Abstract
Objective
Different angiographic protocols may influence the radiation dose and image quality. In this study, we aimed to investigate the effects of filtration and focal spot size on radiation dose and image quality for diagnostic cerebral angiography using an in-vitro model and in-vivo patient groups.
Materials and Methods
Radiation dose and image quality were analyzed by varying the filtration and focal spot size on digital subtraction angiography exposure protocols (1, inherent filtration + large focus; 2, inherent + small; 3, copper + large; 4, copper + small). For the in-vitro analysis, a phantom was used for comparison of radiation dose. For the in-vivo analysis, bilateral paired injections, and patient cohort groups were compared for radiation dose and image quality. Image quality analysis was performed in terms of contrast, sharpness, noise, and overall quality.
Results
In the in-vitro analysis, the mean air kerma (AK) and dose area product (DAP)/frame were significantly lower with added copper filtration (protocols 3 and 4). In the in-vivo bilateral paired injections, AK and DAP/frame were significantly lower with filtration, without significant difference in image quality. The patient cohort groups with added filtration (protocols 3 and 4) showed significant reduction of total AK and DAP/patient without compromise to the image quality. Variations in focal spot size showed no significant differences in radiation dose and image quality.
Conclusion
Addition of filtration for angiographic exposure studies can result in significant total radiation dose reduction without loss of image quality. Focal spot size does not influence radiation dose and image quality. The routine angiographic protocol should be judiciously investigated and implemented.
The advancement of various interventional techniques and image reconstruction modalities has resulted in an increase in radiation-based diagnostic and therapeutic procedures for neurovascular diseases (1). In angiographic studies, the image quality is important for diagnosis, planning and interventional therapy. However, the demand for superior image quality may come at the cost of high doses of radiation, which raises concerns for short-term and long-term radiation effects (2345).
Various dose reduction maneuvers, filtration, focal spot size, detector elements, and image acquisition/processing techniques have influences on the radiation dose and image quality (6789101112). The dose-saving effects of added filtration and the improved spatial resolution with smaller focal spot size are theoretically well known and deterministic (7131415). However, in real-life patient populations, the degree of impact of these individual factors on the total dose reduction and image quality is unclear. An angiographic study includes not only exposures, but also fluoroscopic studies. Recent advances in imaging modalities such as 3D rotational angiograms, flat panel CT, and perfusion imaging performed with the digital subtraction angiography (DSA) machine add to the complexity (16). Also, smaller focal spot size improves spatial resolution, but the actual clinical significance of using the smallest focus at the cost of increased tube loading is questionable (717). Thus, various protocols or the initial factory settings are used routinely by many centers without meticulous investigation (1112).
To address the issues related to reduction of the radiation dose for the routine cerebral angiography protocol in our institution, we conducted in-vitro and in-vivo investigations of the different protocols using different filtration and focal spot size. Based on the investigations, the routine clinical protocol was changed. The purpose of this study was to retrospectively evaluate the results of the investigation on the effects of filtration and focal spot size on radiation dose and image quality using in-vitro vascular phantom and in-vivo analysis consisting of bilateral paired injections, and to validate the results in real-life patient cohort groups.
The study was approved by the Institutional Review Board with waiver of informed consent due to its retrospective design.
All image acquisitions were performed on a flat panel biplane DSA machine (Philips Allura Xper FD 20/20, Philips, Best, the Netherlands). Radiation dose measurements were acquired using the on-board air kerma (AK) (mGy) and dose area product (DAP) (mGycm2) meters. Radiation dose and image quality were investigated by varying the filtration (inherent vs. 0.1 mm copper + 1 mm aluminum filtration) and focal spot size (small vs. large) protocols (1, inherent filtration + large focus; 2, inherent filtration + small focus; 3, copper filtration + large focus; 4, copper filtration + small focus) for DSA exposure studies. Inherent filtration refers to filtration from part of the X-ray tube and housing without copper/aluminum filters. The focal spot size of the frontal/lateral tubes on this equipment were 0.4/0.5 mm for small and 0.7/0.8 mm for large foci. Our initial clinical protocol (protocol 1) was compared with the new protocols (protocols 2, 3, and 4).
