Abstract
Objective
To determine whether hypertonic saline (HS, 36% NaCl) injection prior to or during radiofrequency ablation (RFA) can increase the extent of thermally mediated coagulation in in-vivo rabbit liver tissue, and also to establish the ideal injection time in relation to RFA in order to maximize its effect on the extent of radiofrequency (RF)-induced coagulation.
Materials and Methods
In 26 rabbits, 43 RFA lesions were produced using a 17-gauge internally cooled electrode with a 1-cm active tip under ultrasound (US) guidance. Rabbits were assigned to one of three groups: Group A: RFA alone (n=8); Group B: RFA after the instillation of 1 mL HS (n=8); Group C: RFA after and during the instillation of 0.5 mL HS (n=10). RF energy (30 W) was applied for 3 minutes, and changes occurring in tissue impedance, current, power output, and the temperature of the electrode tip were automatically measured. After RFA, contrast-enhanced spiral CT was performed, and in each group the maximum diameters of the thermal lesions in gross specimens were compared. Technical success and the complications arising were evaluated by CT and on the basis of autopsy findings.
Results
All procedures were technically successful. There were six procedure-related complications (6/26; 23%), including five localized perihepatic hematomas and one thermal injury to the stomach. With instillation of HS in group B rabbits, markedly decreased tissue impedance (73Ω ± 5) and increased current (704 mA ± 41) were noted, compared to RF ablation without saline infusion (116.3Ω ± 13, 308 mA ± 80). With instillation of the solution before RFA (group B), coagulation necrosis was greater (14.9 mm ± 3.8) than in rabbits not injected (group A: 11.5 mm ± 2.4; Group A vs. B: p < .05) and in those injected before and during RFA (group C: 12.5 mm ± 3.1; Group B vs. C: p > .05).
Conclusion
RFA using HS instillation can increase the volume of RFA-induced necrosis of the liver with a single application, thereby simplifying and accelerating the treatment of larger lesions. In addition, HS instillation before RFA more effectively achieves coagulation necrosis than HS instillation before and during RFA.
Recent studies have demonstrated that radiofrequency ablation (RFA) is safe and effective for the treatment of focal metastatic and primary liver tumors (1-6). It is, in addition, gaining increased attention as an imaging-guided tumor ablation technique for the treatment of focal malignant tumors in other organs, including the kidney (7), bone (8), breast (9), and brain (10). Whereas promising initial results have been achieved for liver tumors (1-3), several clinical studies have reported inadequate treatment of focal liver lesions greater than 3 cm in diameter and also relatively high rates of local tumor progression (11, 12). It has been suggested that the latter is due to gross underablation of the tumors and the failure to create an adequate tumor-free margin (13), and in order to create an adequate such margin in most clinically relevant tumors (3-5 cm in diameter), it is, therefore, necessary to induce greater thermal necrosis with one RFA procedure.
Several strategies aimed at increasing the area of thermal necrosis achievable with RF treatment have been tested, and include the use of multiprobe arrays (14), cooled-tip electrode needles (15), bipolar arrays (16), expandable umbrella electrode needles (1, 2), and saline-enhanced RF ablation (14, 17-20). Goldberg et al. (19, 20) recently demonstrated that after injecting small volumes of highly concentrated NaCl prior to RFA, radiofrequency (RF) heating and coagulation increased remarkably. The ideal injection time of the NaCl solution is one which achieves the maximum effect on the dimension of the RF-induced coagulation, but there is little agreement as to whether this should be before, during, or before and during RF energy instillation (19, 21-23). Furthermore, in a clinical situation, RF ablation of liver tumors is performed under ultrasound guidance in many institutes, but in previous experimental studies (19, 20), RF-mediated thermal lesions were created in the liver, intraoperatively, or in tumors arising subcutaneously. It therefore seems necessary to test the feasibility, safety and possible complications of percutaneous hypertonic saline (HS)-enhanced RF ablation of the liver under ultrasound guidance before using this technique in a clinical situation. In addition, we believe that in order to maximize the effect of highly concentrated NaCl solution on the dimension of RF-induced coagulation necrosis, comparative studies aimed at ascertaining the ideal injection time are necessary.
