Abstract
Nonketotic hyperglycinemia (NKH) is a rare metabolic brain disease caused by deficient activity of the glycine cleveage system. Localized proton MR spectroscopy (echo-time 166 msec), performed in an infant with the typical clinical and biochemical features of neonatal NKH, showed a markedly increased peak intensity at 3.55 ppm, which was assigned to glycine. Serial proton MR spectrosocpic studies indicated that glycine/choline and glycine/total creatine ratios correlated closely with the patient's clinical course. Proton MR spectroscopy was useful for the non-invasive detection and monitoring of cerebral glycine levels in this infant with NKH.
Glycine is the simplest of the amino acids but is known to have important cerebral functions, acting as both a neurotransmitter and neuromodulator (1, 2). Although a number of other metabolic fates are possible for glycine, the major catabolic pathway is through the glycine cleavage system, a complex enzyme system found within the inner mitochondrial membrane of the liver, kidney, and brain
Nonketotic hyperglycinemia (NKH) is an autosomal recessive disorder caused by a deficienct glycine cleavage system, and generally results in elevated glycine levels in urine, blood, and cerebrospinal fluid (CSF). Such increases are harmless in blood but can cause extensive neuronal damage in neonatal brain, via N-methyl-D-aspartate glutamate receptor-mediated exitotoxicity (2). Infants with NKH are usually symptomatic within the first 48 hours, and after initial myoclonic or generalized seizure, rapid progression to coma occurs, often with apnea. Most patient die during the neonatal period, despite intensive care.
A search of the literature brought to light only three reports describing the application of proton magnetic resonance spectroscopy (MRS) to NKH (3-5). Each stated that long echo-time MRS detected a prominent peak at 3.5 ppm, which was assigned to glycine. In this case report, we describe our experience of MRS in an infant with NKH.
A male baby, delivered normally and spontaneously after a full-term pregnancy, was well during the first 28 hours of life. He then became lethargic and hypotonic, and the shallow respiration which began at that point progressed to intermittent apnea, requiring mechanical ventilation. On the 4th day, brain computed tomography and ultrasonography were performed, to exclude the possibility of hemorrhage in the posterior cranial fossa: the findings were normal. On the 5th day, amino acid analysis of the CSF and plasma was requested, to exclude the possibility of metabolic disorders, and on the 6th day, brain magnetic resonance (MR) imaging and spectroscopy were performed using a 1.5-tesla system (GE Signa, Milwaukee, Wis., U.S.A.). Again, the imaging findings were normal.
Using a single-voxel stimulated-echo acquisition mode (STEAM) sequence (repetition time / echo time / mixing time, 3000 / 166 / 13.7; number of excitations, 96; spectral width 2500 Hz; number of points, 2048; voxel size, 2×2×2 cm), proton MR spectra of the right parietal white matter were obtained. The raw data were post-processed by water-referenced correction of the eddy current effect, Lorenz-to-Gauss transformation, Gaussian line broadening of 0.5 Hz, zerofilling of 8K, Fourier transformation, and zero-order phasing of the transformed spectra. Major spectral peaks occurring at 2.01, 3.03, 3.22 and 3.55 ppm were assigned to N-acetylaspartate (NAA), creatine and phosphocreatine (total Cr), choline-containing compounds (Cho), and glycine, respectively (3, 6). The spectra obtained were compared with those from an age-matched control subject (Fig. 1). The patient's MR imaging findings were normal (Fig. 2A), but his spectrum revealed that the peak intensity, observed at 3.55 ppm, had increased markedly, and this was assigned to glycine. Glycine/Cho and glycine/total Cr ratios were found to be 1.58 and 2.42, respectively (Fig. 2B).
