Abstract
Objective
To analyze the contrast-enhancement patterns obtained at pulse-inversion harmonic imaging (PIHI) of focal hepatic lesions, and to thus determine tumor vascularity and the acoustic emission effect.
Materials and Methods
We reviewed pulse-inversion images in 90 consecutive patients with focal hepatic lesions, namely hepatocellular carcinoma (HCC) (n=43), metastases (n=30), and hemangioma (n=17). Vascular and delayed phase images were obtained immediately and five minutes following the injection of a microbubble contrast agent. Tumoral vascularity at vascular phase imaging and the acoustic emission effect at delayed phase imaging were each classified as one of four patterns.
Results
Vascular phase images depicted internal vessels in 93% of HCCs, marginal vessels in 83% of metastases, and peripheral nodular enhancement in 71% of hemangiomas. Delayed phase images showed inhomogeneous enhancement in 86% of HCCs; hypoechoic, decreased enhancement in 93% of metastases; and hypoechoic and reversed echogenicity in 65% of hemangiomas. Vascular and delayed phase enhancement patterns were associated with a specificity of 91% or greater, and 92% or greater, respectively, and with positive predictive values of 71% or greater, and 85% or greater, respectively.
Ultrasonography (US) has been used as a non-invasive imaging technique for the detection, characterization and staging of focal hepatic lesions. Unfortunately, since most tumors have nonspecific morphologic features, the usefulness of US for the characterization of hepatic masses is limited. Although color and power Doppler US can provide vascular information, they are less sensitive than angiography for the detection of small vessels, and suffer limitations when hepatic masses are small. Both these modalities are also subject to motion artifacts arising from respiratory and cardiac activity (1, 2).
New ultrasound imaging technologies such as the harmonic technique (3), used with various sonographic contrast agents (4-9), are able to overcome the limitations of conventional and Doppler US. When used with a microbubble contrast agent, pulse-inversion harmonic imaging (PIHI) relies on the asymmetric oscillation of ultrasound bubbles in an acoustic field (10), and used in conjunction with a low mechanical index (MI) technique, allows nondestructive, continuous imaging after injection of a microbubble agent; it can also detect blood flow in the capillary bed within the tumor (tumoral vascularity). In addition, a high-MI technique destroys accumulated microbubbles, causing them to release high-intensity, nonlinear ultrasound echoes which can be optimally detected by delayed phase imaging (10, 11).
Previous studies have demonstrated that compared with conventional US and spiral CT, the use of stimulated acoustic emission imaging improves the conspicuity of hepatic metastases (12, 13). A number of recent studies have reported the value of contrast-enhanced harmonic imaging for the characterization of blood flow in focal hepatic lesions (11, 14-17), but none have described the use of both vascular and delayed phase P1H1 imaging for the characterization of focal hepatic lesions. The purpose of this study is to describe our experience in categorizing focal hepatic lesions on the basis of patterns of tumoral vascularity, as seen at low-MI continuous imaging, as well as the patterns of acoustic emission effect observed at delayed phase imaging with a high MI after injection of a microbubble contrast agent.
Between September 2000 and August 2001, 135 consecutive patients with hepatic masses underwent pulse-inversion harmonic US. A confirmed diagnosis of hepatic mass in one of three categories - hepatocellular carcinoma (HCC), metastasis, or hemangioma - was the only criterion for inclusion. Patients in whom HCC had been treated by transarterial chemoembolization or radiofrequency ablation prior to US examination (n=21), or had a dysplastic nodule (n=8), inflammatory eosinophilic granuloma (n=4) or focal nodular hyperplasia (n=2), were excluded, as were those (n=10) for whom chemotherapy was ongoing or had been performed within the previous 30 days. Thus, 90 patients [60 men and 30 women; mean age, 57 (range 23-79) years] with focal hepatic lesions, including HCC (n=43), metastasis (n=30) and hemangioma (n=17), were included in this study. Informed consent was obtained from all patients and the approval of our institutional review board was obtained.