The purpose of this investigation is to ascertain the feasibility, safety and possible complications of percutaneous RFA using 36% NaCl solution instillation to increase the extent of thermally mediated coagulation in in-vivo rabbit liver tissue and to determine the ideal injection time in relation to RF application in order to maximize its effect on the dimensions of RF-induced coagulation.
Twenty-six New Zealand white rabbits (male, 3-3.5 kg) were anesthetized by intramuscular injection of 50 mg/kg ketamine hydrochloride (Ketamine®; Yuhan, Seoul, Korea) and 5 mg/kg xylazine (Rumpun®; Bayer Korea, Ansan, Korea) prior to RFA and other procedures. Booster injections of up to one-half of the initial dose were administered as needed. For these experiments, RF-induced coagulations served as objective end points. After adequate anesthesia was achieved, the epigastrium and back were shaved and sterilized, and a 10×15-cm wire-mesh ground pad and conductive gel were placed on the animal's back.
To assess the feasibility and safety of 36% hypertonic saline (saturated saline)-enhanced RFA of normal liver parenchyma compared to conventional RFA, rabbits were assigned to one of three groups: Group A (n=8): standard RF ablation without HS infusion; Group B (n=8): 1 mL of HS instilled before RFA; or Group C (n=10): 0.5 mL of HS instilled before and during RFA. In order to assess the possibility of delayed complications, four Group C rabbits were sacrificed after undergoing contrast-enhanced CT scanning at one week (n=2) and two weeks (n=2) after RF instillation. One or two RFA lesions were induced in the liver of 26 rabbits, and all except four in Group C were sacrificed on the day of the procedure. The maximum diameters of the ablation lesions in gross specimens were measured by two observers, who reached a consensus.
One or two lesions were created in the liver of each rabbit using a 500-kHz radiofrequency (RF) generator (series CC-3; Radionics, Burlington, Mass., U.S.A.) capable of producing 200 W of power. A total of 43 lesions were produced: 13 in the Group A rabbits, ten in Group B; and 20 in Group C. An internally cooled, 17-gauge electrode (Radionics) with a 1-cm active tip was placed in the target area of the liver under US guidance, and a polyteflon-coated, 21-gauge Chiba needle (M.I.Tech, Seoul, South Korea) was then inserted using the tandem technique (25). When two lesions were created, the electrode tip was located in a different lobe.
Previous studies have shown that the concentration of NaCl solution which maximizes electrical conductivity during RF energy instillation is 36%(19, 20). This, therefore, was the concentration we selected, and to permit localized distribution of the solution within target tissue while minimizing HS leakage outside the liver, 1 mL of the solution was instilled (based on our unpublished data). To maximize the effects of instillation, the needle tip was placed 3 mm posterior to the tip of the RF electrode. Our previous study (24) showed that the initial tissue impedance of rabbit liver, measured using an electrode with a 1-cm active tip and generator, was approximately 100-140Ω, and impedance rose rapidly within 1 min of beginning RF instillation. We therefore believe that lowering tissue impedance by instilling NaCl solution before RFA could increase the current, and compared two ways of solution instillation, as follows: in Group B, 1 mL of 36% NaCl solution was slowly infused prior to the application of RF energy; in Group C, 0.5 mL of 36% NaCl solution was infused before RFA and 0.5 mL was instilled during RFA. The temperature of the injected saline, solution was 38-40℃, similar to the internal body temperature (39℃) of the rabbit. To avoid extrahepatic spillage, saline was instilled at a rate of 0.1 mL/sec.
During the procedure, a thermocouple embedded within the electrode tip continuously measured local tissue temperature. Tissue impedance was monitored by circuitry incorporated into the generator. A peristaltic pump (Watson-Marlow, Medford, Mass., U.S.A.) was used to infuse normal saline solution at 10℃ into the lumen of the electrodes at a rate sufficient to maintain a tip temperature of 20-25℃. Power output was set at 30 W, and RF energy was applied for three minutes. The applied current, power output and impedance were continuously monitored by the generator during RF ablation and were recorded automatically using a computer program (Real Time Graphics software V 2.0, Radionics).