The investigation consisted of three parts. The in-vitro analysis was performed to compare the DSA exposure radiation dose between the protocols using a phantom. An in-vivo analysis (bilateral paired injections) was performed to compare the image quality and the dose results between the protocols on standardized DSA exposure studies. A second in-vivo analysis (patient cohorts) was performed to evaluate the real-life impact of these protocols on the total dose per procedure, which includes various exposure, fluoroscopic, and 3D rotational angiogram studies, and image quality in routine/magnified diagnostic studies in a cohort of patients with aneurysms.
A homemade silicone aneurysm phantom was immersed in a water container (20 × 21 × 30 cm). Measurement of mean radiation dose in terms of AK/frame and DAP/frame were performed simulating a 20-frame DSA exposure while injecting 7 mL non-diluted contrast at 4 mL/sec. The number of exposures, field of view, table height, source to image distance, and tube angulations were controlled for all injections.
The DSA exposure protocols were compared by bilateral paired injections in 5 patients, respectively. Nine bilateral internal carotid artery and 8 bilateral vertebral artery pairs of normal angiograms were included. Radiation dose and image quality were compared between the ipsilateral and the contralateral anteroposterior (AP) and lateral views (protocol 1 vs. 4; and protocol 3 vs. 4) (Fig. 1). The projection angles, number of exposures, field of view, table height, source to distance, and tube angulations were matched for each paired injection. Injections were excluded from evaluation when selection of the target vessel failed or when there was marked asymmetricity of the vascular distribution. Protocol 2 was not implemented on any of the patients due to concerns of high-dose radiation exposure according to the in-vitro analysis.
Twenty-five consecutive patients studied with protocols 3 and 4, respectively, were compared with a historical group of 25 consecutive patients studied with protocol 1. The total radiation dose (AK and DAP) per examination and the image quality were compared. For each patient, image quality analysis was performed on the routine standardized AP/lateral projection of the vessel of interest bearing the aneurysm and its magnified working projection run. All magnified working projections were obtained from the reconstructed 3D rotational angiogram with full magnification (FD, 15 cm). The age, sex, fluoroscopic time, number of aneurysms, total exposure images, total number of runs, and number of 3D rotational angiograms were compared for each protocol.
Image quality of the in-vivo analysis in terms of contrast, sharpness, noise, and overall quality on a 10 point scale (10, perfect; 8, excellent; 5, good; 3, fair; 1, poor) was performed by three neurointerventionists who were blinded to the study. The image quality features were defined as follows: contrast, ability to detect branch vessels; sharpness, ability to characterize the lesion and branch vessels; noise, graininess of image; and overall, overall preference of the image. The image quality scores were evaluated as a mean value of the three readers. To adjust for inter-rater variability, the image quality scores in six additional angiographic runs (three patients) who were not included in the in-vivo analysis were evaluated by the three readers. The difference between the individual and the total reviewers' median scores for the respective imaging features were obtained from the additionally evaluated angiograms. The adjusted image quality score was calculated by calibrating for this difference from the unadjusted image quality scores.
Statistical analysis was performed using the t test or one-way analysis of variance for comparison of dose and image quality scores between the protocols (MedCalc Statistical Software, v15.8, Ostend, Belgium). The Scheffe test was used for post hoc analysis. The Mann-Whitney and Kruskal Wallis test was used for non-parametric data. p value of < 0.05 was considered as significance.
The mean AK/frame showed a significant difference between the protocols (Table 1). Protocols using inherent filtration (protocols 1 and 2) showed a significantly higher AK/frame dose, as compared to those with added filtration (protocols 3 and 4). DAP/frame was also significantly higher with the protocols using inherent filtration (protocols 1 and 2), as compared to those with added filtration (protocols 3 and 4; Table 1). The mean kV and mA values were 80.0, 80.0, 80.0, 85.7, and 55.7, 58.0, 71.3, 59.0, respectively.
Protocol 4 showed a significantly lower dose than protocol 1. Protocol 4 showed a 52.9% reduction of AK/frame (2.97 ± 0.66 mGy vs. 6.30 ± 1.70 mGy), as compared to protocol 1. Likewise, protocol 4 showed a 54.7% reduction of DAP/frame (1028.88 ± 169.04 mGycm2 vs. 2272.88 ± 609.12 mGycm2), as compared to protocol 1 (Table 2). The mean AK/frame and DAP/frame values showed no significant difference between the large (protocol 3) and the small focal spot size (protocol 4) studies (Table 2). No significant differences were noted in the overall unadjusted/adjusted image quality scores between the protocols. Specific imaging features including contrast, sharpness, and noise showed significant differences between the protocols.