CSF and plasma amino acid analysis revealed glycine levels of 184 (normal, < 13) nmol/mL and 1411 (normal, 232-740) nmol/mL, respectively. The CSF: plasma glycine concentration ratio was 0.13 (normal, < 0.02). The results of amino acid analysis and other laboratory tests indicated NKH, and on the basis of this diagnosis, the patient was treated with dextromethorphan (8.5 mg/kg/day) and sodium benzoate (300 mg/kg/day, intravenous infusion), followed by a double-volume exchange transfusion, peritoneal dialysis and total parenteral amino acid-free nutrition. After intensive medical treatment, his respiration and consciousness level improved slowly over several days, and on the 12th day, follow-up amino acid analysis revealed decreased CSF and plasma glycine concentrations of 69 and 346 nmol/mL, respectively. On the same day, follow-up MRS of the same parietal white matter showed decreased glycine/Cho (1.05) and glycine/total Cr (1.31) ratios, in accordance with decreased CSF and plasma glycine concentrations (Fig. 2C).
After amino acid (0.5 g/kg/day) was added to the parenterally administered nutritional mix, the patient again developed seizure-like motion and intermittent apnea. Over several days, he developed intractable generalized tonic-clonic seizure and his clinical condition deteriorated rapidly, requiring mechanical ventilation. Final follow-up MRS on the 17th day revealed, in comparison with the second MRS study, a prominently increased glycine/Cho ratio (1.80), a slightly increased glycine/total Cr ratio (1.60) and a newly occorring lactate, at 1.3 ppm (Fig. 2D). He expired on the 20th day.
NKH is a rare metabolic brain disease, though is well known to neonatologists and pediatricians. In more than 80% of NKH patients, the condition presents during the neonatal period. Because most patients die at this time, there have been very few neuro-imaging studies. Press et al. (7) reported that in seven patients with NKH, MR imaging studies revealed progressive atrophy, abnormal thinning of the corpus callosum, and decreased or absent myelination in the supratentorial white matter. These morphologic changes can be observed in patients who survived the neonatal period, and may be a late sequela of previous metabolic brain damage. In the acute phase of this disease, routine brain MR imaging findings are usually normal, despite serious clinical symptoms.
MRS has, during the last decade, been applied extensively to various kinds of metabolic brain diseases, in the hope of detecting any specific abnormal metabolites. In the majority of such diseases, however, the findings have been non-specific, especially from a diagnostic point of view (8). NKH is one of the few metabolic brain diseases in which MRS can detect a specific metabolite that accumulates in the brain. In our case, using long echo-time (166 msec) STEAM MRS, we observed that glycine concentration increased, peaking at 3.55 ppm (Fig. 2B-D). Because absolute quantification of metabolite concentrations was not feasible, we used glycine/Cho and glycine/total Cr ratios, as determined at serial MRS, to estimate cerebral glycine levels. After intensive medical treatment and some symptomatic improvement, glycine/Cho decreased from 1.58 to 1.05, in accordance with a decrease in CSF glycine from 184 to 69 nmol/mL. After symptomatic aggravation, glycine/Cho increased again, from 1.05 to 1.80, reflecting close correlation between glycine/Cho levels and the clinical status of the patient. The glycine/total Cr ratio tended to be similar to that of glycine/Cho, and in this respect, Heindel et al. (3) reported good correlation between glycine/Cho, glycine/total Cr, the glycine concentration estimated by MRS, and clinical findings. Suzuki et al. (4) reported that the time course of glycine/total Cr corresponded to changes in plasma and CSF glycine levels, and Gabis et al. (5) reported that glycine levels measured by MRS corresponded to changes in plasma glycine levels. Our results are similar to those of previous reports and we agree with the opinion that MRS can be used as a non-invasive diagnostic or monitoring tool in patients with NKH. The increased lactate peak observed in the final spectrum (Fig. 2D) may reflect anaerobic glycolysis of brain tissue, probably caused by respiratory difficulty and hypoxia. In our case, it is difficult to draw definite conclusions regarding changes in NAA, total Cr, and Cho levels. In order to clarify changes in cerebral metabolites occurring in NKH, absolute quantification of their concentrations may be necessary.
The clinical outcome of neonatal NKH may depend on the severity of the involved enzyme defect. Most neonatal forms of the disorder are usually fatal despite intensive medical treatment, but in some transient forms, the outcome of appropriate treatment is generally excellent (9). These milder or atypical forms of NKH usually present a greater diagnostic challenge (10), however, and close monitoring of their clinical course and glycine levels may be necessary even after the condition is diagnosed. In this situation, MRS can be used as a non-invasive diagnostic or monitoring tool.