Five patients with HCCs and 22 with metastases had multiple (two to seven) lesions. In patients with multiple HCCs or metastatic lesions, only one dominant lesion per patient was analyzed (determined on the basis of size). A diagnosis of HCC was confirmed by US-guided percutaneous needle biopsy in 24 patients and at surgery in three. In the remaining 16 patients, HCC was diagnosed on the basis of the typical imaging findings of three-phase helical CT, angiography and/or CT after intra-arterial injection of iodized oil, as well as elevated levels of serum alpha-fetoprotein (> 200 ng/mL). For metastatic liver tumors, the sites of primary disease were the stomach (n=13), colon (n=10), pancreas (n=5) and lung (n=2). Radiological or histological study showed that no metastatic lesion was hypervascular. For 27 of the 30 patients with hepatic metastases, the diagnosis was histologically confirmed at surgery (n=7) or by biopsy (n=20). In the remaining three cases, diagnosis was based on follow-up serial CT findings showing that the lesions had progressed rapidly. All patients with hemangioma underwent dynamic contrast-enhanced CT (n=17) or MR (n=9) examination. For 16 of the 17 hemangiomas, diagnosis was based on typical imaging findings; the other was diagnosed at biopsy. The HCCs ranged in size from 1.0 to 7.0 (mean, 3.5) cm, metastases from 1.0 to 6.0 (mean, 2.9) cm, and hemangiomas from 1.0 to 4.0 (mean, 2.4) cm.
Pulse-inversion harmonic US was performed by one investigator (K.H.Y.), using a commercially available US system (HDI-5000; Advanced Technology Laboratory, Bothell, Washington, U.S.A.) together with a 5-2 MHz curved linear-array transducer. To assess tumor vascularity, continuous low-MI imaging (< 0.3) was performed immediately following injection and during the delivery of a microbubble contrast agent (the first 60-second period after contrast injection). The second contrast agent was injected five minutes after the first. Five minutes later, high-MI (1.0-1.3) delayed phase images were obtained, and the acoustic emission effect thus determined. During the interval between the vascular phase and delayed phase, the display was frozen and 'interval-delay scanning' was not performed. The microbubble contrast agent used was SH U 508 A (Levovist; Schering, Berlin, Germany), a 2.5 gm bolus of which was injected twice, for vascular and delayed imaging, at a concentration of 300 mg/mL followed by a 10-mL normal saline flush using a 20-gauge peripheral intravenous cannula.
When the area of interest was identified at conventional US, PIHI was activated, with the focal zone set at or just deeper than the level of interest. All imaging was performed with the transducer in a constant position over the region of interest in order to depict the lesion and to include some normal liver. While the contrast agent was being delivered, during a period of shallow breathing, scanning was performed and the enhanced blood signals in both normal hepatic blood vessels and lesional blood vessels were depicted. During low-MI imaging, a medium frame rate was set (8 per second). High-MI scanning, on the other hand, was performed during the shortest possible period (and with breath-hold), in order to reduce the amount of microbubble disruption in the region of interest outside, and the display was then frozen. In order to destroy microbubbles with insonation and to identify white flashes of contrast break-up, we used a medium pulse length, the maximum mechanical index, a high frame rate (15 per second) and low line density. After freezing the display, we reviewed the images using a cine loop and stored the strongest effects seen during the first and second frames. All studies were recorded on S-VHS video, and with selected digital images and cine loops were stored on a magneto-optical disk.
Ultrasonic images were reviewed retrospectively by three radiologists (E.A.K., S.K.J., J.J.W.), who were unaware of the pathologic diagnosis or other radiologic imaging findings. Each had more than five years of experience of ultrasonography involving the use of contrast agents.
