Abstract
Objective
To evaluate, using localized proton magnetic resonance spectroscopy (1H-MRS), the cerebral metabolic change apparent after revascularization surgery in patients with moyamoya disease.
Materials and Methods
Sixteen children with moyamoya disease and eight age-matched normal controls underwent MR imaging, MR angiography, conventional angiography, and 99mTc-ECD SPECT. Frontal white matter and the basal ganglia of both hemispheres were subjected to localized 1H-MRS, and after revascularization surgery, four patients underwent follow-up 1H-MRS.
Results
Decreased NAA/Cr ratios (1.35±0.14 in patients vs. 1.55±0.24 in controls) and Cho/Cr ratios (0.96±0.13 in patients vs. 1.10±0.11 in controls) were observed in frontal white matter. After revascularization surgery, NAA/Cr and Cho/Cr ratios in this region increased. In the basal ganglia, there is no abnormal metabolic ratios.
Moyamoya disease involves slowly progressive stenosis in bilateral proximal intracranial vessels, leading to reduced cerebral perfusion (1, 2). Symptoms such as headaches and unilateral or bilateral transient ischemic attacks develop in young children, especially during hyperventilation (2, 3). Although the exact cause of the disease is still unknown and the specifics of its natural course are a matter of controversy, its outcomes range from reversible ischemia to cerebral infarction associated with permanent neurological deficits and intellectual impairment (4, 5). Early diagnosis followed by accurate treatment is, therefore, critically important, especially for young children.
Revascularization surgery in pediatric patients with moyamoya disease has been shown to be an effective treatment method (6, 7). At present, cerebral or MR angiography and MR imaging are modalities by means of which the disease can be diagnosed with confidence; in addition, single photon emission tomography (SPECT) provides confirmation of the diagnosis and is used to evaluate the cerebral perfusion reserve.
Localized proton MR spectroscopy (1H-MRS) has recently been used to detect the metabolic changes occurring in the brain in numerous diseases (8). Its noninvasive nature has increasingly attracted the attention of pediatricians, who see it as a promising application (9, 10). Although localized 1H-MRS has been used in patients with internal carotid artery flow lesions (11), it has never been employed in those with moyamoya disease. In regions without infarction but with severely reduced internal carotid flow, 1H-MRS has demonstrated decreased NAA/Choline ratios and a high incidence of cerebral lactate (11), and it can be inferred that in the brains of patients with moyamoya disease, NAA and/or Choline levels are lower than normal. In this study we used localized 1H-MRS to measure changes in the cerebral metabolite levels found in moyamoya disease before and after revascularization surgery. Our hypothesis is that localized 1H MRS can be used to preoperatively evaluate cerebral metabolic abnormalities, and to monitor these postoperatively.
Our study group consisted of 16 consecutive children aged 3-13 (mean, 7.6) years in whom moyamoya disease was diagnosed between March 1998 and December 1999, and eight age-matched normal controls aged 4-11 (mean, 8) years. Diagnosis was based on the findings of conventional angiography (n=12), MR imaging (n=16), MR angiography (n=7), SPECT (n=16), and clinical symptoms. Nine children showed unilateral symptoms of transient ischemic attack and six had bilateral symptoms; at the time of initial diagnosis, these included TIA (n=15), headache (n=3), seizure (n=2) and/or aphasia (n=2). All patients underwent initial 1H-MRS, and four underwent follow-up 1H-MRS 6-19 months after surgery (encephalo-duro-arterio-myo-synangiosis; EDAMS). In three patients (numbers 1, 4, 11), revascularization surgery was performed in both hemispheres, and in one (number 7), in the left hemisphere only. These four patients underwent follow-up SPECT 6-22 months after surgery.
All patients and normal controls gave their informed consent to 1H-MRS. The study protocol conformed to the ethical guidelines of the 1975 Declaration of Helsinki, as reflected in its approval by our institution's Human Research Committee.
For MR imaging and angiography, a 1.5T scanner (Magnetom Vision, Siemens, Erlangen, Germany) was used, and in all patients, T1-weighted (TR/TE:600/11-20) and T2-weighted (TR/TE:3500/102) spin-echo images, as well as Gd-DTPA (Magnevist, Schering, Germany)-enhanced T1-weighted images, were acquired. In seven patients, MR angiography with a three-dimensional time-of-flight (3D-TOF) sequence was performed.
Twelve patients underwent transfemoral cerebral angiography using an Integris BN 3000 biplane (Philips Medical System, Eindhoven, Netherlands), after injection of the bilateral internal and external carotid and unilateral vertebral artery. Grading of the involved vessels was based on Suzuki's classification (3).
