Abstract
Radiofrequency ablation (RFA) is a well-known, effective, and safe method for treating benign thyroid nodules and recurrent thyroid cancers. Thyroid-dedicated devices and basic techniques for thyroid RFA were introduced by the Korean Society of Thyroid Radiology (KSThR) in 2012. Thyroid RFA has now been adopted worldwide, with subsequent advances in devices and techniques. To optimize the treatment efficacy and patient safety, understanding the basic and advanced RFA techniques and selecting the optimal treatment strategy are critical. The goal of this review is to therefore provide updates and analysis of current devices and advanced techniques for RFA treatment of benign thyroid nodules and recurrent thyroid cancers.
Radiofrequency ablation (RFA) is an effective and safe method for treating benign thyroid nodules (12345) and recurrent thyroid cancers (6789). RFA has recently been suggested as an alternative treatment modality for primary thyroid microcarcinomas (101112). The 2012 Recommendations of the Korean Society of Thyroid Radiology (KSThR) (13) introduced indications, thyroid-dedicated devices, and basic techniques for thyroid RFA. Since 2012, thyroid RFA has been adopted worldwide, with subsequent advances made in devices and techniques, such as shorter and thinner electrodes, bipolar electrodes, virtual needle tracking systems, and unidirectional electrodes (14151617). Two basic techniques, the trans-isthmic approach and the moving shot technique, have been validated in many studies. Additionally, advanced techniques such as vascular ablation and hydrodissection have also been developed (1819). To improve efficacy and to minimize complications, it is important to understand these novel devices and the recently developed techniques. This review describes and analyzes the current devices and advanced techniques for RFA of benign thyroid nodules and recurrent thyroid cancers.
The trans-isthmic approach and moving shot technique are two fundamental methods for thyroid RFA. The trans-isthmic approach refers to the insertion of a RF electrode via the isthmus, in a midline to lateral direction, for treatment of a nodule in either the right or left thyroid gland (2021). There are several advantages in using the trans-isthmic approach. First, the operator can monitor the association between the electrode, target nodule, and recurrent laryngeal nerve, which is situated in the so called “danger triangle” between the trachea and thyroid gland. Constant monitoring of the relationship between the active tip and recurrent laryngeal nerve is very important for prevention of possible thermal injury during the procedure. Second, the normal isthmic parenchyma between the target nodule and the electrode insertion site prevents the leakage of hot ablated fluid to the perithyroidal area, which would otherwise cause pain. Finally, using trans-isthmic approach, the electrode position remains stable even when a patient talks or coughs.
Application of RF current to tissue agitates ions as they attempt to follow changes in the direction of the alternating current. Such agitation creates frictional heat around the electrode, causing instantaneous protein denaturation and irreversible cell death. Although this frictional heat rapidly damages the surrounding tissue, the damage is limited to the immediate vicinity of the electrode. Simultaneously, tissue remote from the electrode is heated slowly, via thermal conduction from the center of the ablation zone (1522).
In conventional RFA of other organs such as the liver, the active tip of the electrode is fixed to the center of the target tumor, which is ablated by the conducted heat; the ablation zone resulting from the fixed electrode technique is round in shape (23). However, as thyroid nodules are often ellipsoidal exophytic from the thyroid gland, they are difficult to ablate using the fixed technique. Also, many critical structures surround the thyroid gland, such as the esophagus, trachea, recurrent laryngeal nerve, carotid artery, vagus nerve, and cervical sympathetic ganglion (24). Using the fixed technique, the nodule periphery and immediate surrounding tissue may be undertreated or overtreated, depending on the treatment time and energy applied. Therefore, it is advantageous to treat a thyroid nodule by dividing it into multiple small ablation units. These ablation units can be treated individually by using the moving shot technique (2526). Frictional heat generated from the electrode is then focused on ablation of the thyroid nodule, thereby limiting damage to surrounding tissue. In order to prevent limitation of the acoustic window by air bubbles, the electrode tip can be initially positioned in the deepest and most remote portion of the nodule, with subsequent retraction to the most superficial portion of the nodule. Moreover, the ablation should be suspended when the electrode moves in a forward direction, to prevent damage to critical structures (24). Ablation is safer when the tip of the electrode is well positioned within a 1–2 mm distance from the target nodule margin (27). Since there is a gradual increases of the ablation zone when the electrode tip stays at one area, it is recommended to pull back the electrode to ablate the nodule unit-by-unit, with ablation time of several seconds for each unit (428).