The reviewers determined the diameters and echogenicity of the tumors, as seen on unenhanced conventional and pre-contrast pulse-inversion harmonic images. The pattern of contrast enhancement of each tumor depicted by dualphase pulse-inversion harmonic US was evaluated by examining the images obtained during the vascular phase of enhancement (typically 20-60 seconds after contrast injection), and during the delayed phase (5 minutes after a separate injection of contrast agent). All observations of enhancement patterns were totally subjective. In each patient, tumoral vascularity of the dominant lesion, as seen on vascular phase images, was classified as one of four patterns, as follows (Fig. 1). The presence of linear or branched internal vessels was categorized as the 'internal vessels' pattern; that of linear or dot-like marginal vessels at the periphery of the tumor, regardless of the presence or absence of central vessels, as the 'marginal vessels' pattern; that of a discrete and well-defined ring of peripheral enhancing nodules, as 'peripheral nodular enhancement'; and the absence of visible vessels or enhancement in either internal or peripheral areas of the tumor, as the 'minimal or no enhancement' pattern. The acoustic emission effect within the tumor, as seen on delayed phase images, was also classified as one of four patterns (Fig. 2). 'Inhomogeneous enhancement' was defined as irregular and heterogeneous enhancement within the tumor; 'hypoechoic, decreased enhancement' as the presence of contrast, but decreased enhancement with respect to adjacent liver parenchyma; 'isoechoic, homogeneous enhancement' as homogeneous enhancement, similar to that of adjacent liver parenchyma; and the term 'hypoechoic and reversed echogenicity' was used to describe a tumor represented as a hyperechoic lesion on unenhanced US images and as a reversed hypoechoic lesion on delayed phase images.
Agreement regarding the pattern analysis of all lesions was reached by consensus; disagreements were resolved by majority opinion. Sensitivity and specificity were determined for the different histologic diagnoses, and positive predictive values (PPV) for the various observed enhancement patterns.
Vascular phase low-MI enhancement patterns for tumoral vascularity in the 90 patients with focal hepatic lesions are summarized in Table 1. Forty-four lesions [40 HCCs (91%) and four metastases (9%)] demonstrated the 'internal vessels' pattern, while for 27 lesions [25 metastases (93%) and two HCCs (7%)], the 'marginal vessels' pattern was observed. Twelve lesions [12 hemangiomas (100%)] showed peripheral nodular enhancement, and in seven cases [five hemangiomas (71%), one HCC (14%) and one metastasis (14%)], the observed pattern was 'minimal or no enhancement'. Table 2 summarizes the vascular phase enhancement patterns for tumor vascularity associated with each diagnosis, and the corresponding sensitivity, specificity, and PPV values. For HCCs, three patterns were observed: 'internal vessels' [93% (40/43) (Fig. 3)]; 'marginal vessels' [5% (2/43)]; and 'minimal or no enhancement' [2% (1/43)]. For metastases, the same three enhancement patterns occurred: 'internal vessels' [13% (4/30)]; 'marginal vessels' [83% (25/30) (Fig. 4)]; and 'minimal or no enhancement' [3% (1/30)], while for hemangiomas, there were two: 'peripheral nodular enhancement' [71% (12/17) (Fig. 5)] and 'minimal or no enhancement' [29% (5/17)]. The observed patterns were associated with a specificity of 91% or greater, and PPVs of 71% or greater: 'internal vessels' for HCCs; 'marginal vessels' for metastases; 'peripheral nodular enhancement' for hemangiomas.
Delayed phase high-MI acoustic emission effect patterns within the tumor are summarized in Table 3. Thirty-nine lesions [37 HCCs (95%) and two metastases (5%)] demonstrated inhomogeneous enhancement, while 33[28 metastases (85%) and five HCCs (15%)] showed hypoechoic, decreased enhancement. In 11 tumors, all of which were hemangiomas, hypoechoic and reversed echogenecity was observed, and in seven [six hemangiomas (86%) and one HCC (14%)], isoechoic, homogeneous enhancement was noted.
Table 4 summarizes the delayed phase the acoustic emission effect patterns associated with each diagnosis, and the corresponding sensitivity, specificity, and PPV values. For HCCs, three enhancement patterns were observed: 'inhomogeneous enhancement' [86% (37/43) (Fig. 3)], 'hypoechoic, decreased enhancement' [12% (5/43)], and 'isoechoic, homogeneous enhancement' [2% (1/43)]. For metastases, two patterns were apparent: 'hypoechoic, decreased enhancement' [93% (28/30) (Fig. 6)], and 'inhomogeneous enhancement' [7% (2/30)]. Neither conventional nor unenhanced US revealed the presence of a hyperechoic lesion. Hemangiomas showed two enhancement patterns: 'hypoechoic and reversed echogenicity' [65% (11/17) (Fig. 7)], and 'isoechoic, homogeneous enhancement' [35% (6/17) (Fig. 5)]. The above patterns were associated with a specificity of 92% or greater, and PPVs of 85% or greater: 'inhomogeneous enhancement' for HCCs; 'hypoechoic, decreased enhancement' for metastases; and for hemangiomas, 'hypoechoic and reversed echogenicity' or 'isoechoic, homogeneous enhancement'.