The baseline characteristics of the angiographic study such as the mean age, sex ratio, fluoroscopic time, number of aneurysms, total number of exposure images, angiographic runs, 3D rotational angiograms, and working projections/patient did not differ significantly between the protocols (Table 3). The results of the bilateral paired injections were maintained in the real-life patient cohort analysis in terms of mean total dose/patient, which included DSA exposure runs, fluoroscopy, and 3D rotational angiograms. Patients examined with protocols 3 and 4 showed a significantly lower dose, as compared to those with protocol 1. Protocol 3 showed a 40.9% reduction of the mean total AK/patient (987.89 ± 260.21 mGy vs. 1671.91 ± 380.99 mGy), as compared to protocol 1. Likewise, protocol 3 showed a 25.6% reduction of DAP/patient (165783.88 ± 48924.18 mGycm2 vs. 222759.76 ± 40524.46 mGycm2), as compared to protocol 1. Protocol 4 showed a 50.1% reduction of the mean total AK/patient (834.95 ± 181.04 mGy vs. 1671.91 ± 380.99 mGy), as compared to protocol 1. Protocol 4 showed a 37.4% reduction of DAP/patient (139376.36 ± 30862.78 mGycm2 vs. 222759.76 ± 40524.46 mGycm2), as compared to protocol 1 (Table 4). The mean overall unadjusted/adjusted image quality scores showed no significant difference for routine and magnified working projections (Table 5). In addition, the contrast, sharpness, and noise showed no significant difference in the effect on mean unadjusted/adjusted image quality scores.
No remarkable complications occurred related to the angiographic procedures.
Our study highlighted the effects of various filtration and focal spot size protocols on radiation dose and image quality. For a routine cerebral angiographic study, addition of filtration resulted in approximately a 40–50% reduction of total AK/patient and 25–40% of total DAP/patient. Small or large focal spot size had no significant impact on dose and image quality in real-life patients.
The effects of radiation can be categorized into stochastic and deterministic effects. In the stochastic effects, changes induced in a single cell may be sufficient to initiate a biological process such as neoplasms; and the likelihood of initiating the event increases with dose (18). Deterministic effects require a threshold for the biological effects to become apparent. These include cataracts, skin erythema, and alopecia. The severity of the induced effect increases rapidly above the threshold (18). No visible skin effects are anticipated when a single site acute skin-dose is < 2 Gy, but clinically important skin and hair reactions occur when the skin dose is > 5 Gy (19). The dose for various angiographic procedures may vary based on the nature of lesion, anatomy, and complexity of the procedure. According to Miller et al. (3), the mean cumulative dose for a hepatic chemoembolization and cerebral aneurysm embolization were 1.4 Gy and 3.8 Gy, respectively. The mean radiation dose in our routine cerebral angiogram for the evaluation of aneurysm was < 2 Gy for all protocols. However, the total dose varied significantly by protocol (0.83–1.67 Gy) (Table 4). Protocol 1 using only inherent filtration had a two-fold higher dose as compared to the protocols using additional copper filtration (protocols 3 and 4). Since many of these patients are treated endovascularly for the lesions and are thus exposed to additional radiation, the dose difference is not negligible and should be considered with caution.
Methods for reduction of radiation dose during fluoroscopic and angiographic studies are well known. Operator-dependent maneuvers such as maximizing the distance between the X-ray tube and the patient, minimizing the distance between the patient and the detector, avoiding magnification, use of collimation, and lower frame/pulse rates are manipulations that can be performed by the operator for effectively reducing the intraprocedural dose (92021). Non-operator-dependent default factors include filtration, focal spot size, and image reconstruction algorithms, which are either set up as a routine protocol or built into the system and are difficult to manipulate during a procedure (8222324). Thus, setting up an appropriate default protocol as a routine in accordance with the ‘As Low As Reasonably Achievable’ principle is important for saving dose. Schneider et al. (23) implemented a dose reduction strategy in their institution consisting of lower and variable DSA frame rates and tailored examinations, which resulted in > 60% reduction in radiation dose over a course of 4 years. Honarmand et al. (12) used a manually reduced low-dose protocol (1.2 µGy/frame), which resulted in a dose reduction of at least two-fold without compromise to the image quality, as compared to the baseline factory setting (3.6 µGy/frame). Our results also supported these findings, suggesting that improvised protocols with alterations in filtration may have significant benefits in terms of the radiation dose without perceptible difference in the image quality.
In our study, we specifically evaluated the effects of filtration and focal spot size on the radiation dose and image quality in DSA exposure studies. Filtration reduces skin dose by removing low energy photons that would not be able to penetrate deep and contribute to the image (1314). Nicholson et al. (13) showed that addition of 0.18–0.35-mm copper filtration results in a 42–58% reduction in skin dose rate on water phantoms, which is similar to our results. In addition, results from our patient cohort study indicated that protocols with copper filtration (protocol 3 and 4) did not compromise the image quality. Thus, the 0.1-mm copper filtration should be included in the routine cerebral angiogram protocol.