There have been numerous advances in RFA devices for treating thyroid nodules (Table 1) (15161729). As the thyroid gland is relatively small and superficially located, thyroid-dedicated electrodes for RFA are necessary (30). Thyroid dedicated modified internally cooled electrodes (7 cm, 18-gauge) are shorter and thinner than the conventional electrodes used in other organs (173031). Multiple active tips have been used (5, 7, and 10 mm) (7), and currently a new 3.8 mm tip is in use for the smaller recurrent cancers. Small active tips allow precise treatment with minimal collateral tissue damage to the adjacent critical structures (732). A thinner 19-gauge electrode has also been recently developed, and is useful to puncture small recurrent cancers (733).
Bipolar RFA has been used in liver, kidney, and muscle treatments (23). Unlike monopolar electrodes, use of bipolar electrodes simplifies the procedure, as grounding pads are not required. Grounding pads are necessary in monopolar RFA systems since the electric current runs through the entire body, whereas with a bipolar electrode the zone of electric current is limited to the area surrounding the active tip of the electrode (1415). Localization of the electric current makes bipolar use safer in pregnant women and in patients with implanted electrical devices such as pacemakers (1415). Moreover, limiting the electrical current to the area surrounding the bipolar electrode, results in a more predictable size and shape of the ablation zone. The localized current may also generates a higher energy density, leading to a shorter procedure time. The risk of ground pad skin burns is also eliminated using bipolar RFA (34). However, the shape of ablation zone achieved by bipolar electrode tends to be ellipsoidal, and the ablation area is smaller compared to that of unipolar electrode, which can be disadvantageous.
Thyroid RFA using the moving shot technique is a proven safe and effective method (2230). This technique may be difficult for beginners, since constant monitoring of the tip location is required, while simultaneously moving and keeping the electrode within the target nodule during ablation. Straying of the electrode tip outside the target nodule could cause thermal injury to adjacent vulnerable structures (e.g., carotid artery, vagus nerve, cervical sympathetic ganglion, and esophagus), resulting in serious complications (35). There are several reasons why active tip monitoring may be difficult during the procedure. Firstly, a transient hyperechoic zone generated during the ablation creates a posterior acoustic shadow that interferes with the sonic window (4). Secondly, if the nodule is very large, the posterior margin may be indistinct or poorly visualized on ultrasound (US). Several techniques have been suggested to improve electrode monitoring. One approach is the US-CT or US-MRI fusion technique, which is mainly used for RFA in the liver (363738). The other is the virtual needle tracking system, which continuously tracks the RF electrode position using a small sensor on the shaft (17). Commencement of thyroid RFA creates a transient hyperechoic zone by vaporization of nodule tissue, impeding the monitoring of the electrode tip; however, the on-screen guidance shows the direction of travel, with “V” representing the actual location of the electrode tip (Supplementary Movie 1 in the online-only Data Supplement) (17). According to Turtulici et al. (17), this system increases the effectiveness of thyroid RFA, reduces the complication rate, and shortens the procedural time and learning curve, especially for beginners. There are several pitfalls to the needle tracking system. If the RF electrode is advanced along the same path as the virtual tract without any bending occurring, the tip of the RF electrode coincides exactly with the target. However, when dealing with hard nodules, the RF electrode may bend considerably, producing inaccuracies between the virtual track and the actual electrode tip location. Moreover, too much pressure on the US probe may change the perceived electrode depth, inducing errors in the correlation between the “V” annotation and actual location of the electrode tip.
