Abstract
Objective
To evaluate the validity of two abbreviated protocols (AP) of MRI in breast cancer screening of dense breast tissue.
Materials and Methods
This was a retrospective study in 356 participants with dense breast tissue and negative mammography results. The study was approved by the Nanjing Medical University Ethics Committee. Patients were imaged with a full diagnostic protocol (FDP) of MRI. Two APs (AP-1 consisting of the first post-contrast subtracted [FAST] and maximum-intensity projection [MIP] images, and AP-2 consisting of AP-1 combined with diffusion-weighted imaging [DWI]) and FDP images were analyzed separately, and the sensitivities and specificities of breast cancer detection were calculated.
Results
Of the 356 women, 67 lesions were detected in 67 women (18.8%) by standard MR protocol, and histological examination revealed 14 malignant lesions and 53 benign lesions. The average interpretation time of AP-1 and AP-2 were 37 seconds and 54 seconds, respectively, while the average interpretation time of the FDP was 3 minutes and 25 seconds. The sensitivities of the AP-1, AP-2, and FDP were 92.9, 100, and 100%, respectively, and the specificities of the three MR protocols were 86.5, 95.0, and 96.8%, respectively. There was no significant difference among the three MR protocols in the diagnosis of breast cancer (p > 0.05). However, the specificity of AP-1 was significantly lower than that of AP-2 (p = 0.031) and FDP (p = 0.035), while there was no difference between AP-2 and FDP (p > 0.05).
It is recognized that women with dense breasts have a high risk of developing breast cancer (12). Multiple studies have demonstrated mammography (MG) is the standard method for breast screening and can obviously decrease mortality of breast cancer (34). However, it has been proven that the sensitivity of MG detection in dense breasts is much lower than that in non-dense breasts (56). The study by Mandelson et al. (7) showed that the detection rate of breast cancer using MG was only 30% in dense breasts and 80% in non-dense breasts. Ultrasound (US) is not affected by the types of glands and is available for dense breasts, but the dependence on the operator is a source of variability in breast US examination. Hooley et al. (8) demonstrated that US can aid the detection of small mammographically occult breast cancers, but the overall positive predictive value (PPV) is still low.
Magnetic resonance imaging (MRI) has potential value in early breast cancer detection, especially in women with dense breasts (9). Currently, MRI is used as a supplementary examination method of breast screening in high-risk populations (10). Dynamic contrast-enhanced MRI (DCE-MRI) can analyze and accurately localize the lesions from both morphological and hemodynamic aspects, and diffusion-weighted imaging (DWI) provides information about the random motion of water molecules on a cellular level. Several studies have demonstrated that DCE-MRI combined with DWI has a very high sensitivity and specificity for breast cancer detection (1112). However, the high cost, lengthy inspection times, and relatively long interpretation times incurred by MRI have limited its use in breast screening.
Recent studies have evaluated the feasibility of using an abbreviated protocol (AP) of MRI for breast cancer detection (131415), and the results have shown that the AP provided a better detection performance than MG. Therefore, Morris (16) recommended that AP should become a standard protocol for breast cancer screening. However, these studies have not probed the value of DWI for the abbreviated MR protocol. The purpose of this study is to evaluate the usefulness of two APs (AP-1 consisting of the first post-contrast subtracted [FAST] and maximum-intensity projection [MIP] images, and AP-2 consisting of AP-1 combined with DWI) for screening women with dense breast tissue compared to a full diagnostic protocol (FDP), and demonstrate that AP may be an effective and economical method for breast cancer screening.
This retrospective study obtained Institutional Review Board approval from the Nanjing Medical University Ethics Committee. This study included a total of 356 women (mean age, 48.2 ± 4.7 years; range, 30 to 75 years) who had dense breasts examined by MG, and had undergone MR examinations between January 2011 and June 2014. However, all women had not undergone the additional US examination before the MRI examination was performed. Premenopausal women were subjected to imaging between days 7 and 14 of menstruation. All women with MRI positivity had undergone US-guided vacuum-assisted biopsy or surgical excision biopsy, and all women with MRI negativity (81.2%) were followed for 2 years with MG or US.
All breast MRI examinations were performed on a 3T magnet (Area D13, Siemens Healthineers, Erlangen, Germany) using an 8-channel dedicated phased-array breast coil. The subjects assumed a prone position with both breasts symmetrically positioned in the coil. Pre-contrast MR sequences included transverse turbo spin echo (TSE) T1-weighted imaging (T1WI), transverse TSE T2-weighted imaging (T2WI) with fat suppression, and sagittal TSE T2WI with fat suppression. DWI was examined using spin-echo-type single-shot echo planar imaging technique in transverse. The transverse 3D fast spoiled gradient-echo sequence T1WI with fat suppression was then performed in DCE-MRI. Details of the AP and FDP technique are listed in Table 1.
