Abstract
Objective
To identify and evaluate the lateral border zone by comparing the size and distribution of the abnormal signal area demonstrated by MR imaging with the infarct area revealed by pathological examination in a reperfused myocardial infarction cat model.
Materials and Methods
In eight cats, the left anterior descending coronary artery was occluded for 90 minutes, and this was followed by 90 minutes of reperfusion. ECG-triggered breath-hold turbo spin-echo T2-weighted MR images were initially obtained along the short axis of the heart before the administration of contrast media. After the injection of Gadomer-17 and Gadophrin-2, contrast-enhanced T1-weighted MR images were obtained for three hours. The size of the abnormal signal area seen on each image was compared with that of the infarct area after TTC staining. To assess ultrastructural changes in the myocardium at the infarct area, lateral border zone and normal myocardium, electron microscopic examination was performed.
Results
The high signal area seen on T2-weighted images and the enhanced area seen on Gadomer-17-enhanced T1WI were larger than the enhanced area on Gadophrin-2-enhanced T1WI and the infarct area revealed by TTC staining; the difference was expressed as a percentage of the size of the total left ventricle mass (T2= 39.2%; Gadomer-17 =37.25% vs Gadophrin-2 = 29.6%; TTC staining = 28.2%; p < 0.05). The ultrastructural changes seen at the lateral border zone were compatible with reversible myocardial damage.
Because revascularization therapy can potentially salvage viable myocardium, the determination of myocardial viability in patients with myocardial infarction is important for therapeutic decision-making and the evaluation of prognosis. To date, various imaging modalities including thallium-201 single photon emission tomography (SPECT), coronary angiography (CAG), and left ventriculography have been applied for this purpose. These modalities have their limitations, however: thallium-SPECT lacks sufficient spatial resolution to accurately determine the extent of myocardial infarction, while CAG and left ventriculography do not always provide information on the degree of myocardial injury due to ischemia and are, in addition, invasive procedures (1, 2).
Magnetic resonance imaging (MRI), however, offers excellent tissue contrast and high spatial resolution, and is noninvasive. In fact, a large number of studies have already provided considerable information on the value of MRI in myocardial infarction, and in this respect the modality is expected to play an increasingly important role in the evaluation of patients with this condition. Several MR contrast agents have so far been introduced and used. A necrosis-avid MR contrast agent, bis-gadolinium mesoporphyrins (Gadophrin-2; Schering, Berlin, Germany) has a marked affinity for non-viable tissue components and has been proved capable of diagnosing irreversibly damaged myocardium (3-6); a new intravascular MR contrast agent, Gadomer-17, is being tested in animals. In comparison with extracellular agents such as Gd-DTPA, intravascular agents offer much greater T1 relaxivity, higher concentration within the blood pool, and reduced extravasation into the myocardium. Noh et al. recently suggested that Gadomer-17-enhanced MR imaging delineated both reversibly and irreversibly damaged myocardium (7).
The purpose of our study was to identify and evaluate the lateral border zone by comparing the size and distribution of the area with high signal or enhancement seen on T2-weighted and contrast-enhanced T1-weighted MR images with the infarct area revealed by pathological examination in a cat model of reperfused myocardial infarction.
Eight adult cats weighing between 2.9 and 4.4 (mean, 3.9) kg were used in this study. Each animal was anesthetized with 5% halothane administered through an endotracheal tube by a mechanical ventilator (Mini-7; Royal Medical, Seoul, Korea). Pancuronium bromide (Panslan; Reyon Pharmaceutical, Seoul, Korea) (0.6 mg/kg) was used for skeletal muscle relaxation. To monitor blood pressure and allow pulse-gated MR imaging, a femoral artery was cannulated, and for the administration of drugs and contrast agents, this procedure was applied to a femoral vein. After a left lateral thoracotomy along the fifth intercostal space, pericardiotomy was performed by means of a midline incision, and a pericardial cradle was prepared by attaching the margins of the dissected pericardium to the adjacent thoracic wall. The left anterior descending (LAD) coronary artery was isolated distal to the first diagonal branch, and a snare loop was made with 4-0 silk placed in a slender plastic tube. Occlusion or reperfusion of the LAD artery was achieved simply by fastening or releasing the snare loop. Obstruction of this artery was confirmed by observing changes in the color of the myocardium at risk during a preliminary test occlusion. In each cat the LAD artery was occluded for 90 minutes, and this was followed by 90 minutes of reperfusion.