Using a Somatom Plus 4 scanner (Siemens, Erlangen, Germany), spiral CT (2-mm slice thickness and 1.0 pitch) of the entire liver field was performed, before and after contrast injection of 6-9 mL Ultravist 370® (Schering, Berlin, Germany) at a rate of 1 mL/sec through the ear vein in order to immediately monitor the effects of RFA. Post-contrast CT scans were obtained 10, 30, and 60 secs after contrast administration. Two radiologists interpreted and compared the pre- and post-ablation CT images of all animals, reaching a consensus in each case. Each RFA lesion was evaluated in terms of it's location, size and shape; changes in attenuation; and the presence of hemorrhage or air in the peritoneal cavity. To ensure that changes had not occurred prior to RFA, pre- and post-procedural CT images were compared.
The rabbits were sacrificed by injecting an overdose of ketamine and xylazine after obtaining post-procedural (Groups A and B) and follow-up CT images (Group C). The specimens were dissected in planes similar to those of the spiral CT scans. For macroscopic examination, two observers used calipers to measure the central discolored region of coagulation necrosis in each pathologic specimen, reaching a consensus with regard to their findings. Representative lesions were then fixed in 10% formalin for routine histologic processing and were finally processed by means of paraffin sectioning and hematoxylin-eosin staining for light microscopic study. Tissues in all treatment areas were analyzed for nonviability, histologic appearance, and demarcation from surrounding viable tissue. A surgical pathologist and a radiologist determined by consensus the gross and microscopic findings at each RFA site.
Each technique was compared in terms of the technical aspects of RFA (namely, impedance and current changes), the dimensions of the RF-coagulated area, and ensuing complications, if any. For all statistical analysis, SPSS 9.0 computer software (SPSS Inc., Chicago, Ill., U.S.A.) was used. One-way analysis of variance using the Dunnett test was performed to compare the findings of the various methods of HS instillation with those obtained without saline. For all statistical analysis, a p value of less than 0.05 was considered statistically significant.
Mean initial tissue impedance was 116.4Ω ± 13 in the control group and 113.6Ω ± 15 and 110.4Ω ± 20 in Groups B and C, respectively, in which it decreased to 73.2Ω ± 5 and 68.5Ω ± 13.6, respectively, after saturated saline instillation (Table 1). This difference in impedance value before and after HS instillation was statistically significant (p < .05). Without HS instillation (Group A), impedance rose to 200-350Ω within (mean) 58 ± 8.5 seconds of application of RF energy, inducing significantly decreased current flow (less than 250 ± 105 mA) during the procedure (Fig. 1). In group B, impedance decreased with instillation of 1 mL of the solution, but approximately 90 seconds later an increase of 100-250Ω was apparent. With 0.5 mL of HS instillation prior to RFA and an additional 0.5 mL during RFA (Group C), the initial impedance decrease was maintained during RF application.
Radiofrequency ablation was technically successful in all instances, with no deaths occurring during its application. Forty-three lesions were created by RFA with or without HS instillation. After RFA, a well-defined circular lesion with brown discoloration was visible on the liver section of the lesion (Fig. 2). Maximum lesion diameters, as measured in gross specimens of the three groups, were as follows: 14.9 ± 3.8 mm in Group B, 12.5 ± 3.1 mm in Group C, and 11.5 ± 2.4 mm in Group A (p < .05) (Table 2). The mean maximum diameter of the lesions in Group B rabbits was significantly greater than in Group A (p < .05) (Fig. 3), but comparison of Group C with Group A revealed no significant difference in this respect (p > .05). In addition, the mean shortest diameters of the coagulation induced by RF application in each group of rabbits were as follows: 10.6 ± 3.7 mm in Group A, 13.5 ± 4.1 mm in Group B, and 9.7 ± 3.3 mm in Group C. The shortest diameter of coagulation was greater in Group B than in the other groups, but the differences were not statistically significant (p = .08).