Using a Triad XLT20 (Trionix, Twinsburg, U.S.A.) equipped with a LEUR collimator interfaced to a Sun Sparc workstation, serial dynamic SPECT scans (1min×50 frames) were obtained in 16 patients. Two doses of 99mTc-ECD (ethyl cysteinate dimmer) were administered, one at the onset of scanning (20mCi) and one at 30 mins (20mCi). Diamox (1g) was injected intravenously at 10 mins. Two nuclear physicians visually assessed the perfusion status, comparing it with that of cerebellar perfusion.
Using a 1.5T system (Signa; GE Medical Systems, Milwaukee, U.S.A.) equipped with shielded gradients, localized in-vivo 1H-MRS was performed 1-2 weeks after T2-weighted MRI was used to localize and evaluate any changes in brain parenchymal signal intensity, compared to previous MRI findings. Image-guided STEAM spectra were obtained from frontal white matter at the border zone between anterior and middle cerebral artery territory and the basal ganglia in both hemispheres, avoiding areas of old infarcts or abnormal signal intensities (Figs. 1A, 2A). The spectroscopic acquisition parameters were as follows: TR=3.0 sec/ TE=30 msec, and NS=36 AVG with PROton Brain Exam (PROBE) (GE Medical Systems). Voxel volumes were 7-9 ml, and a 3-pulse CHESS (CHEmical Shift Selective) sequence was used for suppression of the H2O signal.
All raw data were transferred to a Sun Sparc-10 work-station (SUN Computer Inc., Sunnyvale, U.S.A.), and processed using Spectral Analysis/General Electric (SA/GE) software (GE Medical Systems) incorporating low frequency filtering of residual water signal, apodization by 0.5 Hz of exponential line broadening, zerofilling of 8k, Fourier transformation, and lorenzian to gaussian transformation according to the scheme described by Kreis et al. (12). Metabolic peaks were fitted by the lorenzian line shape at known frequencies of N-acetylaspartate (NAA) at 2.02 ppm, creatine (Cr) at 3.03 ppm, choline and choline-containing compounds (Cho) at 3.22 ppm, and myo-Inositol (mI) at 3.56 ppm. The values of the [NAA/Cr], [Cho/Cr], and [mI/Cr] ratios were calculated.
The spectroscopic data were expressed as mean±standard error. Mean (right and left) metabolite ratios between patients and normal controls, and between symptom-related and other hemispheres were compared using the Mann-Whitney U test. In four patients, changes in metabolite ratios after surgery were assessed by Wilcoxon's signed rank test. For statistical analyses, SPSS Windows (SPSS for Windows, SPSS Inc., Chicago, U.S.A.) was used.
Tables 1 and 2 summarize the clinical data and findings at MR imaging, angiography, SPECT, and 1H-MRS (Figs. 1, 2).
Localized 1H-MRS revealed decreased NAA/Cr and Cho/Cr ratios(Fig. 1F) in 16 patients, in whom mean NAA/Cr, Cho/Cr, and mI/Cr ratios were 1.35±0.14, 0.96±0.13, and 0.68±0.12, respectively, in frontal white matter, compared with 1.55±0.24, 1.10±0.11, and 0.64±0.08 in normal controls (p<0.05). In patients, mean NAA/Cr, Cho/Cr, and mI/Cr ratios were 1.13±0.14, 0.73±0.07, and 0.44±0.07, respectively, in both basal ganglia, while the corresponding figures for normal controls were 1.21±0.14, 0.77±0.10, and 0.45±0.07. Reduced NAA/Cr and Cho/Cr ratios were detected in 13 patients, while in three, the Cho/Cr ratio had increased. In two of these three, the NAA/Cr ratio had decreased, and in one it was normal.
In the symptomatic hemisphere, NAA/Cr, Cho/Cr and mI/Cr ratios were 1.35±0.28, 0.89±0.26, and 0.67±0.18, respectively, while on the asymptomatic side, the respective readings were 1.33±0.28, 0.93±0.24, and 0.68±0.22. The differences between the two hemispheres were not statistically significant.
Four patients underwent follow-up 1H-MRS 6 to 19 months after revascularization surgery: their NAA/Cr ratios had increased (p < 0.05), slightly overshooting Cho/Cr ratios in the hemispheres (Fig. 2D) with improved symptoms of transient ischemic attack (Table 3). One of the four patients (number 7, Table 3) still exhibited TIA symptoms on the side not operated on, and the NAA/Cr ratio in frontal white matter remained low. Cho/Cr ratios were slightly overshot in all four patients, but were still within the upper normal limit. Basal and diamox stress SPECT showed increased perfusion in both hemispheres of all four patients, as compared with the preoperative results.
N-acetylaspartate is known to be a neuronal marker, and a decrease in its level indicates a loss of neurons or neuronal activity owing to myelin breakdown, as occurs in brain infarct, tumor, and radiation injury (13). Choline appears to contain contributions from phosphorylcholine and glycerophosphorylcholine, which are, respectively, a precursor to cell membrane and a product of its breakdown. An increased choline signal is therefore thought to be an indicator of active demyelination (14).