After the first dynamic scan, the contrast agent, gadopentetate dimeglumine, was injected via the cubital vein at a rate of 2.0 mL/s and a dose of 0.2 mmol/kg using a high-pressure syringe. Six phases were continuously scanned in a total scan time of 10 minutes and 24 seconds. The overall acquisition time of the standard MR protocol was 32 minutes. All images were transferred to a syngo MR workplace, and subtraction, MIP and apparent diffusion coefficient images were automatically obtained.
All images were read by two radiologists (with at least 10 years of experience in breast MRI) independently in three steps. First, they each drew a conclusion based on AP-1 (FAST and MIP) images. The time taken for the diagnosis and the results of the diagnosis were recorded according to the Breast Imaging-Reporting and Data System (BI-RADS). Second, AP-2 (FAST + MIP + DWI) images were analyzed to draw the second conclusion, and the interpretation time was recorded. Finally, the FDP images were read to draw the third conclusion. In order to avoid recall bias, the interval of interpretation was projected at least 1 month apart. When the two radiologists' assessments of the three protocols did not match, a third senior radiologist (with 15 years of breast MRI experience) was called to independently analyze the material and decide the final conclusion. In this study, all MRI findings were retrospectively assessed based on the BI-RADS MRI lexicon, and BI-RADS final assessment categories 1, 2, or 3 were considered MRI negativity, and categories 4 or 5 were considered MRI positivity. Using the pathologic diagnosis as the reference standard, the sensitivity, specificity, PPV, and negative predictive value (NPV) of the three methods were calculated.
The SPSS 16.0 statistical software (SPSS Inc., Chicago, IL, USA) was used for statistical analysis. The one-way analysis of variance was used to assess differences of interpretation time among the AP-1, AP-2, and FDP. The McNemar's test was used to assess differences of sensitivity and specificity among AP-1, AP-2, and FDP. A p value less than 0.05 indicated statistical significance.
Of 356 patients, 142 (39.9%) were premenopausal, and 214 (60.1%) were postmenopausal. Six (1.7%) had a personal history of breast cancer, and 29 (8.1%) had a family history of breast cancer.
When using the FDP for diagnoses, 67 lesions were detected in 67 women. Among these 67 women, 46 (68.7%) underwent US-guided vacuum-assisted biopsy, and 21 (31.3%) underwent surgical excision biopsy because of the invisibility of lesions in US. Histological examination demonstrated that 14 of the 67 women had malignant lesions (ductal carcinoma in situ [DCIS], n = 4; invasive ductal carcinoma not otherwise specified, n = 9; mucinous carcinoma, n = 1), and 53 women were diagnosed with benign breast lesions (hyperplasia, n = 18; fibroadenoma, n = 21; cyst, n = 7; intraductal papilloma, n = 6; granuloma, n = 1). The mean diameters of the malignant and benign lesions were 12 mm (range, 5–17 mm) and 11 mm (range, 4–19 mm), respectively. For DCIS, the distribution of nuclear grading was skewed towards high-grade lesions (grade 2, n = 1; grade 3, n = 3). All invasive cancers were small T1N0 cancers (T1a, n = 4; T1b, n = 6), and the distribution of nuclear grading included grade 1 (n = 2), grade 2 (n = 5), and grade 3 (n = 3).
In this study, the average interpretation times with the AP-1 and AP-2 were 37 seconds (range, 22–56 seconds) and 54 seconds (range, 39–77 seconds), respectivly, while the average interpretation time of the FDP was 3 minutes and 25 seconds. A statistically significant difference was found between AP and FDP (p < 0.001).
Among the 67 MRI-positive patients with dense breasts, the FDP used as the reference criterion achieved an accuracy of 100% (14/14) in breast cancer detection. Of the 14 women with breast cancer, AP-1 detected 13 women, and 1 woman with invasive ductal carcinoma was considered “probably benign” and was overlooked. However, this case was detected by using DWI of AP-2 (Fig. 1). The sensitivity, specificity, PPV, and NPV of the three methods are summarized in Table 2. There were no significant differences in sensitivity among AP-1, AP-2, and FDP in the diagnosis of breast cancer (AP-1 vs. AP-2, p = 0.850; AP-1 vs. FDP, p = 0.774; AP-2 vs. FDP, p = 0.816). However, the specificity of AP-1 was significantly lower than that of AP-2 (p = 0.031) and FDP (p = 0.035), and there was no difference between AP-2 and FDP (p = 0.338). For all women with MRI negativity, follow-up imaging examinations showed negative results for 2 years.
