Abstract
Objective
To assess the utility of multiphasic perfusion CT in the prediction of final infarct volume, and the relationship between lesion volume revealed by CT imaging and clinical outcome in acute ischemic stroke patients who have not undergone thrombolytic therapy.
Materials and Methods
Thirty-five patients underwent multiphasic perfusion CT within six hours of stroke onset. After baseline unenhanced helical CT scanning, contrast-enhanced CT scans were obtained 20, 34, 48, and 62 secs after the injection of 90 mL contrast medium at a rate of 3 mL/sec. CT peak and total perfusion maps were obtained from serial CT images, and the initial lesion volumes revealed by CT were compared with final infarct volumes and clinical scores.
Results
Overall, the lesion volumes seen on CT peak perfusion maps correlated most strongly with final infarct volumes (R2=0.819, p<0.001, slope of regression line=1.016), but individual data showed that they were less than final infarct volume in 31.4% of patients. In those who showed early clinical improvement (n=6), final infarct volume tended to be overestimated by CT peak perfusion mapping and only on total perfusion maps was there significant correlation between lesion volume and final infarct volume (R2=0.854, p=0.008). The lesion volumes depicted by CT maps showed moderate correlation with baseline clinical scores and clinical outcomes (R=0.445-0.706, p≤0.007).
One of the essential roles of stroke imaging is to reveal the ischemic penumbra that is the target of thrombolytic therapy. For optimal qualitative assessment of reversible ischemic tissue (ischemic penumbra), stroke imaging should be able to accurately predict the extent of final infarct volume, and initial images should depict the extent of irreversible damage.
Even though perfusion MR imaging has been more widely used, helical CT techniques providing coverage of all or most of the brain have recently been employed for perfusion CT imaging, demonstrating their potential utility in the detection of arterial occlusion, the assessment of cerebral perfusion, and the prediction of final infarct volume and clinical outcome (1-8). Although perfusion CT offers faster scanning, a greater availability of scanners, and relatively easier data processing than perfusion MR imaging, existing clinical data pertaining to the utility of perfusion CT in the prediction of final infarction, and clinical symptoms and outcomes, are limited. A recent study (8) of patients who underwent intraarterial thrombolytic therapy suggested that the mismatch between unenhanced and perfusion-weighted CT lesion volumes might reflect ischemic penumbra. To establish the role of perfusion CT in guiding thrombolytic therapy beyond unenhanced CT scanning in acute ischemic stroke, further studies may be required.
The most appropriate method for the imaging of ischemic penumbra has not yet been fully established, and difficulty in this regard is also encountered at MR imaging in acute ischemic stroke. Though it has been assumed that the mismatch between diffusion-weighted and perfusion MR imaging is due to ischemic penumbra, a hyperintense or decreased ADC lesion, seen at diffusion-weighted imaging, contains reversible ischemic tissue (9, 10), and in patients with proximal arterial occlusion, lesions which demonstrate perfusion abnormality at qualitative perfusion MR imaging may include an oligemic area as well as ischemic penumbra (11-14).
For the assessment of patients with acute ischemic stroke, we used a modified multiphasic perfusion CT protocol based on a triphasic helical CT technique (4), retrospectively evaluating multiphasic perfusion CT images and testing the a hypothesis that in patients with acute middle cerebral artery (MCA) stroke who have not undergone thrombolytic therapy, multiphasic perfusion CT is predictive of final infarct volume, baseline clinical score, and clinical outcome.
Thirty-five consecutive patients [29 men, 17 women; age range, 31-88 (mean, 64) years] who experienced MCA territory stroke symptoms between October 1998 and January 2001 were enrolled in this study. Informed consent was obtained from each patient or their family before CT imaging, and the study protocol was approved by our institution's review board.
Inclusion criteria were: (a) symptom onset, as identified by the patient or other person, was less than six hours before CT scanning; (b) the National Institutes of Health Stroke Scale (NIHSS) score was 4 or more; (c) unenhanced CT revealed no hemorrhage; (d) post-CT treatment did not include thrombolytic therapy or the use of a neuroprotective agent; (e) follow-up unenhanced CT or MR images were obtained within 2-10 days of initial CT studies, and were regarded as indicative of final infarct volume.