This study has demonstrated that vascular phase PIHI with a low MI and delayed phase PIHI with a high MI after the administration of a microbubble contrast agent may be able to differentiate hepatic tumors such as HCC, hemangioma, and metastasis. Wilson et al. (11) reported their initial experience of harmonic hepatic US with microbubble contrast agent for the characterization of focal hepatic lesions. Although their study and ours showed similar results, ours was different in two respects: firstly, we used SH U 508 A, which consists of galactose microaggregates with a small admixture of palmitic acid. Wilson et al., on the other hand, used a perfluorocarbon microbubble agent, which is a blood pool contrast agent comprising microbubbles stabilized by a protein shell. Although we know of no comparative studies of the various microbubble contrast agents, perfluorocarbon agents are more highly regarded than Levovist for vascular imaging because of their stability (16); Levovist, however, because of its unique liver-specific action, is considered better at providing the stimulated acoustic emission effect at delayed phase imaging (18). Secondly, for delayed phase pulse-inversion imaging we used a high MI, not the interval delay procedure. Kim et al. (14) recently reported that PIHI with an interval delay technique was useful for the characterization of hepatic tumors. Although they found that the technique demonstrated specific enhancement features of hepatic hemangiomas, it was not suitable for demonstrating malignant hepatic tumors such as HCCs and metastases. Ko et al. (19), on the other hand, showed that delayed phase imaging with gray-scale stimulated acoustic emission might be useful for differentiating between HCC and metastatic adenocarcinoma of the liver.
In our study, vascular phase imaging with a low MI detected the morphological characteristics of tumoral vessels. During delivery of the contrast agent, enhanced signals were emitted by both normal hepatic and lesional blood vessels. In HCCs, the 'internal vessels' pattern was striking; the characteristics of these vessels were distinct, unlike those of hemangiomas and metastatic tumors. Jang et al. (15) found that at the vascular imaging phase of the coded harmonic angio technique, a majority of HCCs were depicted as irregular branching vessels or showed randomly stippled vascularity. We believe that even though the US technique employed was somewhat different, their vascular phase imaging findings and ours were similar. For hemangiomas, the observed pattern was 'peripheral nodular enhancement' or 'minimal or no enhancement'; in no case were internal or marginal vessels observed. As in previous studies (14-16), our results showed that 'peripheral nodular enhancement', observed at vascular phase imaging, was a highly specific finding for the diagnosis of hemangiomas. However, for five of the 17 tumors fo this kind, the observed pattern was 'minimal or no enhancement', a result different from that of previous studies (14-15). We believe our results were due to the relatively low sensitivity of the PIHI technique itself when used to evaluate the vascularity of hemangiomas, the dosage of the contrast agent injected, and the histologic characteristics of the tumor.
At delayed phase imaging with a high MI using PIHI, the intensity of the echo was proportional to the number of microbubbles present within the ultrasound beam, reflecting the amount of microbubbles in a tumor's sinusoids and Kupffer cells (11). Although not certain, it is thought that microbubbles are simply retained in the sinusoidal spaces or are encapsulated by Kupffer cells (12). Because the majority of hepatic tumors do not contain sinusoids or Kupffer cells, these tumors appear at stimulated acoustic emission imaging as defects or signal loss compared to normal hepatic parenchyma. HCCs do not usually contain Kupffer cells, though some types, such as well-differentiated HCC and early HCC, may contain a portion of normal-appearing hepatocytes or Kupffer cells. Profuse intratumoral vessels, as well as the sinusoids and reticuloendothelial cells within some types of HCC, might thus appear as bright emission signals, the so-called 'inhomogeneous enhancement' seen on delayed phase images. In our study, 86% of HCCs showed inhomogeneous enhancement, a result somewhat different from those of previous studies (15, 16), in which a hypoechoic washout pattern or no enhancement was observed at interval delay or postvascular imaging.