Breast experts have committed to finding a safe and effective screening method to reduce the mortality of breast cancer, even while controversies still exist regarding breast cancer screening technology and platforms. To date, screening MG has a limitation in women with dense breast tissue because of the overlap between normal breast tissues and lesions. It has been reported that 50% of the lesions in dense breast tissue cannot be displayed clearly on MG (17). A supplemental method using US contributes to improving the rate of detection of breast cancer in women with dense tissue, but this benefit may be offset by high rates of false positives (8). MRI is considered the most sensitive imaging technique for early detection of breast cancer, and it is particularly effective in the detection of tiny hidden nodules in the breast tissue (18). Berg et al. (19) have shown that, even in women who were negative for breast cancer on MG and US, MRI still detects additional 14.7 cancers per 1000 women. However, it is very time-consuming to perform a conventional DCE-MRI scan, and the high cost also restricts the wide use of MRI in breast screening. To make breast MRI more accessible for routine screening populations, some investigators have evaluated the benefit of an abbreviated breast MRI protocol. Although the selected sequence of AP was varied, several prior studies demonstrated that an AP of MRI was not only a time-saving tool for radiologists and patients, but also had the same diagnostic potential as FDP of MRI (1314152021). However, to our knowledge, there are no published reports about DWI being used as an abbreviated breast MRI protocol.
The images of FAST can remove the interference signals caused by fat and increase the contrast between the lesion and its surrounding tissue, and MIP can clearly display distorted and abnormally proliferated blood vessels of the malignant lesions. The enhanced contrast between the breast lesions and the normal breast parenchyma during the early disease phase is particularly important for breast cancer diagnosis (22). Kuhl et al. (23) investigated 443 cases of MG-negative and asymptomatic women, and found that by merely reviewing the FAST and MIP images, 11 cases of breast cancer were detected with an additional cancer yield of 18.3 per 1000 women. This AP of MRI had a high performance (100% sensitivity and 94.4% specificity) consistent with routine FDP. The present study addressed the usefulness of two APs for breast cancer detection in women with dense breast tissue. The review of FAST, MIP and DWI images from 356 MG-negative and asymptomatic women showed that the sensitivities of AP-1 and AP-2 were equivalent to FDP. However, the specificity of AP-1 was significantly lower than that of AP-2 and FDP, and this finding suggested that there may be some limitations in merely reviewing the FAST and MIP images to detect breast cancer in dense breast tissue.
Diffusion-weighted imaging can evaluate the mobility of water within tissue and provide information about the biological characteristics of tumor tissue. In recent years, several studies have indicated that DWI is effective in differentiating malignant lesions from benign lesions, and can be used to increase the specificity of breast MRI (242526). In this study, AP-2 was adapted by adding DWI to AP-1 to improve the specificity of breast MR. A significant difference in the specificity was observed between AP-1 and AP-2, while there was no difference when AP-2 was compared with FDP. These results demonstrated that DWI provides additional benefits with respect to AP-1 analysis.
The average interpretation time of AP-1 and AP-2 were respectively 37 seconds and 54 seconds, with a statistically significant difference compared with the time of FDP (3 minutes and 25 seconds). This result was similar to previously published reports of Kuhl et al. (23) and Heacock et al. (20), but the interpretation times of AP-1 and AP-2 were shorter than those reported by Moschetta et al. (14) and Grimm et al. (15). The shorter interpretation times of AP are attributed to the time needed for reading other sequences. Therefore, this study showed that FAST and MIP images combined with DWI of MRI may be a more appropriate screening protocol for women with dense breasts. The acquisition time of AP-1 and AP-2 was also shorter than the time of FDP, and it may help to improve the cost-effectiveness of breast screening.
This study has several limitations. First, it was a retrospective and single-institutional study. Second, for the selected population, it did not take into account other high risk factors such as familial risk or personal history of breast cancer besides the dense breast tissue. Finally, it was difficult to detect some small-sized nodules (especially for nodules smaller than 10 mm in diameter) and perform US-guided biopsies of them. In this study, second-look US detected about two thirds of the lesions that could be detected with MRI and the histologic results of US-guided biopsy. These limitations stress the need for further research to determine the value of these abbreviated breast MRI protocols for routine breast screening.
In conclusion, the feasibility of detecting breast cancer in dense breast tissue using abbreviated breast MRI protocols was confirmed. For abundant and dense glands, FAST and MIP images combined with DWI should be recommended to help improve the detection rate of breast cancer and cost effectiveness of breast screening.
Acknowledgments
We are very grateful to colleagues in the Breast Imaging Screening Center at Suzhou Hospital affiliated with Nanjing Medical University who provided us with many valuable data. We also thank Mr. Sunxing Zhang for the English revision.
References
1. Sprague BL, Gangnon RE, Burt V, Trentham-Dietz A, Hampton JM, Wellman RD, et al. Prevalence of mammographically dense breasts in the United States. J Natl Cancer Inst. 2014; 106:dju255. PMID: 25217577.