Duijin et al. (15) and Lanfermann et al. (16) observed decreased choline levels in chronic, but not acute or subacute, infarctions. In their study, they also observed that choline and creatine decreased to a lesser extent than N-acetylaspartate in the peripheral region of the infarcted lesion. This may explain the loss of neuronal function while glial cellular structure in the peripheral region is still intact. In our patients, the abnormal NAA/Cr and Cho/Cr values found in frontal white matter may also indicate a loss of neuronal function, a hypothesis supported by the normal MRI findings and absence of a lactate peak. A lactate signal is an end product of anaerobic glucose metabolism, suggesting that this ischemic process may be more likely in the chronic than in the acute state.
Children with moyamoya disease suffer from headache and unilateral or bilateral transient ischemic attack (2, 3), even if they do not have persistent neurological deficits. Decreased cerebral blood flow and cerebral perfusion reserve have been demonstrated in patients with the disease, and these lead to ischemic neurologic symptoms (17-25). CT and MRI are useful for the detection of infarction, but their ability to detect perfusion disturbances is limited. SPECT demonstrates the degree and region of hemodynamic compromise and is commonly used to determine suitable surgical candidates. It cannot, however, disclose metabolic change as a consequence of chronic cerebral ischemia. As shown in this study, 1H-MRS, which is noninvasive, can provide information on deranged metabolites under conditions of chronic cerebral hypoperfusion.
In this study, the findings of 1H-MRS appear to suggest a slightly greater decrease in Cho/Cr ratios in symptom-related hemispheres, but the differences were not statistically significant. Further investigation involving a large series with asymmetric involvement of both hemispheres would help clarity this issue.
In three of 16 patients, Cho/Cr ratios had increased and NAA/Cr ratios had decreased or were normal. Rutgers et al. (26) described the cerebral metabolic changes observed at 1H-MRS in patients with symptomatic occlusion of the internal carotid artery. During the period of acute infarction, the NAA/Cr ratio was significantly lower than in control subjects in the hemisphere ipsilateral to ICA occlusion, and the Cho/Cr ratio was significantly higher. Subsequently, the Cho/Cr ratio decreased significantly and returned to the control value. These findings suggest that increased Cho/Cr ratios may indicate an acute process.
Various revascularization procedures such as EDAMS have served to increase the blood supply to ischemic areas of the brain, and in this study, symptoms of transient ischemic attack disappeared or improved in three patients after revascularization surgery, and NAA/Cr ratios increased. Increased N-acetylaspartate levels have been recognized only in Canavan's disease and in infants during normal development (27, 28), and could account for changes in N-acetylaspartate metabolism and/or neuronal activity (29). In this study, another finding after revascularization surgery was that Cho/Cr ratios had slightly overshot. It has been reported that the choline levels increased to above the normal limit after liver transplantation and that this was due to cyclosporine related encephalopathy or neurotoxicity (8). The patients in our study did not, however, undergo immunosuppressive treatment, and it can therefore be inferred that increased perfusion after revascularization surgery may transiently influence the level of choline. Further study and long-term follow-up of choline levels are therefore needed.
It has been shown that in moyamoya disease, vascular reserve in the cerebral cortex of anterior and middle cerebral artery territory is significantly lower than in posterior cerebral artery territory and the central region around the basal ganglia and thalami (30-32). The results of our study indicated that in both basal ganglia, metabolite levels were normal, though it may be speculated that metabolic change might occur there at a later stage of the disease, when basal collaterals disappear.
In conclusion, localized 1H-MRS in patients with moyamoya disease demonstrated bilaterally decreased N-acetylaspartate and choline ratios. 1H-MRS effectively measures the metabolic changes occurring in the brain in moyamoya disease, and its non-invasive nature lends itself to the preoperative evaluation of metabolic abnormality and its postoperative monitoring.
References
1. Kudoh T. Juvenile occlusion of the circle of Willis. Clin Neurol. 1965; 5:607.
2. Kuwabara Y, Ichiya Y, Otsuka M, et al. Cerebral hemodynamic change in the child and the adult with moyamoya disease. Stroke. 1990; 21:272–277. PMID: 2305403.


