This article has been retracted. See "Notice of Redundant Publication" in Volume 8 on page 184.
Abstract
Objective
To determine, using proton magnetic resonance spectroscopy (1H MRS) whether thalamotomy in patients with Parkinson's disease gives rise to significant changes in regional brain metabolism.
Materials and Methods
Fifteen patients each underwent stereotactic thalamotomy for the control of medically refractory parkinsonian tremor. Single-voxel 1H MRS was performed on a 1.5T unit using a STEAM sequence (TR/TM/TE, 2000/14/20 msec), and spectra were obtained from substantia nigra, thalamus and putamen areas, with volumes of interest of 7-8ml, before and after thalamotomy. NAA/Cho, NAA/Cr and Cho/Cr metabolite ratios were calculated from relative peak area measurements, and any changes were recorded and assessed.
Results
In the substantia nigra and thalamus, NAA/Cho ratios were generally low. In the substantia nigra of 80% of patients (12/15) who showed clinical improvement, decreased NAA/Cho ratios were observed in selected voxels after thalamic surgery (p < 0.05). In the thalamus of 67% of such patients (10/15), significant decreases were also noted (p < 0.05).
Conclusion
Our results suggest that the NAA/Cho ratio may be a valuable criterion for the evaluation of Parkinson's disease patients who show clinical improvement following surgery. By highlighting variations in this ratio, 1H MRS may help lead to a better understanding of the pathophysiologic processes occurring in those with Parkinson's disease.
Parkinson's disease is an irreversible and progressive neurodegenerative disease characterized by resting tremor, rigidity, bradykinesia, and postural instability (1-3). In recent years, increasing interest has been shown in the stereotactic neurosurgical treatment (radiofrequency thalamotomy or pallidotomy) of the condition (4-9), and in the post-levodopa era, stereotactic thalamotomy has been reconsidered as a viable alternative in the treatment of parkinsonian tremor (10, 11). The pathophysiology of thalamic ablation for the relief of Parkinsonism is, however, not completely understood, and little information about the changes in regional brain metabolism occurring after thalamotomy in Parkinson's disease is available.
In-vivo proton magnetic resonance spectroscopy (1H MRS) is a noninvasive technique that allows in-vivo assessment of brain tissue composition and metabolic processes in Parkinson's disease (12-14). The potential for obtaining good spatial localization and high-resolution serial measurements makes in-vivo 1H MRS an ideal method for investigating biochemical interactions such as the mechanism of neurotransmitters (15). This application introduces the possibility of observing major brain metabolites that contribute to the major resonances seen in 1H MR spectra of the brain, such as N-acetylaspartate (NAA), creatine/phosphocreatine (Cr), and choline-containing compounds (Cho). Biochemical information about local cellular metabolism can be obtained by determining the metabolite ratios of spectrally detected neurochemicals (16), and may provide important clues to the pathophysiologic status of patients with Parkinson's disease.
Clinical improvement of Parkinsonian tremor after thalamotomy might be reflected by the changes in brain metabolism seen at 1H MRS. Functional connectivity between the thalamus and other motor-related regions such as the basal ganglia and substantia nigra might also be reflected by the changes in brain metabolism occurring after thalamotomy.
The purpose of this study is to investigate the utility of 1H MRS in demonstrating changes in regional brain metabolism after stereotactic thalamotomy in patients with Parkinson's disease.
Between August 1998 and September 1999, 15 patients (seven men and eight women; mean age, 56.5 years) underwent stereotactic thalamotomy for the control of medically refractory parkinsonian tremor. Prior to the procedure, all received levodopa, but this failed to control their tremors. Three showed no response to the drug, while the initially good response of the other 12 subsequently declined. Nine patients with predominantly right-sided symptoms, and six whose symptoms were predominantly left-sided underwent, respectively, thalamotomy of the left, and right, ventralis lateralis. Clinical assessment of all 15 by a neurologist specializing in movement disorders included neurologic examination and assignment of a score from the four-point Tremor Rating Scale (17, 18): 0, absent; 1, slight, present with action; 2, moderate in amplitude, present with action; 3, moderate in amplitude, with posture holding and action; 4, marked in amplitude, interferes with feeding. This quantitative assessment was performed preoperatively and about 4 months postoperatively. The clinical characteristics of these 15 patients, who underwent unilateral thalamotomy, appear in Table 1.