Procedure-related complications occurred in six of the 26 cases (23%, 6/26), involving five localized perihepatic hematomas and a thermal injury to the stomach. Two hematomas and the thermal stomach injury occurred in Group C rabbits (3/10, 30%), while two hematomas occurred in Group B (2/8, 25%) and one in Group A (1/8, 12.5%). In addition, in 14 of 18 rabbits (78%) from Groups B and C, with HS instillation, 5-10 mL of ascites was found at autopsy to be present in the upper abdominal cavity; this seemed to be related to HS spillage into the peritoneal cavity. However, in all four rabbits sacrificed one or two weeks after the procedure, no ascites was revealed by spiral CT or laparotomy at the time of their sacrifice (Fig. 4). Complications were more frequent in Groups B and C than in Group A, though the difference was not statistically significant (p > . 05). There was no significant difference in complication rates between rabbits with a single RFA lesion (n=9) and those with two such lesions (n=17): two hematomas in rabbits with single lesions (22%) vs. three hematomas and one thermal injury in rabbits with two lesions (23.5%) (p > .05).
After RFA with HS instillation, unenhanced CT scanning demonstrated hyperattenuated regions extending from the site of the inserted electrode, though contrast-enhanced CT scanning revealed no enhancement in the region of altered hepatic parenchymal attenuation (Fig. 4). Greater conspicuity of the coagulated tissues was observed after contrast administration.
Histological examination of an acute lesion revealed a central charred zone with complete parenchymal destruction, including a small central cavity where tissue had been lost (Fig. 2). Surrounding this area were two zones of eosinophilic coagulation necrosis and a peripheral hemorrhagic rim. There was sharp cut-off between the ablation lesions and areas of normal liver. Outside this area there was viable liver tissue containing acute inflammatory cell infiltrate. In addition, histologic examination showed that the lesions of four Group C rabbits, sacrificed 1 or 2 weeks after RF ablation, exhibited typical coagulation necrosis (Fig. 4).
Previous reports (26-28) of surgical resection of primary liver tumors and hepatic metastases has clearly stated that tumor-free resection margins of less than 1 cm are directly related to increased local tumor recurrence rates. Although the percutaneous RFA technique provides a valuable alternative for patients who do not meet the surgical criteria for hepatic resection, a major limitation of the technique is the relatively high rate (34-55%) of local tumor progression after RF ablation, which seems to be related to its failure to create an adequate tumor-free margin (11, 15, 29, 30). We have observed that in clinical situations, the current commercially available RF electrode devices (1, 15, 11) or techniques (31, 32) used in thermal ablation appear unable to both ablate the clinically relevant tumor (< 3-4 cm in diameter) and provide an adequate tumor-free margin. Given, therefore, that in order to achieve complete tumor necrosis, RF ablation should cause a larger area of coagulation that could include focal liver malignancies, as well as some safety margin, strategies must be developed to further increase the volume of induced tumor destruction.
In this study, Group B rabbits, in which HS was injected prior to RFA, showed more extensive areas of coagulation necrosis than those in Group A (RFA without saline infusion) (p < .05), findings which agree with those of an earlier report (20). For RF energy instillation, we used an internally cooled electrode, knowing that this creates a larger ablation lesion than a conventional electrode (15). However, in this study, even with this cooling mechanism, tissue impedance rose during RF energy instillation, leading to decreased current flow and the formation of lesions of limited size. Upon injection of NaCl solution, however, tissue impedance fell markedly from 113.6 ± 15Ω to 73.2 ± 5Ω (p < .05). Furthermore, in animals which underwent HS infusion, there was no rapid impedance rise during RF instillation (Fig. 1), a finding which suggests that even with internal cooling of the electrode, boiling of tissue adjacent to the electrode during RF energy instillation could not be completely avoided, and that in order to decrease current density around the electrode, there is room for improvement in the biological environment of treated tissue.
A further finding was that the mean diameter of Group B lesions was greater than those in Group C (infusion of HS at 39℃ before and during RFA), a fact explained by the heat equation previously described by Pennes (33), which, as a first approximation, can be restated as "coagulation necrosis = energy deposit x local tissue interactions - heat loss" (14). The infusion of HS during RF energy instillation produced two opposite effects. First, it reduced current density around the electrode by improving electrical conductivity, leading to decreased tissue impedance followed by increased current flow into the tissue (33, 34). Second, the infusion of HS at 39℃ during RFA reduced tissue temperature, elevated to almost 100℃ by RF energy instillation, thus decreasing the size of the coagulation necrosis. The balance between these two opposite effects could determine the final extent of the area of coagulation necrosis; in this study, the diameter of RF-induced coagulation was greater in group B rabbits than in group C. We speculated that with the instillation of 0.5 mL HS in Group C rabbits, initial tissue impedance was markedly reduced (and kept low) during RF energy instillation, and that the gain in electrical energy thus achieved could be small and counteracted by heat loss due to the lower temperature of the solution compared to that of treated tissue (Fig. 1).