The majority of metastases were seen as 'hypoechoic, decreased enhancement', indicating that metastatic tumors are usually hypovascular and do not contain reticuloendothelial cells. In some hepatic metastases, however, acoustic emission signals were present at their periphery; this was thought to represent capillarization of the sinusoid, and peripheral fibrosis of the hepatic parenchyma (21). Although a small minority of the metastases in our study showed inhomogeneous enhancement, in 93% of cases the observed pattern was 'hypoechoic, decreased enhancement', a finding similar to those previously reported (14-17).
All hemangiomas showed one of two patterns, namely 'decreased and reversed echogenicity' or 'isoechoic, homogeneous enhancement'. The former indicated that a lesion which was echogenic to surrounding liver at baseline imaging became echo-poor at delayed imaging, suggesting that hemangiomas have fewer sinusoids than normal liver. Hemangiomas showing 'isoechoic, homogeneous enhancement', on the other hand, were those with large sinusoidal spaces, as described by Yamashita et al. (20). As stated above, hemangioma-related findings at interval delay imaging are specific. The histopathology of hemangiomas varies, however, according to their tissue components and vascular pools, and the imaging findings thus present a spectrum, with various patterns observed at dynamic contrast-enhanced CT or MR. We therefore believe that delayed phase imaging of hemangiomas may demonstrate various patterns, and in order to evaluate tissue characteristics in terms of the relationship between histologic features and delayed-phase PIHI findings, further study may be warranted.
This study suffers certain limitations. Firstly, SH U 508 A, used as a contrast agent, is known to have a weak harmonic response when insonated with an ultrasound beam at a low MI (16). The microbubbles are destroyed even at continuous vascular phase imaging, for which Levovist is a less suitable agent. We therefore suggest that in our study, vascular phase imaging might not have depicted tumoral vascularity sufficiently. The second limitation is the inherent drawbacks of US techniques used in this study. Continuous vascular phase US scanning is possible in only one scanning plane, and in patients with multiple lesions it is therefore not possible to characterize all lesions simultaneously. Nor can delayed phase scanning simultaneously include all lesions, and since its duration is very short, it is not easy to obtain the best images depicting the acoustic emission effect in the same area as vascular phase images.
In conclusion, vascular and delayed phase PIHI appears to be a useful imaging technique for the characterization of focal hepatic lesions. The depiction of tumoral vascularity and the acoustic emission effect at dual-phase PIHI after the injection of a microbubble contrast agent can help differentiate HCC, metastasis, and hemangioma.
References
1. Choi BI, Kim TK, Han JK, Chung JW, Park JH, Han MC. Power versus conventional color Doppler sonography: comparison in the depiction of vasculature in liver tumors. Radiology. 1996; 200:55–58. PMID: 8657945.


2. Lencioni R, Pinto F, Armillotta N, Bartolozzi C. Assessment of tumor vascularity in hepatocellular carcinoma: comparison of power Doppler US and color Doppler US. Radiology. 1996; 201:353–358. PMID: 8888222.


3. Burns PN. Harmonic imaging with ultrasound contrast agents. Clin Radiol. 1996; 51:50–55. PMID: 8605774.
4. Goldberg BB, Liu J, Burns PN, Merton DA, Forsberg F. Galactose-based intravenous sonographic contrast agent: experimental studies. J Ultrasound Med. 1993; 12:463–470. PMID: 8411330.


5. Cosgrove D. Why do we need contrast agents for ultrasound? Clin Radiol. 1996; 51:1–4. PMID: 8605763.
6. Schineider M, Broillet A, Bussat P, et al. Gray-scale liver enhancement in VX2 tumor-bearing rabbits using BR14, a new ultrasonographic contrast agent. Invest Radiol. 1997; 32:410–417. PMID: 9228607.