In terms of focal spot size, products from different vendors have different built-in foci. The Philips Allura Xper used in this study uses 0.4/0.7 mm (small/large) while the Siemens Artis Q uses 0.3/0.4/0.7 mm (micro/small/large) foci. In-vitro studies show that smaller focal spot improves spatial resolution by reducing the geometric unsharpness and the penumbra of the X-ray beam at the detector (725). It is known to improve the spatial resolution of microcalcifications in mammograms and marginal clarity of lesions in abdominopelvic computed tomography (71526). However, the clinical implications of the focal spot size may vary among the various modalities and organs. Our results showed that the use of small focus compared to large focus had no significant influence on the perceptible image quality of cerebral angiograms. These effects were maintained not only on routine standard projections but also on the maximum magnified working projections of cerebral angiograms for aneurysms. In terms of dose difference between large and small foci, the mean dose values were slightly higher for the large focus protocols, without significance. These results suggested that either large or small foci with filtration may be used without compromise to the image quality (protocols 3 and 4). However, considering the theoretical advantages, a smaller focus may be preferred in patients who require maximum resolution for very fine or subtle lesions, such as vasculitis. In this regard, the advantages of micro-focus available through some vendors, in terms of dose and detailed image quality, need further investigation.
Higher loading on the X-ray tube is a concern when using added filtration and small focus (1314). Addition of copper filtration results in an increase in tube loading because the X-ray tube output is increased to maintain the necessary exit beam. Our in-vitro results showed increased kV and mA with protocols 3 and 4. This rate of increase in tube loading differs according to the filtration material and the kVp range (14). Excessive use of small focal spot size may result in a small target area resulting in overheating of the anode and shortened tube duty cycle. Application of advanced cooling mechanisms on some newer systems may reduce these effects (6717).
The bilateral paired injections compared the exposure dose and image quality between the protocols. However, the relative proportions of radiation dose by exposure, fluoroscopy and other imaging studies included in a routine procedure may differ between diagnostic or interventional procedures (16). The results of bilateral paired injections were maintained in our patient cohort groups in terms of effectively reducing the total radiation dose for diagnosis of cerebral aneurysms, which typically includes various angiographic exposure, fluoroscopic and 3D rotational studies. The impact of the altered protocols on the dose and image quality remains to be investigated in other interventional procedures.
Prior studies have implemented a repeated injection protocol on the same vessel in which the image quality is evaluated during the procedure and, if satisfactory, the remaining procedure is performed with the low-dose protocol (22). Although this may be the ideal method for comparing dose and image quality, the patient may be at risk for a repeated injection if the low-dose protocol is not satisfactory, resulting in increased radiation dose and contrast injection. Thus, we chose a bilateral paired injection method. The individual patient factors such as bone/air density were controlled and no repeated injections were performed, thus minimizing the risk of increased contrast injection and radiation dose.
This study is limited by its retrospective design. The data from the routine clinical practice that had been implemented for selecting the protocols was retrospectively evaluated. Thus, the respective effects of various filtration and focus protocols could not be fully evaluated, for e.g., protocol 2 (inherent filtration + small focus) was not included in our clinical protocol due to concerns of high dose. Also, all evaluations were performed on a single DSA machine. Different focal spot sizes in DSA equipment from other vendors may show different results in terms of image quality, thus generalization should be performed with caution. Nonetheless, the protocols for each equipment should be thoroughly investigated before routine clinical application using phantom or in-vivo methods, such as bilateral paired injections.
In conclusion, various protocols with altered filtration and focal spot size can be implemented for DSA studies. Our study showed that addition of 0.1 mm Cu filtration can result in significant radiation dose reduction without loss of image quality in diagnostic cerebral angiograms. Large or small focal spot size showed no significant impact on the radiation dose and image quality; hence, considering the theoretical advantages/disadvantages, small focus may be reserved for cases wherein fine or detailed lesions are anticipated. The routine angiographic protocol should be judiciously investigated and clinically implemented at all institutions performing angiographic studies.
References
1. Alexander MD, Oliff MC, Olorunsola OG, Brus-Ramer M, Nickoloff EL, Meyers PM. Patient radiation exposure during diagnostic and therapeutic interventional neuroradiology procedures. J Neurointerv Surg. 2010; 2:6–10. PMID: 21990551.