For the purpose of comparison, 15 age-matched control subjects [six men and nine women aged 39-64 (mean, 54.8) years], none of whom showed clinical evidence of neurologic diseases or MR imaging evidence of structural lesions in the basal ganglia, also underwent MR imaging and 1H MRS. Written informed consent was obtained from all patients and control subjects.
An MRI-compatible stereotactic frame was affixed to the cranial vault after infiltration of the pin insertion sites with 1% lidocaine. Following frame placement, the patient underwent sagittal T1-weighted MR imaging to identify the anterior and posterior commissure (AC, PC) and measure AC-PC length. Next, T1-weighted axial images (TR, 400; TE, 12; FOV, 30×30; 2 NEX; 3 mm thickness) were obtained through the basal ganglia and thalamus, parallel to the AC-PC plane. In most cases the AC-PC line was visible, and could be used for identification of the initial target, located at a point about 4-5 mm posterior to the mid AC-PC plane, 13.5-15 mm lateral to the midline, and 0-1 mm above the level of the intercommissural plane. When the third ventricle is dilated, it is wise to add 1-2 mm to the lateral dimension.
The patient was placed in a comfortable position and the frame was fixed to the operating table with the head slightly above the level of the chest. After infiltration of the scalp with 1% lidocaine, a 2-3 cm parasagittal incision was made and a burr hole was placed 2.5 cm from the midline at the level of the coronal suture. The dura was coagulated and opened and a small pial incision was made, avoiding any cortical veins, to allow for atraumatic introduction of the electrode. At this point the stereotactic arc was brought into position and the electrode guide tube lowered into the burr hole directly over the pial incision. Macrostimulation can also be used to delineate the optimal lesion location. A commercially available lesion generator (Radionics, Burlington, Mass., U.S.A.) was used for impedance monitoring, stimulation and lesion induction. Stimulation involved the use of square wave pulses at 2-3 volts and with a frequency of 5 Hz to obtain motor thresholds, and at 50 Hz to assess the amelioration of sensory response symptoms. Tremor response indicated whether the electrode was well positioned: low frequency (2 Hz) stimulation within Vim usually causes tremor increase, whereas high frequency (50 Hz) stimulation, resulting from good positioning, causes amelioration. Once the target was confirmed, a lesion was generated for 2 minutes at 65℃, and for 2 minutes at 75℃, and tremor reduction was substantial. After withdrawing the generator to a point 1mm from the target, another lesion was induced for 2 minutes at 75℃, and the procedure was repeated with the generator 3 mm from target. After induction of this final lesion, the tremor totally disappeared.
For in-vivo 1H MRS examinations before and after stereotactic thalamotomy, a clinical 1.5T MRI/MRS system (GE Signa Advantage, Version 4.8; GE Medical Systems, Milwaukee, Wis., U.S.A.) with a standard quadrature birdcage head coil was used. Localized single voxels (7-8 ml) centered on the volume of interest regions in the substantia nigra, thalamus, and putamen were selected using T2-weighted MR imaging (TR, 2500 ms; TE, 90 ms). The average follow-up period was four months (range, 14-20 weeks). A stimulated-echo acquisition mode (STEAM) (19, 20) was used for localization; suppression of the water signal involved the use of a three-pulse chemical shift selective (CHESS) sequence (21, 22). For optimization of the homogeneitiy of both total and localized brain volume, higher order offsets and linear shim coils were adjusted by auto prescan (APS), which was also used to adjust the strength of transmitter RF power, receiver gains, and the three RF pulses used for suppression of the water signal. After adjustment, typical line width (full width at half maximum, FWHM) was usually 2-4 Hz, which provided 97-99% of the suppression factor. Image-guided STEAM spectra were obtained with a TE of 20 msec, TR of 2000 msec, data points of 2048, spectral bandwidth of 2500 Hz, and acquisition averages of 128.