3. Suzuki J, Takaku A. Cerebrovascular "moyamoya" disease: disease showing abnormal net-like vessels in base of brain. Arch Neurol. 1969; 20:288–299. PMID: 5775283.
4. Halbach VV, Barkovich AJ. Anomalies of cerebral vasculature: Pediatric neuroimaging. 1995. 2nd ed. New York: Raven Press;p. 619–653.
5. Kinugasa K, Mandai S, Kamata I, Sugiu K, Ohmoto T. Surgical treatment of moyamoya disease: operative technique for encephalo-duro-arterio-myo-synangiosis, its follow-up, clinical results, and angiograms. Neurosurgery. 1993; 32:527–531. PMID: 8474642.
6. Ishikawa T, Houkin K, Kamiyama H, Abe H. Effects of surgical revascularization on outcome of patients with pediatric moyamoya disease. Stroke. 1997; 28:1170–1173. PMID: 9183345.


7. Matsushima Y, Fukai N, Tanaka K, et al. A new surgical treatment of moyamoya disease in children: a preliminary report. Surg Neurol. 1987; 15:313–320. PMID: 7245020.


8. Ross BD, Michaelis T. Clinical applications of magnetic resonance spectroscopy. Magn Reson Q. 1994; 10:191–247. PMID: 7873353.
9. Kimura H, Fujii Y, Itoh S, et al. Metabolic alterations in neonate and infant brain during development : evaluation with proton MR spectroscopy. Radiology. 1995; 194:483–489. PMID: 7529934.
10. Lee JH, Arcinue E, Ross BD. Organic osmolytes in the brain of an infant with hypernatremia. N Engl J Med. 1994; 331:439–442. PMID: 8035840.