When a thyroid nodule abuts critical structures, it is important to minimize the non-target thermal injuries. In such situations, a unidirectional ablation electrode (UAE) is useful, as this partially insulated electrode (270° of insulation) (Fig. 1) creates a half-moon-shaped ablation zone, which ablates tumors that are partially attached to a critical structure without causing thermal injury (39). A UAE is especially useful for the treatment of small recurrent thyroid cancers or secondary hyperparathyroidism abutting critical structures (16). Although several experimental studies on UAE have been conducted, none of the studies evaluated the clinical application of UAE for the treatment of thyroid nodules (16). Therefore, further investigations are necessary to evaluate its clinical efficacy.
To reduce pain during the RFA, it is important to inject sufficient lidocaine into the skin puncture site and thyroid capsule (2239). Sensory nerves are usually present at the thyroid capsule, but not inside the thyroid gland. The lidocaine needle should be inserted at the midline of the anterior neck, just above the thyroid isthmus, and advanced into the thyroid capsule. Before proceeding to the thyroid capsule, the operator should be aware of vessels surrounding the thyroid gland (e.g., the superior thyroid artery) and be careful not to puncture them. Once the needle tip abuts the thyroid capsule, a perithyroidal lidocaine injection is performed. The collected lidocaine appears as an anechoic band separating the thyroid gland and strap muscle (Fig. 2), which gradually disappears as the injected lidocaine flows inferiorly into the mediastinum. An appropriately performed perithyroidal lidocaine injection minimizes pain during the procedure (40). Hence, thyroid RFA is tolerable with local anesthesia using lidocaine (3541). Moreover, major complications are decreased by monitoring the presence of pain during the procedure, as pain is an early indicator of thermal propagation outside of the thyroid gland (35). Since patients are only under local anesthesia, complications such as voice change and ptosis can be continuously monitored by communicating with them. General anesthesia or moderate sedation may delay the detection of these complications (42).
Vascular ablation technique has been recently developed. Two different vascular ablation techniques are available: artery-first ablation and Marginal venous ablation. Artery-first ablation technique can be applied to hypervascular thyroid nodules with prominent feeding artery. However, marginal venous ablation technique is useful for most thyroid nodules, since thyroid nodules usually have marginal draining veins.
Benign thyroid nodules are sometimes hypervascular (434445). It is well known that the efficacy of RFA decreases when treating hypervascular tumors, because of the heat-sink effect (446). Several strategies have been proposed to minimize the heat-sink effect during RFA in other organs. For example, a vessel clamp or transarterial chemoembolization is used in the treatment of hepatocellular carcinomas near the hilum (4748). For thyroid nodules, the arterial or venous flow can be decreased by direct vascular ablation. Ablating the draining vein results in diffuse edema of the thyroid gland, therefore the “artery-first” ablation technique is used to effectively reduce the heat-sink effect in hypervascular tumors. This technique ablates the main feeding artery as the first step, and can be easily applied to a nodule with a feeding artery entering through the isthmus. Arteries supplying upper pole nodules usually arise from the superior thyroid artery, while arteries supplying lower pole thyroid nodules typically arise from the inferior thyroid artery. Doppler US is useful for the identification of the nodule's main arterial supply (Fig. 3) (Supplementary Movie 2 in the online-only Data Supplement). The artery-first ablation technique not only decreases the heat-sink effect, but also limits hemorrhage risk during the procedure. Intra-nodular hemorrhage during RFA increases the volume of a target nodule and interferes with the thermal conduction of RFA (49). When the active tip of an electrode ablates the feeding artery, intranodular linear echogenicities are generated, which then spread to the periphery of the target nodule (Fig. 4). These intranodular linear echogenicities are mostly due to microbubbles filling the arterioles. In some nodules, wedge-shaped hypoechoic changes are seen after the ablation of the main feeding artery of a target nodule. This wedge-shaped hypoechoic area may represent infarction in the area supplied by the feeding artery (Fig. 5). However, this phenomenon does not occur in every ablation, as hypervascular thyroid nodules often have multiple bypassing feeding arteries (24).