Multiphasic perfusion CT images were obtained using a helical CT scanner (High-speed Advantage; GE Medical Systems, Milwaukee, Wis., U.S.A.) according to a modified protocol based on a triphasic helical CT technique (4). Following the acquisition of baseline conventional unenhanced CT images of the whole brain, contrast-enhanced scans of eight slice sections were acquired five times at each of four phases (20, 34, 48, and 62 secs) after the power injection of 90 mL non-ionic contrast agent at a rate of 3 mL/sec. Except for the lower portion of the posterior fossa and vertex, CT scans covered most of the brain. All unenhanced and enhanced CT images were obtained at 10-mm collimation and 10-mm/sec table speed, and were reconstructed at 5-mm intervals. The scanning parameters were 120 kilovolts and 240 mA (conventional unenhanced CT) or 200 mA (helical CT), with a 512×512 image matrix and a 23-cm field of view.
From the serial images obtained during the five phases, two CT perfusion maps were retrospectively generated. Prior to data processing for CT mapping of all patients, possible head movement was corrected, using the semiautomated registration technique (15). Bony calvarium only, rather than whole brain pixels, was coregistered, and the time required for this process was thus shortened. First, a peak perfusion CT map was generated on a pixel-by-pixel basis by simple subtraction of registered, baseline, unenhanced helical CT images from the enhanced images obtained during peak enhancement of the contralateral hemisphere. In the resultant images, voxel intensity is produced by a change in Hounsfield units (ΔHU) proportional to the concentration of contrast agent within a voxel of tissue. Second, a total perfusion CT map was generated on a pixel-by-pixel basis after calculating the area under the time-attenuation curve by subtracting unenhanced helical CT from contrast-enhanced CT images obtained during the four phases of enhanced CT scanning. Two CT maps were generated off-line at a personal computer workstation after the transfer there of raw CT data. The total scanning time for the completion of acquisitions and reconstruction of images was less than five minutes, and about five minutes were required for the processing of CT map data.
Quantitative analysis involved measurement of the lesion volumes seen at initial CT and follow-up MR or CT imaging. After imaging data were transferred to a personal computer, lesion volumes were measured using a commercially available software program (ALICE; Hayden Imaging Processing Solutions, Boulder, Col., U.S.A.). Lesion volumes were measured manually by one rater blinded, except for information as to which hemisphere was affected, to the results of counterpart CT or MR imaging, and follow-up imaging. In order to avoid bias, the volume of each lesion was initially measured on each CT image and then on follow-up images after at least 2 weeks. After the area of a lesion was measured at each eight-slice section, integration and multiplication of slice thickness was used to calculate lesion volume. Before one rater measured this for each image, lesion outlines were visually determined by two raters, who reached a consensus after reviewing the hypoattenuated parenchymal areas seen at conventional unenhanced CT and areas of decreased density on CT peak or total perfusion maps of multiphasic perfusion CT. At follow-up imaging, the lesion volume demonstrated by fluid-attenuated inversion recovery MRI (n=22) or unenhanced CT imaging (n=13) was determined.
Correlation between the initial lesion volume seen on each CT image and final infarct volume at follow-up imaging was analyzed overall and for each subgroup, and either clinical improvement within 24 hours of symptom onset (n=6), or later than this (n=29) was recorded. Mismatched lesion volume between the findings of unenhanced CT and CT peak perfusion maps was compared with the volume of infarct growth determined as final infarct volume minus initial lesion volume as seen at unenhanced CT.
For comparison of individual lesion volumes between initial and follow-up images, and in view of possible errors in manual measurements, a difference was considered significant if greater than 20% of volume and more than 3 cm3. Measured lesion volumes at initial CT or as seen on map images were compared with those obtained at follow-up imaging, and the prediction of final infarct volume on the basis of initial CT or MR imaging findings was categorized as 'overestimation' (more than 120 % of the lesion volume seen at follow-up imaging), 'concordant' (80%-120%), or 'underestimation' (less than 80%).
For each patient, NIHSS scores were recorded by experienced neurologists at baseline, and 24 hours, 3 days, 7 days, and 1 month after onset of symptoms. Since they were in many cases unavailable, an analysis of NIHSS scores at 3 months was not included in this study. The lesion volumes seen at unenhanced CT, and on CT peak and total perfusion maps were compared with clinical scores.
Using linear regression analysis, initial lesion volumes were correlated with the final infarct volumes seen at follow-up imaging. Pearson correlation coefficients and coefficients of determination (R2) were used to represent the relationship between each lesion volume and to estimate the proportion of variation in the dependent variable. To compare the overall tendency of each lesion volume determined at CT imaging, the slope of regression line fitted by a forced zero intercept were determined. To compare the initial lesion volume seen at CT imaging with the NIHSS score, Spearman's rank correlation test was used.