Raw data were transferred to a Sun SPARC Workstation IPC (Sun Micro Systems, Mountain View, Cal., U.S.A.) and processed using a SAGE data analysis package (GE Medical Systems, Milwaukee, Wis., U.S.A.). Postprocessing involved the removal of residual water signal, correction, if needed, of the heavy eddy current, Lorenz-to-Gauss transformation, Gaussian line broadening of 0.5 Hz, zerofilling of 4K, Fourier transformation, and zero-order phasing of the transformed spectrum. Any resulting spectra which contained the features of inadequate signal-to-noise ratio (SNR), outer volume contamination, distorted baselines, severe phase distortion due to the heavy eddy current, hardware artifacts, or inappropriate suppression of the water signal were regarded as unacceptable and excluded from data analysis. Peak areas were derived using the Marquardt algorithm to fit a Lorenzian type (23). Resonance peak assignments of major neurometabolites at in-vivo 1H MRS were NAA at 2.00 ppm, Cr at 3.00 ppm, and Cho at 3.20 ppm. In all patients and all control subjects, the relative ratios of NAA to Cho, NAA to Cr and Cho to Cr in the substantia nigra, thalamus and putamen were determined before and after stereotactic thalamotomy. We assessed whether clinical improvement of Parkinsonian tremor after thalamotomy was reflected by the changes in brain metabolism seen at 1H MRS, also comparing these ratios between control subjects and patients.
All patients with tremor experienced amelioration of their symptoms on the side contralateral to the thalamotomy. Before treatment, three patients scored 2 on the Tremor Rating Scale, ten had a score of 3, and two had a score of 4; four months after treatment, 13 patients scored 0 and two scored 1 (Table 1). Complete abolition of tremor occurred in 87% of patients (13/15), with significant improvement in the remaining two.
During the initial postoperative period of about one week, each patient underwent T2-weighted MR imaging, and temporary neurological deficits, presumably due to perilesional edema, were observed in all cases. The deficits resolved as the edema resolved, and were absent at four-month follow-up examination (Fig. 1B). Typical T2-weighted axial MR images, including voxels of the substantia nigra, thalamus and putamen for localized in-vivo 1H MRS after thalamotomy, are shown in Figure 1. In-vivo 1H MR spectra were also obtained from the voxels of the substantia nigra, thalamus and putamen, on the most affected side, before and after thalamotomy (Fig. 2). NAA/Cho ratios for the substantia nigra, thalamus and putamen are listed in Table 2, and compared graphically in Figure 3. As can be seen in Figures 3A and 3B, the differences between them are substantial. In the substantia nigra of 80% of patients (12/15) who showed clinical improvement following thalamotomy, decreased NAA/Cho ratios were observed at selected voxels, and significant metabolic changes in these ratios were apparent after thalamic surgery (p = 0.03). In 67% of patients (10/15) whose clinical condition improved, these ratios were also significantly lower in the thalamus (p = 0.03). In the putamen, significant statistical change in the ratios was not established (p = 0.08), though a significant reduction was noted in 73% of patients (11/15). Thus, in these 15 cases, NAA/Cho ratios in the substantia nigra, thalamus and putamen were generally low following thalamotomy. No significant changes in other metabolite ratios (NAA/Cr and Cho/Cr) were established. Although the mean ratios appear to indicate lower NAA/Cho for patients than for age-matched controls, t tests showed that these differences were not statistically significant.
Neuroimaging modalities such as computed tomography (CT) and MRI are of limited value in distinguishing the neurodegenerative causes of Parkinson's disease and, as in our study, morphologic abnormalities are often not apparent (Fig. 1). In Parkinson's disease, the presence of iron (or other paramagnetic) deposition in the putamen may cause hypointensity at T2-weighted MR imaging, though this is nonspecific or indistinguishable from the hypointensity seen in many normal elderly subjects (24, 25).