Draining veins along the margin of a thyroid nodule are prominent in most nodules. During RFA, venous drainage increases the heat-sink effect and interferes with complete ablation of the margin of the target nodule (24). To resolve this problem, the marginal vein is ablated after punctured by the electrode tip. When the vein is ablated, air bubbles fill the marginal vein of the nodule, and rapidly flow along the marginal vein as long as there is a remaining venous flow. However, when venous flow gradually decreases and eventually ceases, the air bubbles remain inside the veins, indicating a complete ablation of the marginal vein (Supplementary Movie 3 in the online-only Data Supplement). This technique is useful for marginal veins at the anterior portion of thyroid nodules, which have a sufficiently large safety margin for ablation. According to previous studies, the thermal ablation of benign thyroid nodules has focused on a decrease in volume of the target nodule, rather than complete ablation (50). However, marginal recurrence is often seen during long-term follow up, especially after the ablation of hyperfunctioning thyroid nodules (3051). According to Valcavi et al. (52), there is a tendency for marginal recurrence after 3 years of follow up. Moreover, Døssing et al. (53) reported that 29% of patients treated with laser ablation underwent surgery for marginal recurrence in the 5-year long-term follow up period. In a study by Lim et al. (19), operators attempted to treat the target nodule margins by complete utilization of venous staining, resulting in 93.5% volume reduction and a 4-year follow up with low (5.6%) marginal recurrence rate. The data published indicates that the marginal venous ablation technique may be helpful in preventing recurrence by complete tumor ablation (5455). However, thermal damage to the perithyroidal structure may be induced when applying marginal venous ablation technique, especially for hypervascular thyroid nodules located at the lateral aspect of the thyroid gland. Therefore, it is important to monitor the perithyroidal structures, including the vagus nerve and middle cervical ganglion, during the ablation (24).
An understanding of US-based neck anatomy is essential to improve the efficacy of RFA in the treatment of thyroid and neck tumors (e.g., benign thyroid nodules, recurrent thyroid or neck cancer, and parathyroid lesions) (2451). The neck is relatively narrow, containing many critical structures; therefore, it is difficult to treat a tumor completely. Hydrodissection is effective in separating tumor from adjacent critical structures (39). This technique has also been used in the liver, to avoid thermal injury to surrounding critical structures (5657). A similar technique can be used for the treatment of benign thyroid nodules or recurrent thyroid cancer. Before the insertion of a RF electrode, 5% dextrose is injected between the target tumor and an adjacent critical structure (Fig. 6). However, in the neck, the injected fluid may spread rapidly along the longitudinally arranged neck muscle plane (7). To achieve a sufficient safety margin by hydrodissection, fluid should be injected continuously during the procedure, with the injection needle remaining in place. A solution of 5% dextrose is recommended as the injection material, since normal saline is an anionic fluid and is therefore able to conduct electricity (18). The current applied for the ablation procedure would be carried through the saline to the adjacent structures, resulting in their damage due to frictional heating. However, because of its iso-osmolarity (252 mOsmol/L) and nonionic composition, 5% dextrose does not conduct electricity and potentially provides a thermal barrier when it surrounds the target organ (18). Transient voice change may occur after hydrodissection due to compression or mild irritation of the recurrent laryngeal nerve by cold fluid. However, palsy usually resolves within 30 minutes of the end of the procedure. To check for tiny nerves that are not well visualized on US (e.g., spinal accessory nerve, greater auricular nerve), a provocation test can be performed with lidocaine injection into the soft tissues surrounding the tumor; which allows the identification of any critical nerves near to the tumor (24).
Figures and Tables
Fig. 1
Unidirectional ablation electrode.
A. Longitudinal view. B. Transverse view. Active tip is partially insulated, so that it creates half-moon-shaped ablation zone. Red: ablation zone, white: non-insulated portion, black: insulated portion.
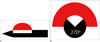
Fig. 2
Perithyroidal lidocaine injection.
Collected lidocaine appears as anechoic band (arrows) separating thyroid gland and strap muscle.
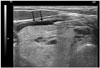
Fig. 3
Ablation of feeding artery.