The mean period between symptom onset and multiphasic perfusion CT was 3.0 ± 1.7 (range, 0.5 to 6) hours, and the mean time interval before follow-up imaging was 4.8 ± 2.6 (range, 2 to 10) days.
Table 1 shows the relationship between initial lesion volumes at CT imaging and follow-up infarct volumes; scatter-plots of each data set are shown in Figure 1. Overall, there was significant correlation between initial lesion volume and final infarct volume, as seen at unenhanced CT and on CT peak and total perfusion maps. The initial lesion volume seen on CT peak perfusion maps correlated most strongly with final infarct volume measured at either follow-up unenhanced CT or MRI (R2=0.819, p<0.001) and tended to be similar to final infarct volume (slope of regression line, 1.016) (Fig. 2). Meanwhile, final infarct volumes were significantly underestimated by total perfusion mapping and unenhanced CT (slope of regression lines, 1.246 and 1.523, respectively), as demonstrated by Figure 3. The mismatch between lesion volume at unenhanced CT and on CT peak perfusion maps correlated significantly with the volume of infarct growth (R=0.756, R2=0.572, p<0.001), showing, overall, a mean value slightly less than this (43.89 ± 42.01 vs. 50.45 ± 39.38, respectively) but slightly more than final infarct volume (slope of regression line=0.942).
In the subgroup (n=6) which showed early spontaneous improvement in the NIHSS score (> 4) at 24 hours after symptom onset, initial lesion volumes on CT peak perfusion maps were greater than final infarct volumes (30.73 ± 24.67 vs. 16.71 ± 20.37), though the difference was statistically insignificant (p=0.226). Meanwhile, in this subgroup, the initial lesion volume seen on CT total perfusion maps was 15.06 ± 15.31 cm3, and final infarct volume correlated strongly only with the lesion volumes on CT total perfusion maps (R2=0.854, p=0.008). In the subgroup (n=29) which showed no early clinical improvement, final infarct volumes were slightly underestimated on CT peak perfusion maps (R2=0.819, p<0.001, slope of regression line=1.027), and more significantly underestimated on total perfusion maps (R2=0.785, p<0.001, slope of regression line=1.247).
The results of analysis of individual data are shown in Table 2. Although there is linearity between the initial lesion volumes seen on peak perfusion maps and final infarct volumes, the latter were underestimated in 11 (31.4%) patients and severely underestimated as less than 50% of actual final infarct volume in five (14.3%). Final infarct volumes were underestimated on CT total perfusion maps and at unenhanced CT in 68.5% and 85.7% of cases, respectively.
Table 3 shows the relationship between initial lesion volume at CT and NIHSS score. Correlation between initial lesion volume at unenhanced CT and baseline NIHSS score or clinical outcome converted into follow-up NIHSS score at days 3, 7 and 30 was only modest (R=0.362-0.482), while that between initial lesion volume at CT peak or total perfusion mapping and baseline NIHSS score or clinical outcome (R=0.445-0.706) was moderate. Between peak or total perfusion mapping and clinical outcome, correlation was relatively stronger at the acute or subacute stage (3 or 7 days) than at the chronic stage (30 days). The relationship between baseline NIHSS score and final infarct volume at follow-up unenhanced CT or MRI was similar to that between this score and the volume seen at initial CT peak or total perfusion mapping, but clinical outcomes converted into follow-up NIHSS score at days 3, 7, and 30 showed slightly stronger correlation with final rather than initial infarct volume.
Because the contrast agent used is continuously injected for 30 seconds, the multiphasic perfusion CT technique does not permit a first pass of contrast agent. Instead, a relatively constant period of peak tissue enhancement is maintained. During this period of peak enhancement, the concentration of contrast agent in intravascular tissue space within a voxel is fairly constant (4), and the CT peak depicted by a perfusion map may be an image of cerebral perfusion determined by cerebral blood volume and cerebral blood flow (3). If delivery of the contrast agent is significantly delayed by low cerebral blood flow in any area, peak perfusion maps will demonstrate low intensity in that area, even though cerebral blood volume is, in fact, high, and this may explain the mismatch between CT peak and total perfusion maps (Fig. 3).