11. Van der Grond J, Balm R, Kappelle L, Eikelboom BC, Mali WP. Cerebral metabolism of patients with stenosis or occlusion of the internal carotid artery: A 1H-MR spectroscopic imaging study. Stroke. 1995; 26:822–828. PMID: 7740574.
12. Kreis R, Ross BD, Farrow NA, Ackerman Z. Metabolic disorders of the brain in chronic hepatic encephalopathy detected with H-1 MR spectroscopy. Radiology. 1992; 182:19–27. PMID: 1345760.


13. Bizzi A, Movsas B, Tedeschi G, et al. Response of non-Hodgkin lymphoma to radiation therapy: early and long-term assessment with H-1 MR spectroscopic imaging. Radiology. 1995; 194:271–276. PMID: 7997566.


14. Rajanayagam V, Grad J, Krivit W, et al. Proton MR spectroscopy of childhood adrenoleukodystrophy. AJNR Am J Neuroradiol. 1996; 17:1013–1024. PMID: 8791909.
15. Duijin JH, Matson GB, Maudsley AA, Hugg JW, Weiner MW. Human brain infarction: Proton MR spectroscopy. Radiology. 1992; 183:711–718. PMID: 1584925.


16. Lanfermann H, Kugel H, Heindel W, Herholz K, Heiss W-D, Lackner K. Metabolic changes in acute and subacute cerebral infarctions: findings at proton MR spectroscopic imaging. Radiology. 1995; 196:203–210. PMID: 7784568.


17. Van der Grond J, Ramos LMP, Eikelboom BC, Mali WP. Cerebral metabolic differences between the severe and critical hypoperfused brain. Neurology. 1996; 47:399–404. PMID: 8757011.


18. Takemichi TK, Prohovnik I, Mohr JP, Correll JW, Quest DO, Jarvis L. Reduced hypercapnic vasoreactivity in moyamoya disease. Neurology. 1988; 38:1575–1581. PMID: 3419602.


19. Taki W, Yonekawa Y, Kobayashi A, et al. Cerebral circulation and metabolism in adult's moyamoya disease: PET study. Acta Neurochir (Wien). 1989; 100:150–154. PMID: 2589122.
20. Ogawa A, Yoshimoto T, Suzuki J, Sakurai Y. Cerebral blood flow in moyamoya disease, part 1: correlation with age and regional distribution. Acta Neurochir (Wien). 1990; 105:30–34. PMID: 2239376.
21. Ogawa A, Nakamura N, Yoshimoto T, Suzuki J. Cerebral blood flow in moyamoya disease, part 2: autoregulation and CO2 response. Acta Neurochir (Wien). 1990; 105:107–111. PMID: 2125802.
22. Sato H, Sato N, Tamuki N, Matsumoto S. Chronic low-perfusion state in children with moyamoya disease following revascularization. Childs Nerv Syst. 1990; 6:166–171. PMID: 2357714.


23. Ohashi K, Fernandez-Ulloa M, Hall LC. SPECT, magnetic resonance and angiographic features in a moyamoya patient before and after external-to-internal carotid artery bypass. J Nucl Med. 1992; 33:1692–1695. PMID: 1517845.
24. Inoue Y, Momose T, Machida K, Honda N, Tsutsumi K. Cerebral vasodilatory capacity mapping using technetium-99m-DTPA-HAS SPECT and acetazolamide in moyamoya disease. J Nucl Med. 1993; 34:1984–1986. PMID: 8229245.
25. Rutgers DR, Klijn CJ, Kappelle LJ, van der Grond J. Cerebral metabolic changes in patients with symptomatic occlusion of the internal carotid artery: a longitudinal 1H magnetic resonance spectroscopy study. J Magn Reson Imaging. 2000; 11:279–286. PMID: 10739559.
26. Janson C, McPhee S, Bilaniuk L, et al. Clinical protocol gene therapy of Canavan disease: AAV-2 vector for neurosurgical delivery of aspartoacylase gene (ASPA) to the human brain. Hum Gene Ther. 2002; 13:1391–1412. PMID: 12162821.
27. Hashimoto T, Tayama M, Miyazaki M, et al. Developmental brain changes investigated with proton magnetic resonance spectroscopy. Dev Med Child Neurol. 1995; 37:398–405. PMID: 7768339.