Gd-DTPA has been widely used as a clinical MR contrast agent. After injection, it is rapidly distributed from the vascular compartment to the extracellular fluid space. In rats, the plasma half-life is approximately 12 minutes (8), and due to a rapidly changing blood concentration resulting from the large volume distributed, the utility of Gd-DTPA for the MR diagnosis of acute myocardial ischemia is therefore limited (9, 10, 11).
Gadomer-17, provided by Schering, is designed for prolonged intravascular retention and reduced transcapillary diffusion and has a molecular weight (MW) of 17453 g/mol. Noh et al. demonstrated that following the administration of Gadomer-17, the signal intensity of the enhanced area seen on T1-weighted images increased rapidly, and maximum enhancement was detected during a 40-60 minute period (7).
Gadophrin-2, a necrosis-avid contrast material, consists of mesoporphyrins linked to gadolinium, and its MW is 1697.25 g/mol. The methods by which it is synthesized, and its chemical structure, physiochemical properties and imaging behaviors have been previously described in detail (12).
MR imaging was performed on a 1.5T Magnetom VISION system (Siemens, Erlangen, Germany). For signal reception, a circularly polarized head array coil 27cm in diameter (Siemens, Erlangen, Germany) was used. During the imaging procedure, heart rates were kept between 140-170 beats/min. Electrocardiography-triggered breath-hold turbo spin-echo T2-weighted MR images were obtained along the short axis of the heart prior to the injection of contrast agents, and in order to obtain additional information as to myocardial status, images in the sagittal plane were also acquired.
The acquisition parameters for T2-weighted MR images were as follows: repetition time msec/ echo time msec of 400-600 (according to heart rate) / 82, echo train length of 33, acquisition time of 9-10 seconds, matrix size of 132×256, field of view of 210×280 mm2, and section thickness of 5 mm.
After acquiring the baseline image and intravenously injecting contrast medium, Gadomer-17-enhanced T1-weighted MR images were obtained dynamically for one hour. After visual comfirmation of complete washout of Gadomer-17 (3 hours after its injection), Gadophrin-2 was administered, and contrast-enhanced T1-weighted MR images were obtained for the next hour. Electrocardiography-triggered multisection T1-weighted spin-echo imaging was performed with the following imaging parameters: repetition time msec/ echo time msec of 300-500 (according to heart rate)/ 25, section thickness of 5 mm, field of view of 210×280 mm2, and one signal acquisition. All images were obtained along the short axis of the heart, and to provide additional confirmation of the signal enhancement of irreversibly damaged myocardium, images in the sagittal plane were also occasionally acquired.
After MR imaging studies were completed, each cat was sacrificed by intravenous injection of KCl solution. The heart was excised and cut into five or six consecutive slices, 5mm thick, in the same planes in which the MR images were obtained. The specimens were immersed in 1.5% TTC solution at 36℃ and stained for 15 minutes, and were then stored in 10% formalin solution for 12 hours. Areas of myocardium unstained by TTC were defined as irreversibly damaged. Photographs of TTC-stained specimens in the same planes, for which MR images were obtained along the short axis of the heart, were scanned into a computer (Macintosh; Apple Computer Inc., Cupertino, Cal., U.S.A.) to measure the size of the infarct area and of the total left ventricle mass using public domain image processing software (NIH Image 1.55; National Institutes of Health, Bethesda, Md., U.S.A.). Finally, the results were compared with those of MRI.