A CT total perfusion map obtained by integration of the area under a time-attenuation curve depicts the total perfused blood volume in a voxel within the period of scanning. On a map of this kind, some areas of a hypoperfused lesion seen on a CT peak perfusion map appear to be normal (Fig. 3), suggesting that while a peak perfusion map depicts perfused blood volume due to rapid effective collateral flow, a total perfusion map depicts the total perfused volume of both slow and rapid collateral flow. Thus, it may be speculated that if a lesion appears on both total and peak perfusion maps, conversion to infarction will be more rapid and there will be more significant vasogenic edema (Fig. 2) than where a lesion appears only on a peak perfusion map (Fig. 3). By reference to peak and total perfusion maps of multiphasic perfusion CT, both the degree of hypoperfusion in tissue and the extent of infarction may be qualitatively predicted.
If imaging is used for the qualitative assessment of cerebral ischemia, the extent of infarct growth, determined according to the difference between final infarct volume at follow-up and the volume of irreversibly damaged tissue seen at initial imaging, may be considered indicative of the extent of ischemic penumbra. Our data for untreated patients showed that on peak perfusion maps, lesion volume was equal to or less than that of the final infarct in 91.5% of patients, suggesting that if early arterial recanalization does not occur, a hypoperfused lesion seen on a CT peak perfusion map is, at follow up, highly specific for infarction. Our data also suggest that with regard to lesion volume, the observed mismatch between a CT peak perfusion map and unenhanced CT is predictive of the extent of infarct growth. The mismatched volume was, however, slightly smaller than the volume of infarct growth, and there was only moderate correlation between initial lesion volume at CT peak perfusion mapping and baseline clinical scores. Thus, the mismatch between a CT peak perfusion map and unenhanced CT may underestimate the true extent of ischemic penumbra. The parenchymal hypodensity seen at unenhanced CT is known to be highly specific for irreversible ischemic tissue (16), but whether this hypodensity accurately represents the extent of such damage has not been definitely established. Although CT peak perfusion maps and unenhanced CT may, respectively, tend to underestimate final infarct volume and the extent of irreversible tissue damage, we suggest that ischemic penumbra may be approximately estimated by the extent of mismatch between a hypoperfused lesion seen at CT peak perfusion mapping and a slightly and low attenuated lesion seen at unenhanced CT.
Our data showed that baseline clinical score correlated more closely with lesion volume at CT peak or total perfusion mapping than with lesion volume at unenhanced CT, which is associated with the severity of clinical symptoms. Patel et al. (17) reported that initial lesion volume at unenhanced CT correlated with baseline NIHSS score and a favorable outcome at three months, and Mark et al. (18) stated that at unenhanced CT, a larger lesion was associated with a higher baseline NIHSS score. The stronger relationship between lesion volume, as revealed by CT peak perfusion mapping, and baseline clinical score suggests that prior to tissue change, as cerebral blood flow decreases below a threshold of cortical dysfunction (20 mL/100 g/min), a hypoperfused lesion seen at CT peak perfusion mapping has a closer relationship with the area of cortical dysfunction. The observed significant correlation between lesion volume at CT total perfusion mapping and baseline clinical score also suggests that severe hypoperfusion is associated with the severity of clinical symptoms. Although correlation between lesion volume on a CT peak or total perfusion map and baseline or acute clinical scores was only moderate, CT maps appear no less predictive of the severity of clinical symptoms than diffusion or perfusion MR imaging. Recent studies (11, 19-21) of stroke MR imaging showed that for various relationships between the acute NIHSS score and lesion volume at diffusion-weighted (r=0.31-0.71) or qualitative perfusion MR imaging (r=0.31-0.96), the data was inconsistent.
It may be speculated that because a large mismatch at multiphasic perfusion CT suggests the possible presence of extensive ischemic penumbra, salvageable by early recanalization, thrombolytic therapy may be of more benefit to patients whose lesion findings show a mismatch between CT peak perfusion mapping and unenhanced CT, than those with no mismatch. In patients with hyperacute ischemic stroke, a mismatch between unenhanced CT and peak perfusion mapping, seen at on multiphasic perfusion CT, might be able to provide useful information for the guidance of thrombolytic therapy. To determine the clinical value of mismatch at multiphasic perfusion CT, further investigation is needed, and our study involving acute ischemic stroke patients who have undergone thrombolytic therapy is, in fact, in progress.