2. Ko SY, Kim EK, Kim MJ, Moon HJ. Mammographic density estimation with automated volumetric breast density measurement. Korean J Radiol. 2014; 15:313–321. PMID: 24843235.


3. Lee EH, Kim KW, Kim YJ, Shin DR, Park YM, Lim HS, et al. Performance of screening mammography: a report of the alliance for breast cancer screening in Korea. Korean J Radiol. 2016; 17:489–496. PMID: 27390540.


4. Graf O, Berg WA, Sickles EA. Large rodlike calcifications at mammography: analysis of morphologic features. AJR Am J Roentgenol. 2013; 200:299–303. PMID: 23345349.


5. Pollán M, Ascunce N, Ederra M, Murillo A, Erdozáin N, Alés-Martínez J, et al. Mammographic density and risk of breast cancer according to tumor characteristics and mode of detection: a Spanish population-based case-control study. Breast Cancer Res. 2013; 15:R9. PMID: 23360535.


6. Boyd NF, Guo H, Martin LJ, Sun L, Stone J, Fishell E, et al. Mammographic density and the risk and detection of breast cancer. N Engl J Med. 2007; 356:227–236. PMID: 17229950.


7. Mandelson MT, Oestreicher N, Porter PL, White D, Finder CA, Taplin SH, et al. Breast density as a predictor of mammographic detection: comparison of interval- and screen-detected cancers. J Natl Cancer Inst. 2000; 92:1081–1087. PMID: 10880551.


8. Hooley RJ, Greenberg KL, Stackhouse RM, Geisel JL, Butler RS, Philpotts LE. Screening US in patients with mammographically dense breasts: initial experience with Connecticut Public Act 09-41. Radiology. 2012; 265:59–69. PMID: 22723501.


9. Seo M, Cho N, Bae MS, Koo HR, Kim WH, Lee SH, et al. Features of undiagnosed breast cancers at screening breast MR imaging and otential utility of computer-aided evaluation. Korean J Radiol. 2016; 17:59–68. PMID: 26798217.
10. Berg WA. How well does supplemental screening magnetic resonance imaging work in high-risk women? J Clin Oncol. 2014; 32:2193–2196. PMID: 24934782.


11. Moschetta M, Telegrafo M, Rella L, Capolongo A, Stabile Ianora AA, Angelelli G, et al. MR evaluation of breast lesions obtained by diffusion-weighted imaging with background body signal suppression (DWIBS) and correlations with histological findings. Magn Reson Imaging. 2014; 32:605–609. PMID: 24721005.


12. Kul S, Cansu A, Alhan E, Dinc H, Gunes G, Reis A. Contribution of diffusion-weighted imaging to dynamic contrast-enhanced MRI in the characterization of breast tumors. AJR Am J Roentgenol. 2011; 196:210–217. PMID: 21178069.