7. Forsberg F, Goldberg BB, Liu J, Merton DA, Rawool NM, Shi WT. Tissue-specific US contrast agent for evaluation of hepatic and splenic parenchyma. Radiology. 1999; 210:125–132. PMID: 9885597.


8. Hauff PD, Fritzsch TD, Reinhardt M, et al. Delineation of experimental liver tumors in rabbits by a new ultrasound contrast agent and stimulated acoustic emission. Invest Radiol. 1997; 32:94–99. PMID: 9039581.


9. Kono Y, Moriyasu F, Nada T, et al. Gray scale second harmonic imaging of the liver: a preliminary animal study. Ultrasound Med Biol. 1997; 23:719–726. PMID: 9253819.


10. Burns PN, Wilson SR, Simpson DH. Pulse inversion imaging of liver blood flow: improved method for characterizing focal masses with microbubble contrast. Invest Radiol. 2000; 35:58–71. PMID: 10639037.
11. Wilson SR, Burns PN, Muradali D, Wilson JA, Lai X. Harmonic hepatic US with microbubble contrast agent: initial experience showing improved characterization of hemangioma, hepatocellular carcinoma, and metastasis. Radiology. 2000; 215:153–161. PMID: 10751481.


12. Blomley MJK, Albrecht T, Cosgrove DO, et al. Improved imaging of liver metastases with stimulated acoustic emission in the late phase of enhancement with the US contrast agent SH U 508A: early experience. Radiology. 1999; 210:409–416. PMID: 10207423.


13. Dalla-Palma L, Bertolotto M, Quaia E, Locatelli M. Detection of liver metastases with pulse-inversion harmonic imaging: preliminary results. Eur Radiol. 1999; 9:S382–S387. PMID: 10602934.
14. Kim TK, Choi BI, Han JK, Hong HS, Park SH, Moon SG. Hepatic tumors: contrast agent-enhancement patterns with pulse-inversion harmonic US. Radiology. 2000; 216:411–417. PMID: 10924562.


15. Jang HJ, Lim HK, Lee WJ, et al. Focal hepatic lesions: evaluation with contrast-enhanced gray-scale harmonic US. Korean J Radiol. 2003; 4:91–100. PMID: 12845304.


16. Dill-Macky MJ, Burns PN, Khalili K, Wilson SR. Focal hepatic masses: enhancement patterns with SH U 508A and pulse-inversion US. Radiology. 2002; 222:95–102. PMID: 11756711.


17. Albrecht T, Hoffmann CW, Schmitz S, et al. Phase-inversion sonography during the liver-specific late phase of contrast enhancement: improved detection of liver metastases. AJR Am J Roentgenol. 2001; 176:1191–1198. PMID: 11312180.
18. Blomley MJK, Albrecht T, Cosgrove DO, et al. Stimulated acoustic emission to image a late liver and spleen-specific phase of Levovist® in normal volunteers and patients with and without liver disease. Ultrasound Med Biol. 1999; 25:1341–1352. PMID: 10626621.


19. Ko CJ, Yoon KH. Gray-scale stimulated acoustic emission: differential diagnosis between hepatocellular carcinoma and metastatic adenocarcinoma. J Korean Radiol Soc. 2001; 44:63–68.
20. Yamashita Y, Ogata I, Urata J, Takahashi M. Cavernous hemangioma of the liver: pathologic correlation with dynamic CT findings. Radiology. 1997; 203:121–125. PMID: 9122378.


21. Baker ME, Pelley R. Hepatic metastases: basic principles and implications for radiologists. Radiology. 1995; 197:329–337. PMID: 7480672.


Fig. 1
The contrast-enhancement patterns observed at vascular phase pulse-inversion harmonic imaging of hepatic tumors.