The results reported in this paper indicate that tremor relief in chronic levodopa-refractory Parkinson's patients can be reliably achieved by means of stereotactic thalamotomy. Tremor was abolished in 13 of our 15 patients, with significant improvement in the other two (Table 2), and overall, 87% of patients showed moderate to marked improvement after a mean follow-up period of four months. These results are consistent with those reported by others during the pre-carbidopa/levodopa era (26-29) and provide additional support for the use of thalamotomy for the treatment of medically refractory tremor. Thalamotomy had no effect, however, on bradykinesia or other manifestations of Parkinson's disease such as mental deterioration and gait disturbance.
In-vivo 1H MRS is a noninvasive method of measuring important proton metabolites in patients with Parkinson's disease, and several MRS investigations of the brain in patients with this condition have been reported (12-14). All compared metabolic rates between normal controls and patients with Parkinson's disease, and most found that in those with the disease, differences in the NAA/Cho ratio were not significant. The studies did not, however, examine the relationship between regional metabolic changes and to clinical outcome following surgery. To our knowledge, this is the first study to use 1H MRS for the follow-up evaluation of Parkinson's disease patients who showed clinical improvement following surgery. Using this technique, we have demonstrated that NAA/Cho ratios showed generally low levels in the substantia nigra, thalamus and putamen of such patients.
Compared with pre-thalamotomy values, the observed changes in NAA/Cho ratios in these patients were substantial (Fig. 3). Among those who underwent thalamotomy, decreased ratios were observed in the substantia nigra and thalamus of 80% (12/15) and 67% (10/15), respectively. It was not statistically established that surgery led to significant change in the ratio, though the reduction observed tended to be significant in 73% of patients (11/15).
The results of this study indicate that significant postoperative metabolic reduction of NAA/Cho ratios occurred in portions of the substantia nigra, thalamus and putamen of the hemisphere that did undergo surgery. Metabolic change occurred, in other words, in Parkinson's disease patients whose clinical condition improved. Table 2 shows that these changes were consistent in the substantia nigra, thalamus and putamen contralateral to the most affected side. The surgical changes induced by thalamotomy may directly affect the specific regional brain networks associated with clinical manifestations of parkinsonism.
Loss of dopaminergic inputs to the striatum associated with degeneration of the nigrostriatal pathway decreases the activity of inhibitory striatal neurons (predominantly D2 receptors) and increases the activity of others (predominantly D1 receptors). The former neurons project directly to the globus pallidus interna, and the latter mainly to the globus pallidus externa and subthalamic nucleus. The mechanism of action of thalamotomy is not known but may involve the destruction of autonomous neural activity, that is, of synchronous bursts that fire at the same frequency as limb tremor. Lenz et al. (30) reported that a small lesion which targets tremor cells (neurons that fire at tremor frequency) is uniformly effective in relieving parkinsonian tremor. Two major pathways are interrupted by a thalamic lesion: the pallidofugal, which arises from the globus pallidus interna and enters the inferior part of the ventralis oralis nucleus via the ansa lenticularis, and the contralateral pathway, which arises from the cerebellum. The ventralis intermedius (Vim) projects to the motor cortex, and as a result of the connections involved could become passively entrained by oscillations throughout the motor system. Thus, lesions in the Vim would reduce tremor by curtailing this entrainment; thalamotomy plays this role, interrupting the passive entrainment of Vim by distant structures, and thus its inherent rhythmicity. Hence, we suppose that the pathophysiologic mechanisms generated in the selected regions may be directly related.
Considerable effort has been invested in attempting to elucidate the role of NAA in cerebral metabolism (31, 32). Although its exact function is still unknown, there is strong evidence, especially from investigations of human brain tumors (33, 34), tumor extracts (35), and studies of extracts from cultured cells (36), to suggest that this compound is limited solely to neurons. Furthermore, it has been shown that lesions produced by specific neurotoxic agents lead to reductions in NAA (37). NAA has therefore been used as a marker of neuronal integrity, and it has been suggested that the NAA reductions seen at proton MRS are secondary to neuronal loss in cerebral pathology as exemplified by stroke (38), acquired immunodeficiency syndrome (AIDS) (39), multiple sclerosis (40, 41), demyelinating diseases (39), and diabetes mellitus (42). NAA reductions attributed to neuronal degeneration have been further verified by a large database derived from using temporal lobe epilepsy (44), AIDS (45), and Alzheimer's disease cases (46).