2. Cohnen M, Wittsack HJ, Assadi S, Muskalla K, Ringelstein A, Poll LW, et al. Radiation exposure of patients in comprehensive computed tomography of the head in acute stroke. AJNR Am J Neuroradiol. 2006; 27:1741–1745. PMID: 16971627.
3. Miller DL, Balter S, Cole PE, Lu HT, Schueler BA, Geisinger M, et al. Radiation doses in interventional radiology procedures: the RAD-IR study: part I: overall measures of dose. J Vasc Interv Radiol. 2003; 14:711–727. PMID: 12817038.


4. O'Brien B, van der Putten W. Quantification of risk-benefit in interventional radiology. Radiat Prot Dosimetry. 2008; 129:59–62. PMID: 18287187.
5. Orbach DB, Stamoulis C, Strauss KJ, Manchester J, Smith ER, Scott RM, et al. Neurointerventions in children: radiation exposure and its import. AJNR Am J Neuroradiol. 2014; 35:650–656. PMID: 24157736.


6. Kei Ma W, Hogg P, Norton S. Effects of kilovoltage, milliampere seconds, and focal spot size on image quality. Radiol Technol. 2014; 85:479–485. PMID: 24806050.
7. Goh YP, Lau KK, Low K, Buchan K, Oh LC, Kuganesan A, et al. Fine focal spot size improves image quality in computed tomography abdomen and pelvis. Eur Radiol. 2016; 26:4545–4550. PMID: 26973145.