A. Before thyroid RFA, main feeding artery from superior thyroid artery is identified on Doppler study. B. After ablation of main feeding artery, no more vascular signal is seen on Doppler study, suggesting complete ablation of vessels. RFA = radiofrequency ablation
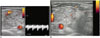
Fig. 4
Artery-first ablation technique.
Intranodular linear echogenicities (arrows) spreading to periphery of target nodule can be seen, which may be microbubbles filling arterioles.
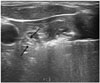
Fig. 5
Artery-first ablation technique.
After ablation of feeding artery, wedge-shaped hypoechoic change (arrows) can be seen. This wedge-shaped hypoechoic area represents infarction in area supplied by feeding artery.

Fig. 6
Hydrodissection technique.
A. Needle of syringe with 5% dextrose (arrow) is inserted posterior to recurrent tumor. B. 5% dextrose is slowly injected (arrows) between target tumor and adjacent critical structures including trachea, esophagus and recurrent laryngeal nerve.
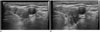
Table 1
Summary of Updates on Devices and Techniques
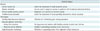
References
1. Spiezia S, Garberoglio R, Milone F, Ramundo V, Caiazzo C, Assanti AP, et al. Thyroid nodules and related symptoms are stably controlled two years after radiofrequency thermal ablation. Thyroid. 2009; 19:219–225.
2. Faggiano A, Ramundo V, Assanti AP, Fonderico F, Macchia PE, Misso C, et al. Thyroid nodules treated with percutaneous radiofrequency thermal ablation: a comparative study. J Clin Endocrinol Metab. 2012; 97:4439–4445.
3. Cesareo R, Pasqualini V, Simeoni C, Sacchi M, Saralli E, Campagna G, et al. Prospective study of effectiveness of ultrasound-guided radiofrequency ablation versus control group in patients affected by benign thyroid nodules. J Clin Endocrinol Metab. 2015; 100:460–466.
4. Jeong WK, Baek JH, Rhim H, Kim YS, Kwak MS, Jeong HJ, et al. Radiofrequency ablation of benign thyroid nodules: safety and imaging follow-up in 236 patients. Eur Radiol. 2008; 18:1244–1250.
5. Ugurlu MU, Uprak K, Akpinar IN, Attaallah W, Yegen C, Gulluoglu BM. Radiofrequency ablation of benign symptomatic thyroid nodules: prospective safety and efficacy study. World J Surg. 2015; 39:961–968.
6. Long B, Li L, Yao L, Chen S, Yi H, Ye X, et al. Combined use of radioiodine therapy and radiofrequency ablation in treating postsurgical thyroid remnant of differentiated thyroid carcinoma. J Cancer Res Ther. 2015; 11:Suppl. C244–C247.
7. Lim HK, Baek JH, Lee JH, Kim WB, Kim TY, Shong YK, et al. Efficacy and safety of radiofrequency ablation for treating locoregional recurrence from papillary thyroid cancer. Eur Radiol. 2015; 25:163–170.
8. Dupuy DE, Monchik JM, Decrea C, Pisharodi L. Radiofrequency ablation of regional recurrence from well-differentiated thyroid malignancy. Surgery. 2001; 130:971–977.
9. Kim JH, Yoo WS, Park YJ, Park DJ, Yun TJ, Choi SH, et al. Efficacy and safety of radiofrequency ablation for treatment of locally recurrent thyroid cancers smaller than 2 cm. Radiology. 2015; 276:909–918.
10. Zhang M, Luo Y, Zhang Y, Tang J. Efficacy and safety of ultrasound-guided radiofrequency ablation for treating low-risk papillary thyroid microcarcinoma: a prospective study. Thyroid. 2016; 26:1581–1587.
11. Kim JH, Baek JH, Sung JY, Min HS, Kim KW, Hah JH, et al. Radiofrequency ablation of low-risk small papillary thyroidcarcinoma: preliminary results for patients ineligible for surgery. Int J Hyperthermia. 2017; 33:212–219.
12. Sun J, Liu X, Zhang Q, Hong Y, Song B, Teng X, et al. Papillary thyroid carcinoma treated with radiofrequency ablation in a patient with hypertrophic cardiomyopathy: a case report. Korean J Radiol. 2016; 17:558–561.
