result of myelin breakdown. Decreased NAA/Cr ratios in the BG may be indirect evidence of extensive small vessel injury in basal ganglia seen at MRI as apparently normal (21, 22). These spectroscopic findings suggest that the clinical presentation of the neuropsychiatric symptoms indicates severe cerebral insult resulting in significant neuronal injury.
Because a serious fire can cause hypoxic or toxic brain insults, our MRS findings might reflect such insult rather than the existence of typical PTSD. We believe, however, that this is unlikely: no abnormal brain MR imaging findings, observed in patients with hypoxic or toxic insult, were apparent, and among our patients, the neuropsychiatric symptoms typically occurring in PTSD cases were present.
A possible weakness inherent in our study is that its MRS findings did not correlate closely with the severity of the neuropsychiatric symptoms of the patients involved. This might be partly attributed to the fact that the evaluation and grading of such symptom was on the basis of the PTSD interview alone, reliance being placed mainly on the subjective description these patients provided. Conse-quently, the grading of the symptoms is not objective. In addition, our study included only a small number of PTSD patients, and for these reasons, further series, involving MRI and 1H-MRS studies of more such patients, are essential.
In conclusion, decreased NAA/Cr ratios in the BG, seen at 1H-MRS, might be an early sign of abnormality in acute fire-related PTSD.
Figures and Tables
Fig. 1
Transverse T2-weighted fast spin-echo MR spectrographic images (TR/TE: 4,000/98) depict examples of voxel placement (box) in (A) the basal ganglia, (B) frontal periventricular white matter, and (C) parietal periventricular white matter in patients with PTSD.
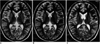
Fig. 2
1H-MR spectra show NAA, Cr, and Cho levels in the basal ganglia (A, B), frontal white matter (C, D), and parietal white matter (E, F) of normal controls (A, C, E) and PTSD patients with severe neuropsychiatric symptoms (B, D, F). In (A), NAA is present at 2.02 ppm, Cr at 3.02 ppm, and Cho at 3.22 ppm. The NAA peak is lower in (B) than in (A).
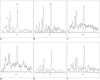
References
1. Bremner D, Randall P, Scott TM, et al. MRI-based measurement of hippocampal volume in patients with combat-related post-traumatic stress disorder. Am J Psychiatry. 1995. 152:973–981.
2. Gurvits TV, Shenton ME, Hokama H, et al. Magnetic resonance imaging study of hippocampal volume in chronic, combat-related posttraumatic stress disorder. Biol Psychiatry. 1996. 40:1091–1099.
3. Bremner D, Randall P, Vermetten E, et al. Magnetic resonance imaging-based measurement of hippocampal volume in post-traumatic stress disorder related to childhood physical and sexual abuse - a preliminary report. Biol Psychiatry. 1997. 41:23–32.
4. Rauch SL, van der Kolk BA, Fisler RE, et al. A symptom provocation study of posttraumatic stress disorder using positron emission tomography and script-driven imagery. Arch Gen Psychiatry. 1996. 53:380–387.
5. Lee JH, Seo DW, Lee YS, et al. Proton magnetic resonance spectroscopy (1H-MRS) findings for the brain in patients with liver cirrhosis reflect the hepatic functional reserve. Am J Gastroenterol. 1999. 94:1–10.
6. Ross BD, Jacobson S, Villamil F, et al. Subclinical hepatic encephalopathy: proton MR spectroscopic abnormalities. Radiology. 1994. 193:457–463.
7. Lee CH, Lee JH, Kim JJ, et al. Cerebral metabolic abnormalities in congestive heart failure detected by proton magnetic resonance spectroscopy. J Am Coll Cardiol. 1999. 33:1196–1202.
8. Chang KH, Kim HD, Park SW, et al. Usefulness of single-voxel proton MR spectroscopy in the evaluation of hippocampal sclerosis. Korean J Radiol. 2000. 1:25–32.
9. Davidson J, Krishnan K, Charles H, et al. Magnetic resonance spectroscopy in social phobia: preliminary findings. J Clin Psychiatry. 1993. 54:Suppl. 19–25.
10. Choi CG, Lee HK, Yoon JH. Localized proton MR spectroscopic evaluation of nonketotic hyperglycemia in an infant. Korean J Radiol. 2001. 2:239–242.
11. Baik HM, Choe BY, Lee HK, Suh TS, Son BC, Lee JM. Metabolic alterations in Parkinson's disease after thalamotomy, as revealed by 1H-MR spectroscopy. Korean J Radiol. 2002. 3:180–188.
12. Nasrallah HA, Skinner TE, Schmalbrock P, Pobitaille PM. Proton magnetic resonance spectroscopy of the hippocampal formation in schizophrenia: a pilot study. Br J Psychiatry. 1994. 165:481–485.
13. Freeman TW, Caldwell D, Karson CN, Komoroski RA. In-vivo proton magnetic resonance spectroscopy of the medial temporal lobes of subjects with combat-related posttraumatic stress disorder. Magn Reson Med. 1998. 40:66–71.
14. de Bellis MD, Keshavan MS, Spencer S, Hall J. N-acetyl aspartate concentration in the anterior cingulated of maltreated children and adolescents with PTSD. Am J Psychiatry. 2000. 157:1175–1177.
15. Hahn OS, Ahn JH, Song SH, et al. Development of Korean version of structured clinical interview schedule for DSM-IV axis I disorder: inter-rater reliability. J Korean Neuropsychiatr Assoc. 2000. 39:362–372.
16. Watson CG, Juba MP, Manifold V, Kucala T, Anderson PE. The PTSD interview: rationale, description, reliability and concurrent validity of a DSM-III-based technique. J Clin Psychol. 1991. 47:179–188.
17. Kreis R, Ernst T, Ross BD. Development of the human brain: in-vivo quantification of metabolic and water content with proton magnetic resonance spectroscopy. Magn Reson Med. 1993. 30:424–437.
18. Marshall I, Wardlaw J, Cannon J, Slattery J, Sellar RJ. Reproducibility of metabolite peak areas in 1H-MRS of brain. Magn Reson Imaging. 1996. 14:281–292.
19. Strauss WL, Tsuruda JS, Richards TL. Partial volume effects in volume-localized phased-array proton spectroscopy of the temporal lobe. J Magn Reson Imaging. 1995. 4:433–436.
20. Passe TJ, Charles HC, Rajagopalan P, Krishnan KR. Nuclear magnetic resonance spectroscopy: a review of neuropsychiatric applications. Prog Neuropsychopharmacol Biol Psychiatry. 1995. 19:541–563.
21. Lim MK, Suh CH, Kim HJ, et al. Systemic lupus erythematosus: brain MR imaging and single-voxel hydrogen 1-MR spectroscopy. Radiology. 2000. 217:43–49.
22. De Stefano N, Matthews PM, Arnold DL. Reversible decreases in N-acetylaspartate after acute brain injury. Magn Reson Med. 1995. 34:721–727.