28. Shimizu H, Shirane R, Fujiware S, Takahashi A, Yoshimoto T. Proton magnetic resonance spectroscopy in children with moyamoya disease. Clin Neurol Neurosurg. 1997; 99:S64–S67. PMID: 9409409.


29. Touho H, Karasawa J, Ohnishi H. Preoperative and postoperative evaluation of cerebral perfusion and vasodilatory capacity with 99mTc-HMPAO SPECT and acetazolamide in childhood moyamoya disease. Stroke. 1996; 27:282–289. PMID: 8571424.
30. Hoshi H, Ohnishi T, Jinnouchi S, et al. Cerebral blood flow study in patients with moyamoya disease evaluated by IMP SPECT. J Nucl Med. 1994; 35:44–50. PMID: 8271059.
31. Miller JH, Khonsary A, Raffel C. The scintigraphic appearance of childhood moyamoya disease on cerebral perfusion imaging. Pediatr Radiol. 1996; 26:833–883. PMID: 8929293.


Fig. 1
MRI, basal and diamox stress SPECT, cerebral angiography and 1H-MR spectra obtained in patient No. 5.
A. T2-weighted axial image shows a voxel location for 1H-MRS and no abnormal signal intensity in the brain.
B. Basal SPECT (axial view) discloses normal uptake in both hemispheres.
C. Diamox stress SPECT (axial view) reveals decreased uptake in both hemispheres.
D. Arterial-phase angiogram of the left common carotid artery (frontal view) shows marked stenosis of the distal internal carotid and anterior and middle cerebral arteries, with tortuous and dilated medial and lateral lenticulostriate vessels (Suzuki stage II).