All MR images were independently analyzed by two experienced radiologists, and discrepancies were resolved by consensus. The size of the infarct area in the myocardium of the left ventricle was measured by outlining the high signal area seen on T2-weighted images and enhanced areas on Gadomer-17- and Gadophrin-2-enhanced T1-weighted images. The size of the infarct area was expressed as a percentage of the size of the total left ventricle, as revealed by MR imaging and TTC histochemical staining. The size of the enhanced areas seen on Gadomer-17 and Gadophrin-2-enhanced T1-weighted images, and of the high signal area on T2-weighted images, was compared with that of the infarct area disclosed by TTC histochemical staining. To determine statistical significance (defined as p < 0.05), a paired Student t test was used.
Electron microscopic examinations were performed on tissue taken from three areas. That from the infarct area was sampled from the centers of TTC-unstained areas; for the lateral border zone, tissue from the TTC-stained peripheral region adjacent to the TTC-unstained area (2 mm apart from that area) was sampled; and for normal myocardium, tissue was taken from the center of the TTC-stained area of the posterior wall. After collection, tissues were cut into 1-mm cubes and fixed in a 2.5% buffered glutaraldehyde solution for 12 to 16 hours followed by additional fixation in a solution of osmotic acid at 5℃ for 2 hours. The cubes were then dehydrated in graded alcohol at room temperature, passed through propylene oxide, and placed in a 1:1 mixture of propylene oxide and Epon 812 (Polyscience Inc.) for 12 to 16 hours. Sections approximately 0.5 µm thick were cut on an LKB ultramicrotome (Bromma, Sweden) using a diamond knife, and were mounted on a copper grid and stained with 4% aqueous uranyl acetate and lead citrate for examination with a transmission electron microscope (JEM-1200 EX II, Tokyo, Japan).
The electron microscopic criteria for irreversibly damaged myocardium were that all mitochondria were swollen, had disorganized cristae, and contained electron-dense deposits and contraction bands in addition to disrupted sarcolemmas. On the other hand, the ultrastructures of reversibly damaged myocardium showed mild edematous myocytes, increased sarcoplasmic space, a prominent I-band, and mild peripheral aggregation of nuclear chromatin without the features of irreversibly damaged myocardium (13).
The high signal area seen on T2-weighted images and the enhanced area on Gadomer-17-enhanced T1-weighted images were larger than the enhanced area on Gadophrin-2-enhanced T1-weighted images and the infarct area disclosed by TTC histochemical staining (T2= 39.2 %; Gadomer-17 =37.25 % vs Gadophrin-2 = 29.6 %; TTC staining = 28.2 %; p < 0.05). The size of the high signal area seen on T2-weighted images correlated closely with that of the enhanced area on Gadomer-17-enhanced T1-weighted images, and the size of the enhanced area on Gadophrin-2-enhanced T1-weighted images showed close correlation with that of the infarct area revealed by TTC histochemical staining (Figs. 1, 2).
Electron microscopic examination of tissue taken from the three areas showed virtually the same results in each cat. Ultrastructural changes in the infarct area indicated irreversibly damaged myocardium; in the lateral border zone, the features of reversibly damaged myocardium were observed but findings of irreversibly damaged myocardium were absent (Fig. 3).
In this study, we found that the high signal area seen on T2-weighted images and the enhanced area on Gadomer-17-enhanced T1-weighted images were larger than the enhanced area on Gadophrin-2-enhanced T1-weighted images and the infarct area revealed by TTC histochemical staining, with statistical significance. Electron microscopic examination showed that tissue taken from the TTC-stained peripheral region adjacent to the TTC-unstained area exhibited the features of reversibly damaged myocardium. In a cat model of reperfused myocardial infarction, identification of the lateral border zone is therefore possible, and by means of MR imaging we were able to determine the size and distribution of this zone.