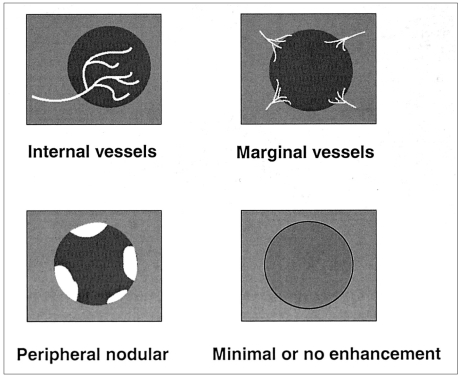
Fig. 2
The contrast-enhancement patterns seen at delayed phase pulse-inversion harmonic imaging of hepatic tumors.
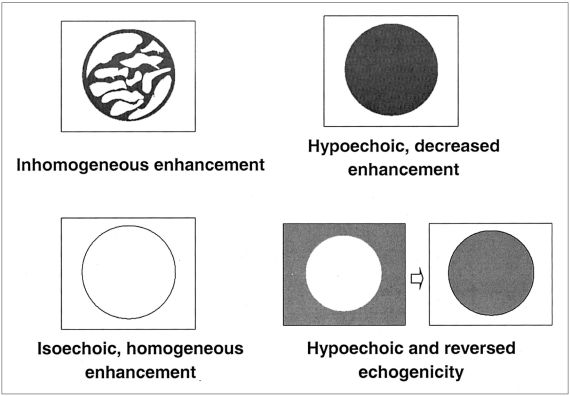
Fig. 3
Hepatocellular carcinoma showing the 'internal vessels' pattern during the vascular phase and 'inhomogeneous enhancement' during the delayed phase.
A. Pulse-inversion harmonic US image before injection of the contrast agent depicts a hyperechoic mass (arrow).
B, C. Vascular phase pulse-inversion harmonic US low-MI images obtained 15 sec (B) and 45 sec (C) after contrast injection depict the tumor's internal vessels (arrows).
D. Delayed phase image (high MI) obtained five minutes after contrast injection demonstrates inhomogeneous enhancement (arrow) and the acoustic emission effect in hepatic parenchyma.
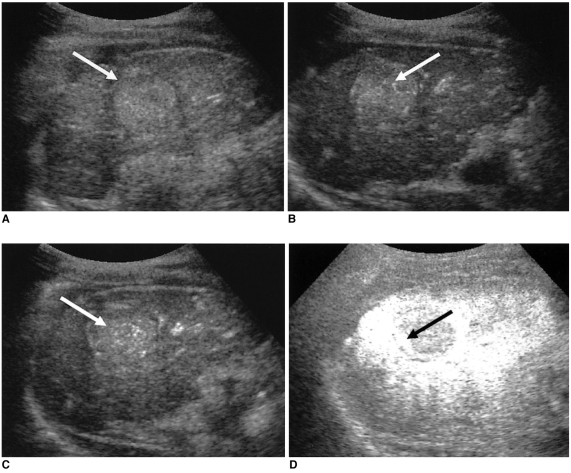
Fig. 4
Metastases from colon cancer showing 'marginal vessels' and 'hypoechoic, decreased enhancement'.
A. Pulse-inversion harmonic US image obtained before contrast injection shows a hypoechoic mass (arrow).
B, C. Vascular phase pulse-inversion harmonic US images (low MI) obtained 35 sec (B) and 54 sec (C) after contrast injection depict marginal tumor vessels (arrows) and some central vessels.
D. Delayed phase image (high MI) shows a hypoechoic mass with decreased enhancement (arrow) compared to the acoustic emission effect of surrounding normal hepatic parenchyma.
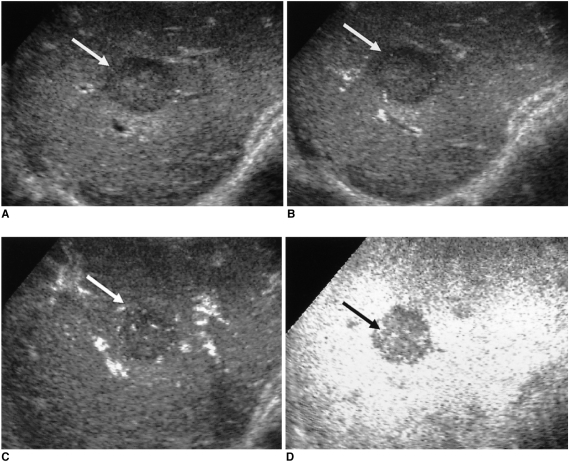
Fig. 5
Hemangioma demonstrating 'peripheral nodular enhancement' and 'isoechoic, homogeneous enhancement' patterns.