13. Na DG, Lee JH, Jung SL, Kim JH, Sung JY, Shin JH, et al. Radiofrequency ablation of benign thyroid nodules and recurrent thyroid cancers: consensus statement and recommendations. Korean J Radiol. 2012; 13:117–125.
14. Kohlhase KD, Korkusuz Y, Gröner D, Erbelding C, Happel C, Luboldt W, et al. Bipolar radiofrequency ablation of benign thyroid nodules using a multiple overlapping shot technique in a 3-month follow-up. Int J Hyperthermia. 2016; 32:511–516.
15. Korkusuz Y, Erbelding C, Kohlhase K, Luboldt W, Happel C, Grünwald F. Bipolar radiofrequency ablation of benign symptomatic thyroid nodules: initial experience. Rofo. 2016; 188:671–675.
16. Na DG, Lee JH, Kim SM, Lim HK, Baek JH. Unidirectional ablation electrode to minimize thermal injury during radiofrequency ablation: an experimental study in an ex vivo bovine liver model. J Vasc Interv Radiol. 2011; 22:935–940.
17. Turtulici G, Orlandi D, Corazza A, Sartoris R, Derchi LE, Silvestri E, et al. Percutaneous radiofrequency ablation of benign thyroid nodules assisted by a virtual needle tracking system. Ultrasound Med Biol. 2014; 40:1447–1452.
18. Laeseke PF, Sampson LA, Brace CL, Winter TC 3rd, Fine JP, Lee FT Jr. Unintended thermal injuries from radiofrequency ablation: protection with 5% dextrose in water. AJR Am J Roentgenol. 2006; 186:5 Suppl. S249–S254.
19. Lim HK, Lee JH, Ha EJ, Sung JY, Kim JK, Baek JH. Radiofrequency ablation of benign non-functioning thyroid nodules: 4-year follow-up results for 111 patients. Eur Radiol. 2013; 23:1044–1049.
20. Ahn HS, Kim SJ, Park SH, Seo M. Radiofrequency ablation of benign thyroid nodules: evaluation of the treatment efficacy using ultrasonography. Ultrasonography. 2016; 35:244–252.
21. Huh JY, Baek JH, Choi H, Kim JK, Lee JH. Symptomatic benign thyroid nodules: efficacy of additional radiofrequency ablation treatment session--prospective randomized study. Radiology. 2012; 263:909–916.
22. Baek JH, Lee JH, Valcavi R, Pacella CM, Rhim H, Na DG. Thermal ablation for benign thyroid nodules: radiofrequency and laser. Korean J Radiol. 2011; 12:525–540.
23. Ikeda K, Osaki Y, Nakanishi H, Nasu A, Kawamura Y, Jyoko K. Recent progress in radiofrequency ablation therapy for hepatocellular carcinoma. Oncology. 2014; 87:Suppl 1. 73–77.
24. Ha EJ, Baek JH, Lee JH. Ultrasonography-based thyroidal and perithyroidal anatomy and its clinical significance. Korean J Radiol. 2015; 16:749–766.
25. Ha EJ, Baek JH, Lee JH. Moving-shot versus fixed electrode techniques for radiofrequency ablation: comparison in an ex-vivo bovine liver tissue model. Korean J Radiol. 2014; 15:836–843.
26. Baek JH, Ha EJ, Choi YJ, Sung JY, Kim JK, Shong YK. Radiofrequency versus ethanol ablation for treating predominantly cystic thyroid nodules: a randomized clinical trial. Korean J Radiol. 2015; 16:1332–1340.
27. Ha EJ, Baek JH, Lee JH, Kim JK, Shong YK. Clinical significance of vagus nerve variation in radiofrequency ablation of thyroid nodules. Eur Radiol. 2011; 21:2151–2157.
28. Sung JY, Baek JH, Kim KS, Lee D, Yoo H, Kim JK, et al. Single-session treatment of benign cystic thyroid nodules with ethanol versus radiofrequency ablation: a prospective randomized study. Radiology. 2013; 269:293–300.