It has been well documented that the coronary artery is the major source of ischemic heart disease: thrombotic occlusion of an epicardial coronary artery is usually the cause of acute myocardial infarction (14). The myocardium initiates anaerobic glycolysis within 10 seconds of occlusion of the coronary artery, with the accumulation of lactate and other metabolites (15). Acute myocardial infarction first begins in the subendocardium within 20-40 minutes of occlusion and spreads toward the subepicardium. This concept of infarct progression has been previously described and termed the wavefront of myocardial necrosis by Reimer at al. (16, 17). A part of the transmural progression of injury is related to the transmural gradient of collateral blood flow. Previous clinical and experimental studies have indicated that collateral blood flow following coronary occlusion is extremely poor in the subendocardial region and blood is shunted preferentially to the subepicardial zone. Furthermore, two other factors, greater systolic wall stress and oxygen consumption, can contribute to subendocardial ischemia. Previous studies involving dogs and humans have shown that the evolution of infarction is usually completed within six hours of coronary occlusion; if reperfusion therapy is instituted while viable myocardium is present, the infarct can therefore be confined to a smaller area. Another study has suggested, however, that further myocardial cell death due to reperfusion may occur after prolonged ischemia (reperfusion injury) (18). The reduction of infarct size by reperfusion results primarily from the salvage of myocardium in the subepicardial region of the ischemia. In cases associated with coronary artery stenosis, coronary collateral flow plays an important role in maintaining the viability of the myocardium and in the smaller and confined infarct area (19, 20).
The lateral border zone (peri-infarct area) is defined as the lateral area of reversibly injured myocardium adjacent to the core of the infarct and within the area supplied by the occluded artery. Studies of acute myocardial infarction (21, 22) have determined whether salvageable myocardium existed along the lateral border zone as well as in the transmural direction. They found that intermediate levels of collateral blood flow and biochemical derangement, as well as intermediate functional impairment of myocardium, occur in the lateral margins of an ischemic region. Such data have been interpreted to imply the existence of a lateral border zone where salvage of the myocardium may be possible. However, some studies have argued that the lateral border zone of acute myocardial infarction is limited to a narrow zone or does not exist as a quantitatively significant region (23, 24).
This controversy over the existence of such a zone of intermediate injury may have arisen because of a difference in research methods. In their analysis of biochemical and flow gradient, Yellon et al. reported that a quantitatively significant and spatially identifiable "border zone" region did not exist, a conclusion probably due to the tissue sampling technique they employed and differences in the ligation time of the coronary artery (23). On the other hand, others have reported that if necrosis-avid contrast agents were used, a lateral border zone was demonstrated by cardiac MR imaging.
Various MR sequences and techniques have been developed for the assessment of ischemic heart disease. Previous reports have indicated that non-enhanced T1-weighted MRI fails to distinguish between myocardial infarction and normal myocardium. Lim et al. (25) reported that breath-hold turbo spin-echo T2-weighted MRI can successfully detect acute myocardial infarction, providing excellent tissue contrast and high spatial resolution in a reasonably short scan time. In that study, segmental analysis of acute myocardial infarction showed a diagnostic concordance rate between T2-weighted MRI and rest thallium-SPECT of 95%. However, even optimal T2-weighted images revealed both reversibly and irreversibly injured myocardium (26, 27).
Many attempts have been made to use contrast-enhanced T1-weighted MRI for the evaluation of acute myocardial infarction. De Roos et al. (28) reported that if infarct size was estimated on the basis of Gd-DTPA-enhanced spin-echo images, the effect of reperfusion therapy on infarct size could be accurately assessed. Other researchers, however, have reported that the use of a contrast agent such as Gd-DTPA overestimated the extent of myocardial infarction by approximately 10-20 % (29-31). Using Gd-DTPA polylysine-enhanced MRI in a cat model of reperfused myocardial infarction, Choi et al. (32) investigated changes in the size and degree of signal enhancement during the evolution of myocardial infarction over a six-day period. After observing that during those six days the enhanced area became smaller, they concluded that the highly enhancing area seen during the acute stage of reperfused myocardial infarction included both an irreversibly damaged necrotic area and a reversibly damaged peri-infarct zone.