8. Söderman M, Mauti M, Boon S, Omar A, Marteinsdóttir M, Andersson T, et al. Radiation dose in neuroangiography using image noise reduction technology: a population study based on 614 patients. Neuroradiology. 2013; 55:1365–1372. PMID: 24005833.


9. Mahesh M. Fluoroscopy: patient radiation exposure issues. Radiographics. 2001; 21:1033–1045. PMID: 11452079.


10. Aichinger H. Radiation exposure and image quality in X-ray diagnostic radiology: physical principles and clinical applications. 2nd ed. Heidelberg: Springer;2012.
11. Kahn EN, Gemmete JJ, Chaudhary N, Thompson BG, Chen K, Christodoulou EG, et al. Radiation dose reduction during neurointerventional procedures by modification of default settings on biplane angiography equipment. J Neurointerv Surg. 2016; 8:819–823. PMID: 26245735.


12. Honarmand AR, Shaibani A, Pashaee T, Syed FH, Hurley MC, Sammet CL, et al. Subjective and objective evaluation of image quality in biplane cerebral digital subtraction angiography following significant acquisition dose reduction in a clinical setting. J Neurointerv Surg. 2017; 9:297–301. PMID: 27053704.


13. Nicholson R, Tuffee F, Uthappa MC. Skin sparing in interventional radiology: the effect of copper filtration. Br J Radiol. 2000; 73:36–42. PMID: 10721318.


14. Kohn ML, Gooch AW Jr, Keller WS. Filters for radiation reduction: a comparison. Radiology. 1988; 167:255–257. PMID: 3347728.


15. Law J. The influence of focal spot size on image resolution and test phantom scores in mammography. Br J Radiol. 1993; 66:441–446. PMID: 8319066.


16. Chun CW, Kim BS, Lee CH, Ihn YK, Shin YS. Patient radiation dose in diagnostic and interventional procedures for intracranial aneurysms: experience at a single center. Korean J Radiol. 2014; 15:844–849. PMID: 25469098.


17. Bushberg J, Seibert J, Leidholdt J, Boone J. essential physics of medical imaging. 3rd ed. Philadelphia: Lippincott Willians & Wilkins;2012.
18. Wagner LK, Eifel PJ, Geise RA. Potential biological effects following high X-ray dose interventional procedures. J Vasc Interv Radiol. 1994; 5:71–84. PMID: 8136601.


19. Balter S, Hopewell JW, Miller DL, Wagner LK, Zelefsky MJ. Fluoroscopically guided interventional procedures: a review of radiation effects on patients’ skin and hair. Radiology. 2010; 254:326–341. PMID: 20093507.


20. Sanchez RM, Vano E, Fernández JM, Moreu M, Lopez-Ibor L. Brain radiation doses to patients in an interventional neuroradiology laboratory. AJNR Am J Neuroradiol. 2014; 35:1276–1280. PMID: 24627454.


21. Stecker MS, Balter S, Towbin RB, Miller DL, Vañó E, Bartal G, et al. Guidelines for patient radiation dose management. J Vasc Interv Radiol. 2009; 20(7 Suppl):S263–S273. PMID: 19560006.


22. Söderman M, Holmin S, Andersson T, Palmgren C, Babic D, Hoornaert B. Image noise reduction algorithm for digital subtraction angiography: clinical results. Radiology. 2013; 269:553–560. PMID: 23737536.


23. Schneider T, Wyse E, Pearl MS. Analysis of radiation doses incurred during diagnostic cerebral angiography after the implementation of dose reduction strategies. J Neurointerv Surg. 2016; 4. 07. [Epub ahead of print]. DOI: 10.1136/neurintsurg-2015-012204.


24. Pearl MS, Torok C, Wang J, Wyse E, Mahesh M, Gailloud P. Practical techniques for reducing radiation exposure during cerebral angiography procedures. J Neurointerv Surg. 2015; 7:141–145. PMID: 24489125.


25. Poletti J, McLean D. Monte Carlo simulation of the effect of focal spot size on contrast-detail detectability. Australas Phys Eng Sci Med. 2012; 35:41–48. PMID: 22143903.