In this study, procedure-related complications, namely localized hematomas (n=5) and thermal injury to the stomach (n=1), occurred in six of the 26 cases (23%); incidence rates were not significantly higher in rabbits with HS instillation (28%) than in those without (13%) (p > .05). Interestingly, there was only one unexpected thermal injury to the stomach among 18 rabbits which underwent HS instillation (1/18: 6%), a proportion much lower than that reported previously (5/7: 71%) in a study describing the application of saline-enhanced RFA to VX2 tumors embedded in fat (23). We speculate that this difference between our study and the earlier one could be related to the amount of injected saline (1 mL of 36% HS in ours, compared with 5-10 mL of physiologic saline earlier). The greater the amount of saline used, the greater the complications that can occur; irregular RF zones may, for example, be created, thereby increasing the potential risk of unexpected thermal injury or seeding as tumor cells are carried by fluid (22). The use of a small volume of highly concentrated (36%) NaCl solution may thus be better than the use of a larger volume of lower concentration.
Our study suffers certain limitations. First, the results obtained in rabbit liver tissue may differ from those obtained with hepatocellular carcinomas or liver metastases in human liver. However, on the basis of the results of previous studies regarding cell degeneration caused by the effect of heating on tissue (32, 35), we believe that the same results may occur with either normal liver tissue or human cancer cells. Second, a rabbit's liver is small and we therefore restricted RF energy to a lower level and shortened the time of RF instillation compared to the clinical parameters (1, 10, 11, 13). To explore the positive effects of HS instillation on coagulation necrosis and its negative effects in producing unwanted thermal injury to adjacent structures before or during RFA, an experimental study using radiological guidance in a large animal model of clinically relevant size will therefore be necessary before clinical application of RFA with HS instillation for the treatment of liver malignancies. Third, we tested only 1 mL of 36% NaCl solution and believe that further experimental study for optimization of the concentration and amount of HS solution is warranted. Last, at autopsy after percutaneous RFA, it was very difficult to find a plane perpendicular to the axis of the electrode insertion, and to measure RF-induced coagulation necrosis we therefore dissected the specimens in planes similar to those of the spiral CT scans. Since these might not parallel the axis of the electrode, the measured dimensions of the necrotic area in those cases could be smaller than the real dimensions.
In conclusion, RF liver ablation performed just before the instillation of HS is more effective for achieving coagulation necrosis than RFA without saline infusion or with HS infusion before and during the procedure. We believe that the greater volume of coagulation necrosis created by HS infusion increases the clinical utility of RFA therapy by allowing successful treatment of larger tumors or by reducing the number of sessions needed for treatment.
Figures and Tables
Fig. 1
Graphic depiction of the changes occurring in tissue impedance, RF current and power during radiofrequency ablation in the four groups. Left: In a Group A rabbit, tissue impedance increased sharply and current decreased during RF energy instillation. Middle and Right: In Group B rabbits (middle) and those of Group C (right), an abrupt increase in tissue impedance after RF energy instillation was prevented by the infusion of NaCl solution.
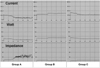
Fig. 2
Radiofrequency ablation without the use of saturated saline in a Group A rabbit.
A. Left: A 17-gauge RF electrode and a 21-gauge coated Chiba needle (arrow) were placed in the left lobe of the liver under ultrasound guidance. Right: After radiofrequency ablation, an echogenic area (arrow) was produced around the electrode.
B. At post-procedural CT scanning, a 10-mm round defect (arrow) corresponding to the area of coagulative necrosis is apparent.
C. Gross specimen demonstrating the presence of a light brown, coagulative, 10-mm necrotic zone surrounded by a peripheral hemorrhagic rim (arrows).
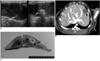
Fig. 3
Radiofrequency ablation with saturated saline pretreatment in a Group B rabbit.