29. Gharib H, Hegedüs L, Pacella CM, Baek JH, Papini E. Clinical review: nonsurgical, image-guided, minimally invasive therapy for thyroid nodules. J Clin Endocrinol Metab. 2013; 98:3949–3957.
30. Baek JH, Moon WJ, Kim YS, Lee JH, Lee D. Radiofrequency ablation for the treatment of autonomously functioning thyroid nodules. World J Surg. 2009; 33:1971–1977.
31. Deandrea M, Sung JY, Limone P, Mormile A, Garino F, Ragazzoni F, et al. Efficacy and safety of radiofrequency ablation versus observation for nonfunctioning benign thyroid nodules: a randomized controlled international collaborative trial. Thyroid. 2015; 25:890–896.
32. Lee YH, Baek JH, Jung SL, Kwak JY, Kim JH, Shin JH, et al. Ultrasound-guided fine needle aspiration of thyroid nodules: a consensus statement by the Korean Society of Thyroid Radiology. Korean J Radiol. 2015; 16:391–401.
33. Lee SJ, Jung SL, Kim BS, Ahn KJ, Choi HS, Lim DJ, et al. Radiofrequency ablation to treat loco-regional recurrence of well-differentiated thyroid carcinoma. Korean J Radiol. 2014; 15:817–826.
34. Saaiq M, Zaib S, Ahmad S. Electrocautery burns: experience with three cases and review of literature. Ann Burns Fire Disasters. 2012; 25:203–206.
35. Baek JH, Lee JH, Sung JY, Bae JI, Kim KT, Sim J, et al. Complications encountered in the treatment of benign thyroid nodules with US-guided radiofrequency ablation: a multicenter study. Radiology. 2012; 262:335–342.
36. Lee MW. Fusion imaging of real-time ultrasonography with CT or MRI for hepatic intervention. Ultrasonography. 2014; 33:227–239.
37. Makino Y, Imai Y, Igura T, Ohama H, Kogita S, Sawai Y, et al. Usefulness of the multimodality fusion imaging for the diagnosis and treatment of hepatocellular carcinoma. Dig Dis. 2012; 30:580–587.
38. Toshikuni N, Tsutsumi M, Takuma Y, Arisawa T. Real-time image fusion for successful percutaneous radiofrequency ablation of hepatocellular carcinoma. J Ultrasound Med. 2014; 33:2005–2010.
39. Shin JH, Baek JH, Ha EJ, Lee JH. Radiofrequency ablation of thyroid nodules: basic principles and clinical application. Int J Endocrinol. 2012; 2012:919650.
40. Hong MJ, Baek JH, Choi YJ, Lee JH, Lim HK, Shong YK, et al. Radiofrequency ablation is a thyroid function-preserving treatment for patients with bilateral benign thyroid nodules. J Vasc Interv Radiol. 2015; 26:55–61.
41. Sung JY, Baek JH, Jung SL, Kim JH, Kim KS, Lee D, et al. Radiofrequency ablation for autonomously functioning thyroid nodules: a multicenter study. Thyroid. 2015; 25:112–117.
42. Bernardi S, Lanzilotti V, Papa G, Panizzo N, Dobrinja C, Fabris B, et al. Full-thickness skin burn caused by radiofrequency ablation of a benign thyroid nodule. Thyroid. 2016; 26:183–184.
43. Moon WJ, Jung SL, Lee JH, Na DG, Baek JH, Lee YH, et al. Benign and malignant thyroid nodules: US differentiation--multicenter retrospective study. Radiology. 2008; 247:762–770.
44. Shin JH, Baek JH, Chung J, Ha EJ, Kim JH, Lee YH, et al. Ultrasonography diagnosis and imaging-based management of thyroid nodules: revised Korean Society of Thyroid Radiology consensus statement and recommendations. Korean J Radiol. 2016; 17:370–395.
45. Russ G. Risk stratification of thyroid nodules on ultrasonography with the French TI-RADS: description and reflections. Ultrasonography. 2016; 35:25–38.