26. Sickles EA. Further experience with microfocal spot magnification mammography in the assessment of clustered breast microcalcifications. Radiology. 1980; 137(1 Pt 1):9–14. PMID: 7422866.


Fig. 1
Anteroposterior views of bilateral paired internal carotid artery (ICA) injections (Rt ICA, protocol 4; Lt ICA, protocol 1).
Number of exposures, field of view, table height, source to distance, and tube angulations were matched for both injections. Despite lack of perceptible difference in image quality, about 40–50% of total AK and 25–40% of total DAP reduction was seen in patients studied with protocol 4. AK = air kerma, DAP = dose area product
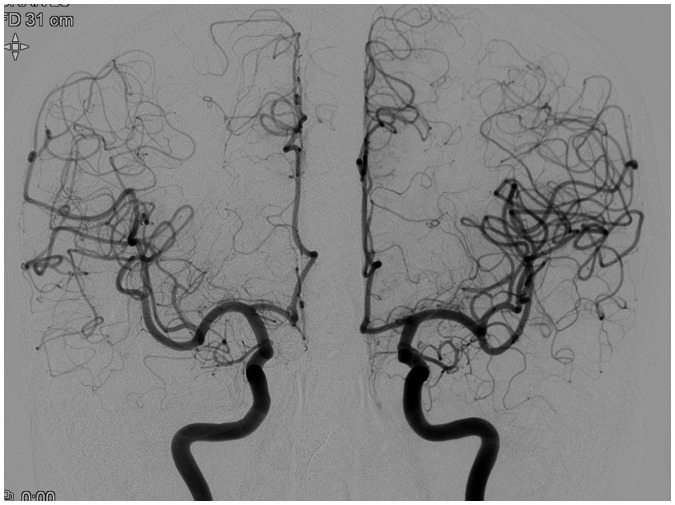
Table 1
In-Vitro Comparison of Mean Radiation Dose Using Phantom Device

Table 2
In-Vivo Bilateral Paired Injection Comparison of Mean Radiation Dose and Image Quality
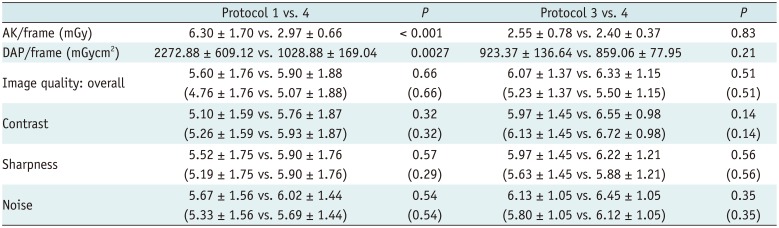
Table 3
Comparison of General Characteristics of Cohort Groups
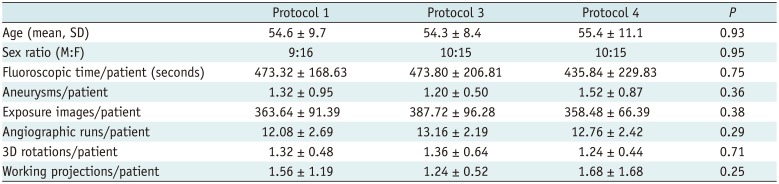
Table 4
Comparison of Total Mean Radiation Dose/Patient of Cohort Groups for Routine Cerebral Angiographic Study

Table 5
Comparison of Mean Image Quality Scores of Cohort Groups
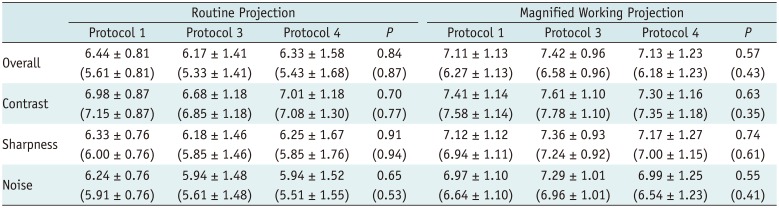