In summary, our case shows the typical clinical, laboratory and MRS features of neonatal NKH. The most characteristic feature of MRS was a marked increase in peak glycine intensity, to 3.55 ppm, in the spectra obtained using a long echo-time. We believe that in patients with NKH, MRS not only provides diagnostic information but is also a suitable noninvasive monitoring tool.
Figures and Tables
Fig. 1
A control spectrum of stimulated-echo acquisition mode (STEAM) sequence (TR/TE, 3000/166) obtained at the parietal white matter of a one-month-old infant with neonatal seizure, for comparison. NAA, N-acetylaspartate; tCr, total creatine; Cho, choline-containing compounds
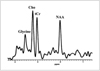
Fig. 2
Proton MR imaging and spectra obtained from an infant with nonketotic hyperglycinemia. All spectra are scaled individually and cannot be directly compared.
A. Axial T1-weighted (600/16) image obtained with a spin-echo sequence and 18-cm field of view, 256 × 192 matrix, and 5-mm contiguous sections, with the voxel location as indicated for proton MR spectroscopy.
B. A spectrum obtained from the parietal white matter shows a prominent singlet at 3.55 ppm (arrow), which is assigned to glycine. The peak intensity of glycine is much higher than that of Cho (glycine/Cho, 1.58) and of tCr (glycine/tCr, 2.42).
C. A follow-up spectrum obtained after treatment and symptomatic improvement shows a slightly decreased peak glycine intensity. The observed peak intensity is similar to that of Cho (glycine/Cho, 1.05) and slightly higher than that of tCr (glycine/tCr, 1.31).
D. A final spectrum, obtained after symptoms worsened, shows a markedly increased peak glycine intensity. The observed peak intensity is again much higher than that of Cho (glycine/Cho, 1.80) and of tCr (glycine/tCr, 1.60), and peak lactate intensity has increased to 1.33 ppm.
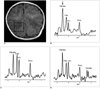
References
1. Probst A, Cortes R, Palacios JM. The distribution of glycine receptors in the human brain: a light microscopic autoradiographic study using [3H]strychinine. Neuroscience. 1986. 17:11–35.
2. Kemp JA, Leeson PD. The glycine binding site of the NMDA receptor: five years on. Trends Pharmacol Sci. 1993. 14:20–25.
3. Heindel W, Kugel H, Roth B. Noninvasive detection of increased glycine content by proton MR spectroscopy in the brains of two infants with non-ketotic hyperglycinemia. Am J Neuroradiol. 1993. 14:629–635.
4. Suzuki Y, Ueda H, Toribe Y. Proton MR spectroscopy of nonketotic hyperglycinemia. No To Hattatsu. 2001. 33:422–425.
5. Gabis L, Parton P, Roche P, Lenn N, Tudorica A, Huang W. In vivo 1H magnetic resonance spectroscopic measurement of brain glycine levels in nonketotic hyperglycinemia. J Neuroimaging. 2001. 11:209–211.
6. Michaelis T, Merboldt KD, Haenicke W, Gyngell ML, Bruhn H, Frahm J. On the identification of cerebral metabolites in localized 1H NMR spectra of human brain in vivo. NMR Biomed. 1991. 4:90–98.
7. Press GA, Barshop BA, Haas RH, Nyhan WL, Glass RF, Hesselink JR. Abnormalities of the brain in nonketotic hyperglycinemia: MR manifestations. Am J Neuroradiol. 1989. 10:315–321.
8. Frahm J, Hanefeld F. Bachelard HS, editor. Localized proton magnetic resonance spectroscopy of brain disorders in childhood. Magnetic resonance spectroscopy and imaging in neurochemistry. 1997. New York: Plenum Press;329–402.
9. Schiffmann R, Kaye EM, Willis JK 3rd, Africk D, Ampola M. Transient neonatal hyperglycinemia. Ann Neurol. 1989. 25:201–203.
10. Jackson AH, Applegarth DA, Toone JR, Kure S, Levy HL. Atypical nonketotic hyperglycinemia with normal cerebrospinal fluid to plasma glycine ratio. J Child Neurol. 1999. 14:464–467.