A. At post-procedural CT scanning, a 15-mm diameter, oval-shaped perfusion defect (arrow) is seen.
B. Gross specimen shows that the liver contains a light brown coagulative necrotic zone (arrowhead), 15-mm in diameter.
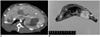
Fig. 4
Radiofrequency ablation with saturated saline infusion before and during the procedure in a Group C rabbit.
A. Contrast-enhanced transverse CT scan of the liver obtained one week after RF ablation; two wedge-shaped defects (arrows) are seen.
B. Gross specimen demonstrating the presence of two light brown coagulative necrotic zones (arrows) in the liver.
C. Microscopic image shows typical coagulation necrosis (arrows) of the ablated lesions.
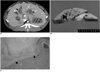
Acknowledgements
The authors wish to thank Seon Ok Lee, R.T., for her assistance in animal observation and anesthesia and for her outstanding support in performing CT imaging. We also wish to thank Bonnie Hami, M.A., Department of Radiology, University Hospitals of Cleveland, for her editorial assistance and manuscript preparation.
References
1. Lim HK. Radiofrequency thermal ablation of hepatocellular carcinomas. Korean J Radiol. 2000. 1:175–184.
2. Curley SA, Izzo F, Ellis LM, Vauthey JN, Vallone P. Radiofrequency ablation of hepatocellular cancer in 110 patients with cirrhosis. Ann Surg. 2000. 232:381–391.
3. Curley SA, Izzo F, Delrio P, Ellis LM, et al. Radiofrequency ablation of unresectable primary and metastatic hepatic malignancies: results in 123 patients. Ann Surg. 1999. 230:1–8.
4. Rossi S, Di Stasi M, Buscarini E, et al. Percutaneous RF interstitial thermal ablation in the treatment of hepatic cancer. AJR Am J Roentgenol. 1996. 167:759–768.
5. Goldberg SN, Livraghi T, Solbiati L, Gazelle GS. Gazelle GS, Saini S, Mueller PR, editors. In-situ ablation of focal hepatic neoplasms. Hepatobiliary and pancreatic radiology: imaging and intervention. 1997. New York: Thieme;470–502.
6. Gazelle GS, Goldberg SN, Solbiati L, Livraghi T. Tumor ablation with radiofrequency energy. Radiology. 2000. 217:633–646.
7. Gervais DA, McGovern FJ, Wood BJ, Goldberg SN, McDougal WC, Mueller PR. Radio-frequency ablation of renal cell carcinoma: early clinical experience. Radiology. 2000. 217:665–672.
8. Rosenthal DI, Hornicek FJ, Wolfe MW, Jennings LC, Gebhardt MC, Mankin HJ. Percutaneous radiofrequency coagulation of osteoid osteoma compared with operative treatment. J Bone Joint Surg Am. 1998. 80:815–821.
9. Jeffrey SS, Birdwell RL, Ikeda DM, et al. Radiofrequency ablation of breast cancer: first report of an emerging technology. Arch Surg. 1999. 134:1064–1068.
10. Anzai Y, Lufkin R, DeSalles A, Hamilton DR, Farahani K, Black KL. Preliminary experience with MR-guided thermal ablation of brain tumors. AJNR Am J Neuroradiol. 1995. 16:39–48.
11. Livraghi T, Goldberg SN, Lazzaroni S, et al. Hepatocellular carcinoma: radiofrequency ablation of medium and large lesions. Radiology. 2000. 214:761–768.
12. de Baere T, Elias D, Dromain C, et al. Radiofrequency ablation of 100 hepatic metastases with a mean follow-up of more than one year. AJR Am J Roentgenol. 2000. 175:1619–1625.
13. Dodd GD, Frank MS, Aribandi M, Chopra S, Chintapalli KN. Radiofrequency thermal ablation: computer analysis created by overlapping ablations. AJR Am J Roentgenol. 2002. 177:777–782.
14. Goldberg SN, Gazelle GS, Mueller PR. Thermal ablation therapy for focal malignancy: a unified approach to underlying principles, techniques, and diagnostic imaging guidance. AJR Am J Roentgenol. 2000. 174:323–331.
15. Solbiati L, Goldberg SN, Ierace T, et al. Hepatic metastases: percutaneous radiofrequency ablation with cooled-tip electrodes. Radiology. 1997. 205:367–373.