A necrosis-avid MR contrast agent, bis-gadolinium mesoporphyrins (Gadophrin-2; Schering, Berlin, Germany), has recently become available and this shows a marked affinity for non-viable tissue components. The mechanism of signal enhancement by the contrast material in irreversibly damaged myocardium is still not well understood, but it can be assumed to result from some kind of binding of the compound to the sites of denatured tissue components by means of reperfused coronary flow and progressive extravascular diffusion. Further studies to elucidate the mechanism of accumulation are needed. In an animal model with occlusive and reperfused myocardial infarction, Gadophrin-2-enhanced MR imaging has proved capable of distinguishing between irreversibly and reversibly injured myocardium, with strong, persistent signal enhancement of the infarct area (33, 34). In addition, another intravascular contrast agent, Gadomer-17 (Schering, Berlin, Germany), has recently been introduced. According to studies performed at this institution, the enhanced area seen on Gadomer-17-enhanced T1-weighted images was similar to the high signal area on T2-weighted images, and statistical analysis showed no significant difference between them. We therefore believe that the enhanced area seen on Gadomer-17-enhanced T1-weighted images probably included both infarct and peri-infarct areas. Consequently, direct comparison of the enhanced area revealed by contrast-enhanced MRI when Gadophrin-2 and Gadomer-17 are used may provide the means of distinguishing between irreversibly and reversibly injured myocardium.
For revascularization therapy to be successful, it is imperative to distinguish between viable and non-viable myocardium: only the former is likely to benefit from this therapy. In this current study, the statistical difference in size between the abnormal signal areas on MR images and the infarct areas revealed by TTC histochemical staining may represent the lateral border zone and thus suggest the extent of reversibly damaged myocardium.
Unfortunately, our study suffers from certain limitations. First of all, we did not consider the in-vivo interaction between Gadophrin-2 and Gadomer-17. A previous study (34) reported that maximal enhancement took place 40-60 minutes after the administration of Gadomer-17 but 1-3 hours after the administration of Gadophrin-2, and that with both agents gradual washout then occurred; we therefore expected that since the maximal enhancement time of these two agents differed, the effect would be minor or non-existent. Second, we did not quantitatively analyze the lateral border zone seen on MR images which suggested reversibly damaged myocardium. Thus, in order to better understand the clinical usefulness of Gadomer-17 and Gadophrin-2, and the interaction between them, and to quantitatively analyze the lateral border zone seen on MR images, further study may be required.
In conclusion, by means of MR imaging and pathologic correlation we were able to identify the lateral border zone in reperfused myocardial infarction in a cat model, and it may therefore be assumed that both the lateral and transmural border zone contain potentially salvageable myocardium. Contrast-enhanced MR imaging using Gadophrin-2 and Gadomer-17 is potentially useful for determining the size and distribution of the lateral border zone.
Figures and Tables
Fig. 1
Comparison between the abnormal signal areas depicted by MRI and the infarct area revealed by TTC staining.
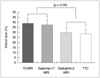
Fig. 2
MR images and the corresponding TTC-stained slice of reperfused myocardium in a cat model.
A. T2-weighted MRI shows high signal intensity in the territory of the left anterior descending (LAD) artery.
Gadomer-17(B) and Gadophrin-2(C)-enhanced T1-weighted images demonstrate enhancement in the corresponding area. The high signal intensity area on the T2-weighted image and the enhanced area on the Gadomer-17-enhanced T1-weighted image are significantly larger than the enhanced area on Gadophrin-2-enhanced T1-weighted images and the infarct area revealed by TTC staining (p < 0.05).
D. Macroscopic photograph of TTC-stained myocardial specimen taken at the same level as the MR images. The area of infarct corresponds to the non-stained area seen in the territory of the LAD artery.
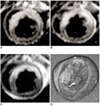
Fig. 3
The ultrastructural features of cat myocardium after 90 minutes of LAD occlusion followed by 90 minutes of reperfusion.
A. Ultrastructures of the infarct area. The sarcolemma is markedly disrupted (arrowheads), the mitochondria are swollen and contain electron-opaque dense granular bodies (arrows), and contraction bands (asterisk) are also seen.
B. Ultrastructure of the lateral border zone (peri-infarct area) shows interfiber and intramitochondrial edema, and prominent I-bands (arrows). The sarcolemma is intact (arrowheads). These findings are consistent with the ultrastructural changes seen in reversibly injured myocardium.