A, B, C. Vascular phase pulse-inversion harmonic US images obtained 24 sec (A), 38 sec (B) and 56 sec (C) after contrast injection depict peripheral nodular enhancement with progressive centripetal fill-in (arrows).
D. Delayed phase image (high MI) demonstrates isoechoic, homogeneous enhancement (arrowheads), which is the same appearance as normal hepatic parenchyma.
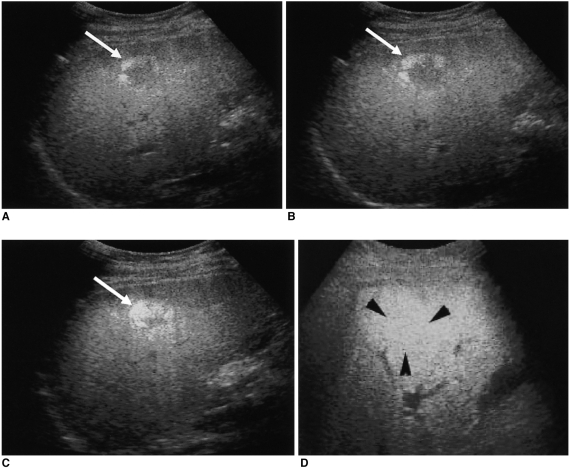
Fig. 6
Metastasis from stomach cancer, exhibiting the 'hypoechoic, decreased enhancement' pattern on delayed phase image.
A. Pulse-inversion harmonic US image obtained prior to contrast injection depicts a hypoechoic nodule (arrow).
B. Delayed phase image (high MI) shows a hypoechoic mass with decreased enhancement (arrow) compared to homogenous bright echogenic hepatic parenchyma.
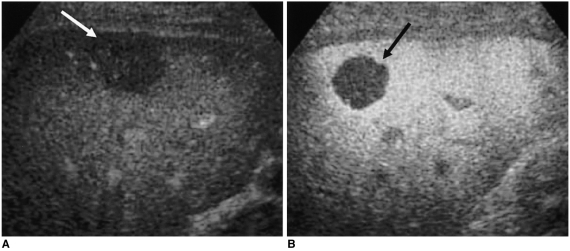
Fig. 7
Hemangioma demonstrating 'hypoechoic and reversed echogenicity' at the delayed phase.
A. Conventional sonogram depicts a hyperechoic hepatic nodule (arrow).
B. Delayed phase pulse-inversion harmonic image reveals a hypoechoic nodule with decreased enhancement compared to adjacent liver (arrow), and hypoechoic and reversed echogenicity at stimulated acoustic emission imaging.
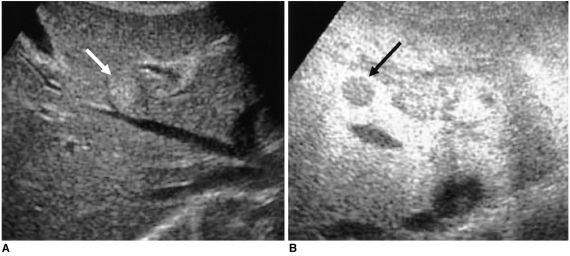
Table 1
Enhancement Patterns for Tumoral Vascularity at Vascular Phase Pulse-inversion Harmonic US in 90 Patients with HCC, Metastasis, and Hemangioma
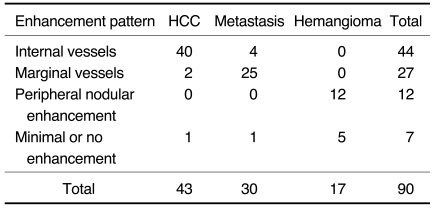
Table 2
Vascular Phase Enhancement Patterns for Each Diagnosis, and the Corresponding Sensitivity, Specificity, and PPV Values