16. McGahan JP, Gu WZ, Brock JM, Tesluk H, Jones CD. Hepatic ablation using bipolar radiofrequency electrocautery. Acad Radiol. 1996. 3:418–422.
17. Munver R, Threatt CB, Delvecchio FC, Preminger GM, Polascik TJ. Hypertonic saline-augmented radiofrequency ablation of VX2 tumor implanted in rabbit kidney: a short-term survival pilot study. Urology. 2002. 60:170–175.
18. Livraghi T, Goldberg SN, Monti F, et al. Saline-enhanced radiofrequency tissue ablation in the treatment of liver metastases. Radiology. 1997. 202:205–210.
19. Goldberg SN, Ahmed M, Gazelle GS, et al. Radiofrequency thermal ablation with NaCl solution injection: effect of electrical conductivity on tissue heating and coagulation-phantom and porcine liver study. Radiology. 2001. 219:157–165.
20. Ahmed M, Lobo SM, Weinstein J, et al. Improved coagulation with saline solution pretreatment during radiofrequency tumor ablation in a canine model. J Vasc Interv Radiol. 2002. 13:717–724.
21. Miao Y, Ni Y, Yu J, Marchal G. A comparative study on validation of a novel cooled-wet electrode for radiofrequency liver ablation. Invest Radiol. 2000. 35:438–444.
22. Boehm T, Malich A, Reichenbach JR, Fleck M, Kaiser WA. Percutaneous radiofrequency (RF) thermal ablation of rabbit tumors embedded in fat: a model for RF ablation of breast tumors. Invest Radiol. 2001. 36:480–486.
23. Boehm T, Malich A, Goldberg SN, et al. Radiofrequency tumor ablation: internally cooled electrode versus saline-enhanced technique in an aggressive rabbit tumor model. Radiology. 2002. 222:805–813.
24. Lee JD, Lee JM, Kim SW, Kim CS, Mun WS. MR imaging-histopathologic correlation of radiofrequency thermal ablation lesion in a rabbit liver model: observation during acute and chronic stages. Korean J Radiol. 2001. 2:151–158.
25. Haaga JR. Haaga JR, Lanzieri CF, editors. Interventional CT-guided procedures. Computed tomography and magnetic resonance imaging of the whole body. 1994. 3rd ed. St.Louis: Mosby Yearbook;1572–1693.
26. Neeleman N, Andersson R. Repeated liver resection for recurrent liver cancer. Br J Surg. 1996. 83:893–901.
27. Cady B, Jenkins RL, Steele GD Jr, et al. Surgical margin in hepatic resection for colorectal metastases: a critical and improvable determinant of outcome. Ann Surg. 1998. 227:566–571.
28. Elias D, Cavalcanti A, Sabourin JC, et al. Resection of liver metastases from colorectal cancer: the real impact of the surgical margin. Eur J Surg Oncol. 1998. 24:174–179.
29. McGahan JP, Dodd GD III. Radiofrequency ablation of the liver: current status. AJR Am J Roentgenol. 2001. 176:3–16.
30. Kainumao O, Asano T, Aoyama H, et al. Combined therapy with radiofrequency thermal ablation and intra-arterial infusion chemotherapy for hepatic metastases from colorectal cancer. Hepatogastroenterology. 1999. 46:1071–1077.
31. Goldberg SN, Stein M, Gazelle GS, Sheiman RG, Kruskal JB, Clouse ME. Percutaneous radiofrequency tissue ablation: optimization of pulsed-RF technique to increase coagulation necrosis. J Vasc Interv Radiol. 1999. 10:907–916.
32. Goldberg SN. Radiofrequency tumor ablation: principles and techniques. Eur J Ultrasound. 2001. 13:129–147.
33. Pennes HH. Analysis of tissue and arterial blood temperatures in the resting human forearm. J Appl Physiol. 1948. 1:93–122.
34. Nath S, Haines DE. Biophysics and pathology of catheter energy delivery systems. Prog Cardiovasc Dis. 1995. 37:185–204.
35. Rhim HS, Goldberg SN, Dodd GD II, et al. Essential techniques for successful radiofrequency thermal ablation of malignant hepatic tumors. Radiographics. 2002. 21:S17–S39.