C. Ultrastructure of normal myocardium. Myocardial cells are surrounded by an intact sarcolemma with abundant mitochondria.
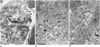
Acknowledgements
The authors wish to thank Chung-Hwan Lim, BS, Department of Radiology, Asan Medical Center, for his technical assistance in conducting MRI with animals, and Schering A.G., Berlin, Germany, for providing Gadophrin-2 and Gadomer-17.
References
1. Wackers FJ, Bodenheimer M, Fleiss JL, Brown M. Factors affecting uniformity in interpretation of planar thallium-201 imaging in a multicenter trial. The Multicenter Study on Silent Myocardial Ischemia(MSSMI) Thallium-201 Investigator. J Am Coll Cardiol. 1993. 21:1064–1074.
2. Klocke FJ. Measurements of coronary blood flow and degree of stenosis : current clinical implications and continuing uncertainties. J Am Coll Cardiol. 1983. 1:31–41.
3. Ni Y, Marchal G, Yu J, et al. Localization of metalloporphyrin-induced "specific" enhancement in experimental liver tumors: Comparison of MRI, microangiographic and histologic findings. Acad Radiol. 1995. 2:687–699.
4. Hindre F, LePlouzennec M, De Certaines JD, et al. Tetra-pamonophenylporphyrin-conjugated Gd-DTPA: Tumor-specific contrast agent for MR imaging. J Magn Reson Imaging. 1993. 3(1):59–65.
5. Young SW, Sidhu MK, Qing F, et al. Preclinical evaluation of gadolinium texaphyrin complex: a new paramagnetic contrast agent for magnetic resonance imaging. Invest Radiol. 1994. 29:330–338.
6. Ni Y, Marchal G, Herijgers P, et al. Paramagnetic metalloporphyrins: from enhancers of malignant tumors to markers of myocardial infarcts. Acta Radiol. 1996. 3(2):395–397.
7. Noh HN, Choi SI, Choi SH, et al. Gadomer-17 in contrast enhanced MR imaging of reperfused myocardial infarction in a cat model. J Kor Radiol Soc. 2000. 43:539–544.
8. Weinmann HJ, Brasch RC, Press WR, Wesby GE. Characteristics of gadolinium-DTPA complex: a potential NMR contrast agent. AJR. 1984. 142:619–624.
9. Saeed M, Wendland MF, Yu KK, Li HT, Higgins CB. Dual effects of gadodiamide injection in depiction of the region of myocardial ischemia. J Magn Reson Imaging. 1993. 3:21–29.
10. Muller RN, Vander Elst L, Colet JM, et al. Spectroscopic monitoring of the cellular internalization of paramagnetic ions and their complexes: a perfused cells and perfused organs approach. Acad Radiol. 1996. 3:suppl. 2. 277–281.
11. Watson AD, Rocklage SM, Carvlin MJ. Stark DD, Bradley WG, editors. Contrast agents. Magnetic resonance imaging. 1992. 2nd ed. St Louis, MO: Mosby;372–437.
12. Ni Y, Marchal G, Yu J, et al. Localization of metalloporphyrin-induced specific enhancement in experimental liver tumors: comparison of MRI, microangiographic and histologic findings. Acad Radiol. 1995. 2:687–699.
13. Jennings RB, Steenberger C, Reiner KA. Schien FJ, Gimbrone MA, editors. Myocardial ischemia and reperfusion. Cardiovascular pathology. 1995. 1st ed. Baltmore, MD: Williams & Wilkins;58–64.
14. Braunwald E. Myocardial reperfusion, limitation of infarct size, reduction of left ventricular dysfunction, and improved survival. Should the paradigm be expanded? Circulation. 1989. 79:441–444.
15. Whiteman G, Kieval R, Wetstein L, et al. The relationship between global myocardial redox state and high-energy phosphate profile: a phosphorus-31 nuclear magnetic resonance study. J Sur Res. 1983. 35:339.