Our analysis of the combined data from all patients showed a decreased NAA/Cho ratio in the substantia nigra and thalamus of Parkinson's disease patients. Although we feel that in this study the ratio of NAA/Cho peak area measurements reflects a significant decrease in NAA itself, we acknowledge the possibility that increased Cho levels also account for the decreased NAA/Cho ratios. A reduced NAA/Cho ratio may, in other words, be interpreted as either decreased NAA, reflecting neuronal loss, or increased Cho, presumably reflecting gliosis (47, 48). A further interpretation is that both NAA and Cho are affected early in the surgical process, and it is this combination that allows the significant difference to be detected. Our present result suggests that since changes in the NAA/Cho ratio may be related to clinical improvement following surgery, the ratio may be a valuable criterion for the evaluation of Parkinson's disease. Because significant metabolic change was demonstrated in patients with Parkinson's disease, 1H MRS may be a more sensitive diagnostic tool than MRI in monitoring the progressive change occurring in such patients.
Additional analysis of the data presented in Table 2 showed a trend toward slightly decreased NAA/Cho and NAA/Cr ratios in the putamen, whereas Cho/Cr was within the normal range. These findings may indicate slightly decreased NAA and unaffected Cho and Cr levels, or alternatively, they may indicate that increased Cho and Cr levels lead to decreased NAA/Cho and NAA/Cr ratios, and a normal Cho/Cr ratio. Absolute quantification of the metabolites involved would resolve this question. For the present, however, we cannot exclude the possibility that neuronal reduction in the putamen of PD patients might be sufficient for MRS examination to reveal significantly decreased NAA levels.
Although thalamotomy has gained renewed acceptance as a treatment for advanced Parkinsonism, the pathophysiology of improved motor function following surgery is not fully understood. However, the present study has shown that 1H MRS centered on the substantia nigra, thalamus and putamen in carefully selected Parkinson's disease patients reveals a consistent, reproducible, and significant reduction in the NAA/Cho ratio, which is most likely to reflect changes in NAA itself. Decreased NAA/Cho ratios may reflect neuronal loss, and we suggest that thalamic ablation may have metabolic consequences for beyond the lesion site. Moreover, postsurgical clinical improvement may in part be predicated by these metabolic changes, which although remote, may be critical determinants of therapeutic success.
Bowen et al. (49) reported that in patients with Parkinson's disease, the lactate signal was elevated, a finding which might support the hypothesis that mitochondrial electron transport chain deficits and a concomitant increase in free radical generation due to defects in oxidative phosphorylation are present in brain tissue in Parkinson's disease, and that associated lactate elevation is a sign of metabolic stress (50). Neither before nor after surgery, however, were we able to demonstrate a lactate signal in substantia nigra, thalamus, or putamen tissue in either controls or Parkinson's disease patients, and this has also been the finding of other studies (12, 51-53).
In conclusion, the present study suggests that by highlighting metabolic variation, particularly that of the NAA/Cho ratio, water-suppressed in-vivo 1H MRS may help provide a better understanding of the pathophysiologic process occurring in patients with Parkinson's disease. Indeed, an understanding of the functional basis for surgical response may have great importance in the design of new treatment strategies for Parkinson's disease and related disorders. Investigation of the MR spectral alterations seen at after a long-term follow-up examination in patients with Parkinson's disease can provide the basis for the further detailed analysis required.
Figures and Tables
Fig. 1
Typical T2-weighted axial MR images obtained approximately 4 months after stereotactic thalamotomy show no apparent morphologic abnormalities. Voxels of (A) substantia nigra, (B) thalamus, and (C) putamen of the hemisphere that did undergo surgery were selected for localized in-vivo 1H magnetic resonance spectroscopy.