46. Pillai K, Akhter J, Chua TC, Shehata M, Alzahrani N, Al-Alem I, et al. Heat sink effect on tumor ablation characteristics as observed in monopolar radiofrequency, bipolar radiofrequency, and microwave, using ex vivo calf liver model. Medicine (Baltimore). 2015; 94:e580.
47. Zhu AX, Salem R. Combining transarterial chemoembolization with radiofrequency ablation for hepatocellular carcinoma: one step forward? J Clin Oncol. 2013; 31:406–408.
48. Chok KS, Ng KC, Lam CM, Ng KK, Poon RT, Fan ST. Selective portal vein clamping for radiofrequency ablation of hepatocellular carcinoma with portal vein invasion. J Gastrointest Surg. 2005; 9:489–493.
49. Yoon HM, Baek JH, Lee JH, Ha EJ, Kim JK, Yoon JH, et al. Combination therapy consisting of ethanol and radiofrequency ablation for predominantly cystic thyroid nodules. AJNR Am J Neuroradiol. 2014; 35:582–586.
50. Deandrea M, Limone P, Basso E, Mormile A, Ragazzoni F, Gamarra E, et al. US-guided percutaneous radiofrequency thermal ablation for the treatment of solid benign hyperfunctioning or compressive thyroid nodules. Ultrasound Med Biol. 2008; 34:784–791.
51. Park HS, Baek JH, Choi YJ, Lee JH. Innovative techniques for image-guided ablation of benign thyroid nodules: combined ethanol and radiofrequency ablation. Korean J Radiol. 2017; 18:461–469.
52. Valcavi R, Riganti F, Bertani A, Formisano D, Pacella CM. Percutaneous laser ablation of cold benign thyroid nodules: a 3-year follow-up study in 122 patients. Thyroid. 2010; 20:1253–1261.
53. Døssing H, Bennedbæk FN, Hegedüs L. Long-term outcome following interstitial laser photocoagulation of benign cold thyroid nodules. Eur J Endocrinol. 2011; 165:123–128.
54. Zhao CK, Xu HX, Lu F, Sun LP, He YP, Guo LH, et al. Factors associated with initial incomplete ablation for benign thyroid nodules after radiofrequency ablation: first results of CEUS evaluation. Clin Hemorheol Microcirc. 2016; 11. 14. [Epub]. DOI: 10.3233/CH-16208.
55. Wang B, Han ZY, Yu J, Cheng Z, Liu F, Yu XL, et al. Factors related to recurrence of the benign non-functioning thyroid nodules after percutaneous microwave ablation. Int J Hyperthermia. 2017; 01. 12. [Epub]. DOI: 10.1080/02656736.2016.1274058.
56. Levit E, Bruners P, Günther RW, Mahnken AH. Bile aspiration and hydrodissection to prevent complications in hepatic RFA close to the gallbladder. Acta Radiol. 2012; 53:1045–1048.
57. Chen MH, Yang W, Yan K, Hou YB, Dai Y, Gao W, et al. Radiofrequency ablation of problematically located hepatocellular carcinoma: tailored approach. Abdom Imaging. 2008; 33:428–436.
Supplementary Movie Legends
Movie 1
Virtual needle tracking system. During thyroid radiofrequency ablation, a transient hyperechoic zone is created by vaporization of the nodule tissue impeding monitoring of the electrode tip. On the virtual needle tracking system, the on-screen guidance reveals the direction of travel, with “V” representing the actual location of the electrode tip.
Movie 2
The tip of the electrode is located at the main feeding artery (hypoechoic area), which is ablated for a few seconds. During ablation, echogenic bubbles are generated from the active tip.
Movie 3
Marginal venous ablation technique. When the marginal vein is punctured by the electrode tip and ablated, air bubbles fill the marginal vein of the nodule. Initially, these air bubbles flow rapidly along the marginal vein as long as there is a remaining venous flow. However, when venous flow gradually decreases and eventually ceases, the air bubbles stay inside the veins, indicating complete ablation of the marginal vein.