E. Arterial-phase angiogram of the right common carotid artery (frontal view) demonstrates findings similar to those of the left side (Suzuki stage II).
F. Representative 1H-MR spectra of frontal white matter (both sides) show lower NAA/Cr [Lt(b): 1.21, Rt(c): 1.34], Cho/Cr [Lt(b): 0.71, Rt (c): 0.77] ratios than in an age-matched control (a).
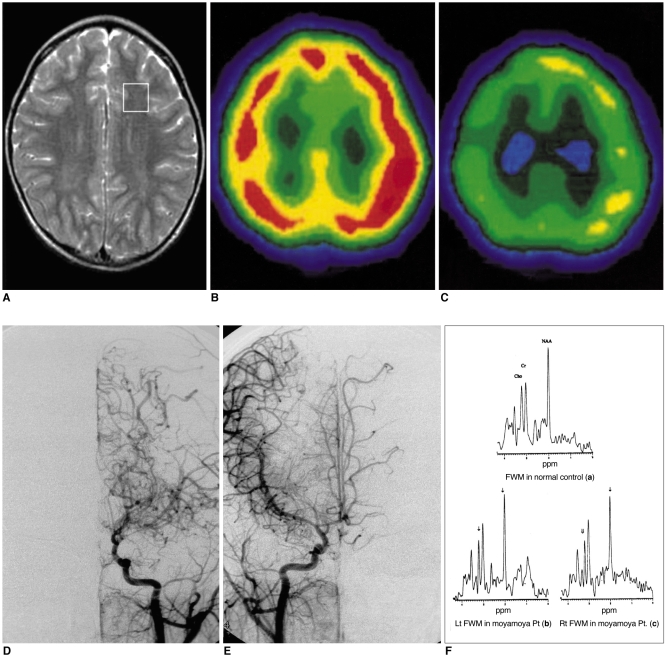
Fig. 2
MRI, cerebral angiography and 1H-MR spectra obtained in patient No. 1.
A. T2-weighted axial image shows no abnormal signal intensity in the brain.
B. Arterial-phase angiogram of the left common carotid artery (frontal view) reveals narrowing of the carotid fork (Suzuki stage I).
C. Angiogram of the right common carotid artery (frontal view) shows the beginning of basal moyamoya vessels, and narrowing of the supraclinoid internal carotid and anterior and middle cerebral arteries (Suzuki stage II).
D. 1H-MR spectra of frontal white matter (both sides) obtained after revascularization surgery disclose an increased NAA/Cr ratio [Lt(b): 1.56, Rt(d): 1.41] and a slightly increased upper normal range of Cho/Cr [Lt(b): 1.23, Rt(d): 1.20] as compared with preoperative NAA/Cr [Lt(a): 1.24, Rt(c): 1.14] and Cho/Cr [Lt(a): 0.93, Rt(c): 1.02] levels.
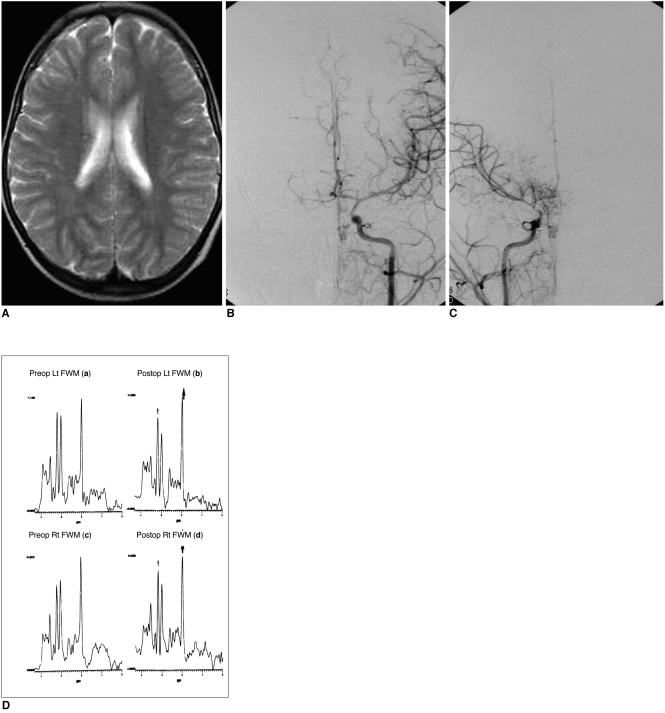
Table 1
Clinical History and Findings of MRI, Angiography and SPECT in Patients with Moyamoya Disease
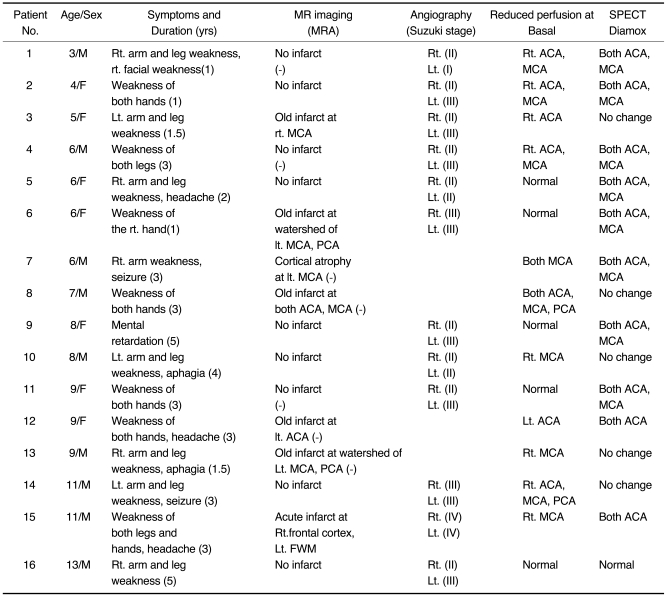