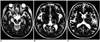
Fig. 2
In-vivo 1H magnetic resonance spectra obtained before and after thalamotomy from selected voxels of (A) substantia nigra, (B) thalamus, and (C) putamen in patients with Parkinson's disease.
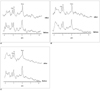
Fig. 3
Comparison of NAA/Cho ratios obtained before and after thalamotomy from selected voxels of (A) substantia nigra, (B) thalamus, and (C) putamen in patients with Parkinson's disease. The results indicate significant postoperative metabolic reduction in NAA/Cho ratios in the substantia nigra, thalamus, and putamen of the hemisphere that did undergo surgery.
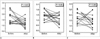
Table 1
Clinical Data of Patients with Parkinson's Disease Who Underwent Unilateral Stereotactic Thalamotomy
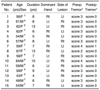
Acknowledgments
The authors wish to thank Sang-Mook Lee, Ho-Gun Lee, Seuing-Hoon Jeong, and Chun-Dal Son (Kangnam St. Mary's Hospital, Seoul, Korea) for their technical support and treatment of patients. We also thank the staff, residents, interns, and graduate students of the Catholic University Medical Center for their voluntary participation.
References
1. Barbeau A. Six years of high-level levodopa therapy in severely akinetic parkinsonian patients. Arch Neurol. 1976. 33:333–338.
2. Boshes B, Arbit J, Dolkart M, et al. The sixth year after L-dopa: quality of life and insights derived. Trans Am Neurol Assoc. 1975. 100:166–168.
3. Delaney P, Fermaglich J. Parkinsonism and levodopa: a five-year experience. J Clin Pharmacol. 1976. 16:652–659.
4. Jankovic J, Cardoso F, Grossman RG, Hamilton WJ. Outcome after stereotactic thalamotomy for parkinsonian, essential, and other types of tremor. Neurosurgery. 1995. 37:680–686.
5. Lang AE, Lozano AM, Montgomery E, Duff J, Tasker R, Hutchinson W. Posteroventral medial pallidotomy in advanced Parkinson's disease. N Engl J Med. 1997. 337:1036–1042.
6. Samuel M, Ceballos-Baumann AO, Turjanski N, et al. Pallidotomy in Parkinson's disease increases supplementary motor area and prefrontal activation during performance of volitional movements on H215O PET study. Brain. 1997. 120:1301–1313.
7. Davis KD, Taub E, Houle S, et al. Globus pallidus stimulation activates the cortical motor system during allevation of parkinsonian symptoms. Nat Med. 1997. 3:671–674.
8. Limousin P, Greene J, Pollak D, Rothwell J, Benabid AL, Frakowiak R. Changes in cerebral activity pattern due to subthalamic nucleus or internal pallidum stimulation in Parkinson's disease. Ann Neurol. 1997. 42:283–291.
9. Dogali M, Fazzini E, Kolodny E, et al. Stereotactic ventral pallidotomy for Parkinson's disease. Neurology. 1995. 45:753–761.
10. Burchiel KJ. Thalamotomy for movement disorders. Neurosurg Clin N Am. 1995. 6:55–71.
11. Fox MW, Ahlskog JE, Kelly PJ. Stereotactic ventrolateralis thalamotomy for medically tremor in post-levodopa era Parkinson's disease. Neurosurgery. 1995. 36:1118–1127.
12. Holshouser BA, Komu M, Moller HE, et al. Localized proton NMR spectroscopy in the striatum of patients with idiopathic Parkinson's disease: a multicentre pilot study. Magn Reson Med. 1995. 33:589–594.
13. Ellis CM, Lemmens G, Williams SC, et al. Changes in putamen N-acetylaspartate and choline ratios in untreated and levodopa-treated Parkinson's disease: a proton magnetic resonance spectroscopy study. Neurology. 1997. 49:438–444.
14. Choe BY, Park JW, Lee KS, et al. Neuronal laterality in Parkinson's disease with unilateral symptom by in-vivo 1H MR spectroscopy. Invest Radiol. 1998. 33:450–455.