16. Reimer KA, Lowe JE, Rasmussen MM, Jennings RB. The "wavefront phenomenon" of ischemic cell death. I: Myocardial infarct size vs duration of coronary occlusion in dogs. Circulation. 1977. 56:786–794.
17. Reimer KA, Jennings RB. The "wavefront phenomenon" of myocardial ischemic cell death. II: transmural progression of necrosis within the framework of ischemic bed size(myocardium at risk) and collateral flow. Lab Invest. 1979. 40:634–644.
18. Maxwell SR, Lip GY. Reperfusion injury: a review of the pathophysiology, clinical manifestations and therapeutic options. Int J Card. 1997. 58:95–117.
19. Jennings RB, Reimer KA. Factors involved in salvaging ischemic myocardium: effect of reperfusion of arterial blood. Circulation. 1983. 68:25–36.
20. Rivas F, Cobb FR, Bache RJ, Grrenfield JC. Relationship between blood flow to ischemic regions and extent of myocardial infarction. Circ Res. 1976. 38:439–447.
21. Jugdutt BI, Hutchins GM, Bulkey BH, Pitt B, Becker LC. Effect of indomethacin on collateral blood flow and infarct size in the conscious dog. Circulation. 1979. 59:734–743.
22. Gottlieb GJ, Kudo SH, Alonso DR. Ultrastructural characterization of the border zone surrounding early experimental myocardial infarctions in dogs. Am J Pathol. 1981. 103:292–303.
23. Yellon DM, Hearse DJ, Crone R, Granelle J. Characterization of the lateral interface between normal and ischemic tissue in the canine heart during evolving myocardial infarction. Am J Cardiol. 1981. 47:1233–1239.
24. Factor SM, Okun EM, Kirk ES. The histological lateral border of acute canine myocardial infarction. Circ Res. 1981. 48:640–649.
25. Lim T-H, Hong MK, Lee JS, et al. Novel application of breath-hold turbo spin-echo T2 MRI for detection of acute myocardial infarction. J Magn Reson Imaging. 1997. 7:996–1001.
26. Johnston D, Thompston R, Liu P. Magnetic resonance imaging during acute myocardial infarction. Am J Cardiol. 1986. 58:214–219.
27. Adzamli IK, Blau M, Pfeffer MA, Bavis MA. Phosphonate-modified Gd-DTPA complexes. III: the detection of myocardial infarction by MRI. Magn Reson Med. 1993. 29:505–511.
28. De Roos A, Matheijssen NA, Doornbos J, et al. Myocardial infarct size after reperfusion therapy: assessment with Ga-DTPA-enhanced MR imaging. Radiology. 1990. 176:517–521.
29. Saeed M, Wendland MF, Matusi T, Higgins CB. Reperfused myocardial infarction on T1- and susceptibility-enhanced MRI: evidence of loss compartmentalization contrast media. Magn Reson Med. 1994. 31:31–39.
30. Judd RM, Lugo OC, Aria M, et al. Physiological basis of myocardial contrast enhancement in fast magnetic resonance imaging of 2-day-old reperfused canine infarct. Circulation. 1995. 92:1902–1910.
31. Lim T-H, Lee JH, Lee TK, Mun CW. Comparison of gadolinium polylysine and gadopentetate in contrast-enhanced MR imaging of myocardial ischemia-reperfusion in cats. J Kor Radiol Soc. 1995. 33:59–65.
32. Choi SH, Jiang CZ, Lee TK, et al. Myocardial assessment during subacute stage after ischemia-reperfusion: Gd-DTPA-polylysine enhanced MR imaging in cats. J Kor Radiol Soc. 1998. 39:1069–1073.
33. Marchal G, Ni Y, Flameng W, et al. Paramagnetic metalloporphyrin: infarct-avid contrast agents for diagnosis of acute myocardial infarction by MRI. Eur Radiology. 1996. 6:2–8.
34. Choi SI, Choi SH, Kim ST, et al. Irreversibly damaged myocardium at MR imaging with a necrotic tissue-specific contrast agent in a cat model. Radiology. 2000. 215:863–868.