The drawbacks of our CT method include the noisy image quality of CT maps, the possibility of contrast agent-induced complications, and a lack of quantitative information about cerebral perfusion. A small ischemic lesion may not be detected at peak perfusion CT mapping, and because the perfusion of white matter is relatively low (less than half that of gray matter), a mildly hypoperfused white matter lesion may not be apparent on CT maps. Because, in most patients, follow-up scans were obtained during acute-stage ischemia, the size of a lesion thus depicted may not correspond to the true size of the infarct; this may in some cases be overestimated due to brain edema or swelling (22), or underestimated because the infarct continues to grow (23).
In conclusion, initial lesion volume at CT peak perfusion mapping showed strong correlation with final infarct volume, was highly specific for final infarction, and accurately predicted final infarct volume in about two-thirds of the 35 patients. A mismatch between lesion volume at CT peak or total perfusion mapping and at unenhanced CT was predictive of lesion growth. Clinical outcome correlated more strongly, though still only moderately, with lesion volume at CT peak or total perfusion mapping than with lesion volume at unenhanced CT. Multiphasic perfusion CT is useful for prediction of the initial severity of clinical symptoms, the extent of final infarction, and clinical outcomes.
Figures and Tables
Fig. 1
Overall correlation between initial lesion volume and final infarct at unenhanced CT, and on CT peak and total perfusion maps. The relationship between initial lesion and final infarct volume was strongest on CT peak perfusion maps (R2=0.819), followed by CT total perfusion maps (R2=0.797) and unenhanced CT (R2=0.641). CT peak perfusion maps showed that initial lesion volume was slightly less than final infarct volume (slope of regression line=1.016), while CT total perfusion maps and unenhanced CT showed it as significantly less (slope of regression line=1.246 and 1.523, respectively).
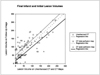
Fig. 2
Correspondence between lesion volume at CT peak perfusion mapping and final infarct volume in a 31-year-old female who underwent multiphasic perfusion CT five hours after the onset of stroke symptoms. Unenhanced CT (A) shows diffuse parenchymal hypodensity and loss of cortex/white matter distinction (arrows) in left MCA territory. Lesion size at unenhanced CT is similar to that of the hypoperfused lesion (arrows) seen on CT peak (B) and total perfusion maps (C), indicating no mismatch between these two maps or between unenhanced CT and CT maps. Follow-up CT images (D) obtained 3 days after initial CT show a significant mass effect and infarction edema in the left hemisphere. Seven days after the onset of stroke, the NIHSS score was slightly aggravated (increase of 4).
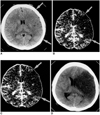
Fig. 3
Overestimation of lesion volume on CT peak perfusion map in a 75-year-old female who underwent multiphasic perfusion CT 2 1/2 hours after the onset of stroke symptoms. Unenhanced CT (A) reveals no parenchymal hypodensity. The CT peak perfusion map (B), though not the total perfusion map (C), depicts a diffuse hypoperfused lesion (arrows) in left MCA territory, suggesting abundant delayed collateral flow. This case shows significant mismatch between the CT peak perfusion map and unenhanced CT, as well as between CT peak perfusion and total perfusion maps. Follow-up CT (D) performed two days after initial CT, shows little mass effect of the ischemic lesion and a smaller infarction than the hypoperfused lesion seen on the CT peak perfusion map. Seven days after the onset of stroke, the NIHSS score showed no significant change (decrease of 1).
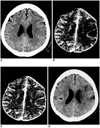
Table 1
Correlation between Lesion Volumes at Initial Multiphasic Perfusion CT and Follow-Up Imaging (n=35)

References
1. Shrier DA, Tanaka H, Numaguchi Y, Konno S, Patel U, Shibata D. CT angiography in the evaluation of acute stroke. Am J Neuroradiol. 1997. 18:1011–1020.
2. Knauth M, von Kummer R, Jansen O, Hahnel S, Dorfler A, Sartor K. Potential of CT angiography in acute ischemic stroke. Am J Neuroradiol. 1997. 18:1001–1010.
3. Hunter GJ, Hamberg LM, Ponzo JA, et al. Assessment of cerebral perfusion and arterial anatomy in hyperacute stroke with three-dimensional functional CT: early clinical results. Am J Neuroradiol. 1998. 19:29–37.
4. Na DG, Byun HS, Lee KH, et al. Acute occlusion of the middle cerebral artery: early evaluation with triphasic helical CT-preliminary results. Radiology. 1998. 207:113–122.