15. Bottomley PA. Spatial localization in NMR spectroscopy in-vivo. Ann NY Acad Sci. 1987. 508:333–348.
16. Hughes A, Daniel S, Kilford L, Lees A. Accuracy of clinical diagnosis of idiopathic Parkinsion's disease: a clinicopathological study of 100 cases. J Neurol Neurosurg Psychiatry. 1992. 55:181–184.
17. Fahn S, Marsden CD, Calne DB, Goldstein M, editors. Recent development in Parkinson's disease. 1987. Vol 2. Florham Park, NJ: Macmillan Health Care Information;153–163.
18. The Parkinson Study Group. Low-dose clozapine for the treatment of drug-induced psychosis in Parkinson's disease. N Engl J Med. 1999. 340:757–763.
19. Frahm J, Merbold KD, Hanicke W. Localized proton spectroscopy using stimulated echoes. J Magn Reson. 1987. 72:502–508.
20. Moonen CTW, van Zijl PCM. Highly effective water suppression for in-vivo proton NMR spectroscopy (DRY STEAM). J Magn Reson. 1990. 88:28–41.
21. Hasse A, Frahm J, Hanicke W, Mattaei D. 1H NMR chemical shift selective (CHESS) imaging. Phys Med Biol. 1985. 30:341.
22. Doddrell DM, Galloway G, Brooks W, et al. Water signal elimination in-vivo using suppression by mistimed echo and repetitive gradient episodes. J Magn Reson. 1986. 70:176–180.
23. Kreis R, Farrow N, Ross BD. Localized 1H NMR spectroscopy in patients with chronic hepatic encephalopathy: analysis of change in cerebral glutamate, choline, and inositols. NMR in Biomed. 1994. 4:109–116.
24. Drayer BP, Olanow W, Burger P, et al. Parkinson-plus syndrome: diagnosis using high-field MR imaging of brain iron. Radiology. 1989. 159:493–498.
25. Stern MB, Braffman BH, Skolnick BE, et al. Magnetic resonance imaging in Parkinson's disease and Parkinsonian syndromes. Neurology. 1989. 39:1524–1526.
26. Benabid AL, Pollak P, Gao D, et al. Chronic electrical stimulation of the ventralis intermedius nucleus of the thalamus as a treatment of movement disorders. J Neurosurg. 1996. 84:203–214.
27. Goldman MS, Ahlskog E, Kelly P. The symptomatic and functional outcome of stereotactic thalamotomy for medically intractable essential tremor. J Neurosurg. 1992. 76:924–928.
28. Jankovic J, Cardoso F, Grossman R, et al. Outcome after stereotactic thalamotomy for Parkinsonian, essential, and other types of tremor. Neurosurgery. 1995. 37:680–687.
29. Nagaseki Y, Shibazaki T, Hirai T, et al. Long-term follow-up results of selective VIM-thalamotomy. J Neurosurg. 1986. 65:296–302.
30. Lenz FA, Normand SL, Kwan HC, et al. Statistical prediction of the optimal site for thalamotomy in parkinsonian tremor. Mov Disord. 1995. 10:318–328.
31. Birken DL, Oldendorf WH. N-acetyl-aspartate acid: a literature review of a compound prominent in 1H NMR spectroscopic studies of the brain. Neurosci Biobehav Rev. 1989. 13:23–31.
32. Miller BL. A review of chemical issues in 1H NMR spectroscopy: N-acetyl-L-aspartate, creatine and choline. NMR Biomed. 1991. 4:47–52.
33. Bruhn H, Frahm J, Gyngell ML, et al. Noninvasive differentiation of tumors with use of localized 1H MR spectroscopy in vivo: initial experience in patients with cerebral tumors. Radiology. 1989. 172:541–548.
34. Langkowski JH, Wieland J, Bomsdorf H, et al. Pre-operative localized in-vivo proton spectroscopy in cerebral tumors at 4.0 Tesla-first results. Magn Reson Imaging. 1989. 7:547–555.