5. Lee KH, Cho SJ, Byun HS, et al. Triphasic perfusion computed tomography in acute middle cerebral artery stroke: correlation with angiographic findings. Arch Neurol. 2000. 57:990–999.
6. Lee KH, Lee SJ, Cho SJ, et al. Usefulness of triphasic perfusion computed tomography for intravenous thrombolysis with tissue-type plasminogen activator in acute ischemic stroke. Arch Neurol. 2000. 57:1000–1008.
7. Lev MH, Farkas J, Rodriguez VR, et al. CT angiography in the rapid triage of patients with hyperacute stroke to intraarterial thrombolysis: accuracy in the detection of large vessel thrombus. J Comput Assist Tomogr. 2001. 25:520–528.
8. Lev MH, Segal AZ, Farkas J, et al. Utility of perfusion-weighted CT imaging in acute middle cerebral artery stroke treated with intra-arterial thrombolysis: prediction of final infarct volume and clinical outcome. Stroke. 2001. 32:2021–2028.
9. Kidwell CS, Saver JL, Mattiello J, et al. Thrombolytic reversal of acute human cerebral ischemic injury shown by diffusion/perfusion magnetic resonance imaging. Ann Neurol. 2000. 47:462–469.
10. Fiehler J, Foth M, Kucinski T, et al. Severe ADC decreases do not predict irreversible tissue damage in humans. Stroke. 2002. 33:79–86.
11. Barber PA, Darby DG, Desmond PM, et al. Prediction of stroke outcome with echoplanar perfusion- and diffusion-weighted MRI. Neurology. 1998. 51:418–426.
12. Neumann-Haefelin T, Wittsack HJ, et al. Diffusion- and perfusion-weighted MRI: influence of severe carotid artery stenosis on the DWI/PWI mismatch in acute stroke. Stroke. 2000. 31:1311–1317.
13. Rohl L, Ostergaard L, Simonsen CZ, et al. Viability thresholds of ischemic penumbra of hyperacute stroke defined by perfusion-weighted MRI and apparent diffusion coefficient. Stroke. 2001. 32:1140–1146.
14. Grandin CB, Duprez TP, Smith AM, et al. Usefulness of magnetic resonance-derived quantitative measurements of cerebral blood flow and volume in prediction of infarct growth in hyperacute stroke. Stroke. 2001. 32:1147–1153.
15. Woods RP, Grafton ST, Holmes CJ, Cherry SR, Mazziotta JC. Automated image registration: I. General methods and intrasubject, intramodality validation. J Comput Assist Tomogr. 1998. 22:139–152.
16. von Kummer R, Bourquain H, Bastianello S, et al. Early prediction of irreversible brain damage after ischemic stroke at CT. Radiology. 2001. 219:95–100.
17. Patel SC, Levine SR, Tilley BC, et al. National Institute of Neurological Disorders and Stroke rt-PA Stroke Study Group. Lack of clinical significance of early ischemic changes on computed tomography in acute stroke. JAMA. 2001. 286:2830–2838.
18. Marks MP, Holmgren EB, Fox AJ, Patel S, von Kummer R, Froehlich J. Evaluation of early computed tomographic findings in acute ischemic stroke. Stroke. 1999. 30:389–392.
19. Lovblad KO, Baird AE, Schlaug G, et al. Ischemic lesion volumes in acute stroke by diffusion-weighted magnetic resonance imaging correlated with clinical outcome. Ann Neurol. 1997. 42:164–170.
20. Tong DC, Yenari MA, Albers GW, O'Brien M, Marks MP, Moseley ME. Correlation of perfusion- and diffusion-weighted MR in patients with NIHSS score in acute (<6.5 hour) ischemic stroke. Neurology. 1998. 50:864–870.
21. Schellinger PD, Fiebach JB, Jansen O, et al. Stroke magnetic resonance imaging within 6 hours after onset of hyperacute cerebral ischemia. Ann Neurol. 2001. 49:460–469.
22. Beaulieu C, de Crespigny A, Tong DC, Moseley ME, Albers GW, Marks MP. Longitudinal magnetic resonance imaging study of perfusion and diffusion in stroke: evolution of lesion volume and correlation with clinical outcome. Ann Neurol. 1999. 46:568–578.
23. Pantano P, Caramia F, Bozzao L, Dieler C, von Kummer R. Delayed increase in infarct volume after cerebral ischemia: correlations with thrombolytic treatment and clinical outcome. Stroke. 1999. 30:502–507.