35. Gill SS, Thomas DG, Van Bruggen N, et al. Proton MR spectroscopy of intracranial tumors: in-vivo and in-vitro studies. J Comput Assist Tomogr. 1990. 14:497–504.
36. Gill SS, Thomas DG, Thomas DG, et al. Brain metabolites as 1H NMR markers of neuronal and glial disorders. NMR Biomed. 1989. 2:196–200.
37. Koller KJ, Zaczek R, Coyle JT. N-acetyl-aspartyl-glutamate: regional levels in rat brain and the effects of brain lesions as determined by a new HPLC method. J Neurochem. 1984. 43:1136–1142.
38. Bruhn H, Frahm J, Gyngell ML, Merboldt KD, Hanicke W, Sauter R. Cerebral metabolism in man after acute stroke: new observations using localized proton NMR spectroscopy. Magn Reson Med. 1989. 9:126–131.
39. Menon DK, Baudouin CJ, Tomlinson D, Hoyle C. Proton MR spectroscopy and imaging of the brain in AIDS: evidence of neuronal loss in regions that appear normal with imaging. J Comput Assist Tomogr. 1990. 14:882–885.
40. Van Hecke P, Marchal G, Johannik K, et al. Human brain proton localized NMR spectroscopy in multiple sclerosis. Magn Reson Med. 1991. 18:199–206.
41. Matthews PM, Francis G, Antel J, Arnold DL. Proton magnetic resonance spectroscopy for metabolic characterization of plaques in multiple sclerosis. Neurology. 1991. 41:1251–1256.
42. Kreis R, Ross BD. Cerebral metabolic disturbances in patients with subacute and chronic diabetes mellitus: detection with proton MR spectroscopy. Radiology. 1992. 184:123–130.
43. Ende GR, Kaxer KD, Knowlton RC, et al. Temporal lobe epilepsy: bilateral hippocampal metabolic changes revealed at proton MR spectroscopic imaging. Radiology. 1997. 202(3):809–817.
44. Chang KH, Kim HD, Park SW, et al. Usefulness of single voxel proton MR spectroscopy in the evaluation of hippocampal sclerosis. Korean J Radiol. 2000. 1:25–32.
45. Lu D, Pavlakis SG, Frank Y, et al. Proton MR spectroscopy of the basal ganglia in healthy children and children with AIDS. Radiology. 1996. 199:423–428.
46. Shonk TK, Moats RA, Gifford P, et al. Probable Alzheimer disease: diagnosis with proton MR spectroscopy. Radiology. 1995. 195:65–72.
47. Connelly A, Jackson GD, Duncan JS, King MD, Gadian DG. Magnetic resonance spectroscopy in temporal lobe epilepsy. Neurology. 1994. 44:1411–1417.
48. Matthews PM, Andermann F, Arnold DL. A proton magnetic resonance spectroscopy study of focal epilepsy in humans. Neurology. 1990. 40:985–989.
49. Brown BC, Block RE, Sanchez-Ramos J, et al. Proton MR spectroscopy of the brain in vivo in 14 patients with Parkinson's disease. Am J Neuroradiol. 1995. 16:61–68.
50. Beal MF. Does impairment of energy metabolism result in excitotoxic neuronal death in neurodegenerative illness? Ann Neurol. 1992. 31:119–130.
51. Federico F, Simone IL, Lucivero V, et al. Localized proton NMR spectroscopy in Parkinson's disease and progressive supranuclear palsy. J Neurol Neurosurg Psychiatry. 1997. 62:239–242.
52. Clarke CE, Lowy M, Horsman A. Unchanged basal ganglia N-acetylaspartate and glutamate in idiopathic Parkinson's disease measured by proton magnetic resonance spectroscopy. Mov Disord. 1997. 12:297–301.
53. Davie CA, Wenning GK, Barker GJ, et al. Differentiation of multiple system atrophy from idiopathic Parkinson's disease using proton magnetic resonance spectroscopy. Ann Neurol. 1995. 37:204–210.