13. Harvey SC, Di Carlo PA, Lee B, Obadina E, Sippo D, Mullen L. An abbreviated protocol for high-risk screening breast MRI saves time and resources. J Am Coll Radiol. 2016; 13:374–380. PMID: 26521970.


14. Moschetta M, Telegrafo M, Rella L, Stabile Ianora AA, Angelelli G. Abbreviated combined MR protocol: a new faster strategy for characterizing breast lesions. Clin Breast Cancer. 2016; 16:207–211. PMID: 27108218.


15. Grimm LJ, Soo MS, Yoon S, Kim C, Ghate SV, Johnson KS, et al. Abbreviated screening protocol for breast MRI: a feasibility study. Acad Radiol. 2015; 22:1157–1162. PMID: 26152500.
16. Morris EA. Rethinking breast cancer screening: ultra FAST breast magnetic resonance imaging. J Clin Oncol. 2014; 32:2281–2283. PMID: 24958827.


17. Roubidoux MA, Bailey JE, Wray LA, Helvie MA. Invasive cancers detected after breast cancer screening yielded a negative result: relationship of mammographic density to tumor prognostic factors. Radiology. 2004; 230:42–48. PMID: 14695385.


18. Tudorica LA, Oh KY, Roy N, Kettler MD, Chen Y, Hemmingson SL, et al. A feasible high spatiotemporal resolution breast DCE-MRI protocol for clinical settings. Magn Reson Imaging. 2012; 30:1257–1267. PMID: 22770687.


19. Berg WA, Zhang Z, Lehrer D, Jong RA, Pisano ED, Barr RG, et al. Detection of breast cancer with addition of annual screening ultrasound or a single screening MRI to mammography in women with elevated breast cancer risk. JAMA. 2012; 307:1394–1404. PMID: 22474203.


20. Heacock L, Melsaether AN, Heller SL, Gao Y, Pysarenko KM, Babb JS, et al. Evaluation of a known breast cancer using an abbreviated breast MRI protocol: correlation of imaging characteristics and pathology with lesion detection and conspicuity. Eur J Radiol. 2016; 85:815–823. PMID: 26971429.


21. Mango VL, Morris EA, David Dershaw D, Abramson A, Fry C, Moskowitz CS, et al. Abbreviated protocol for breast MRI: are multiple sequences needed for cancer detection? Eur J Radiol. 2015; 84:65–70. PMID: 25454099.


22. Heywang SH, Wolf A, Pruss E, Hilbertz T, Eiermann W, Permanetter W. MR imaging of the breast with Gd-DTPA: use and limitations. Radiology. 1989; 171:95–103. PMID: 2648479.


23. Kuhl CK, Schrading S, Strobel K, Schild HH, Hilgers RD, Bieling HB. Abbreviated breast magnetic resonance imaging (MRI): first postcontrast subtracted images and maximum-intensity projection-a novel approach to breast cancer screening with MRI. J Clin Oncol. 2014; 32:2304–2310. PMID: 24958821.


24. Yoon H, Yoon D, Yun M, Choi JS, Park VY, Kim EK, et al. Metabolomics of breast cancer using high-resolution magic angle spinning magnetic resonance spectroscopy: correlations with 18F-FDG positron emission tomography-computed tomography, dynamic contrast-enhanced and diffusion-weighted imaging MRI. PLoS One. 2016; 11:e0159949. PMID: 27459480.


25. Kızıldağ Yırgın İ, Arslan G, Öztürk E, Yırgın H, Taşdemir N, Gemici AA, et al. Diffusion weighted MR imaging of breast and correlation of prognostic factors in breast cancer. Balkan Med J. 2016; 33:301–307. PMID: 27308074.


26. Sharma U, Sah RG, Agarwal K, Parshad R, Seenu V, Mathur SR, et al. Potential of diffusion-weighted imaging in the characterization of malignant, benign, and healthy breast tissues and molecular subtypes of breast cancer. Front Oncol. 2016; 6:126. PMID: 27242965.


Fig. 1
40-year-old woman with dense breast tissue.
FAST (A) and MIP (B) of MRI show 11-mm homogeneously enhancing mass with circumscribed margins in left breast, which was classified as probably benign (BI-RADS 3). However, DWI (C) shows high signal (low signal on apparent diffusion coefficient), which was classified as malignant (BI-RADS 4), and biopsy yielded invasive ductal carcinoma.
BI-RADS = Breast Imaging-Reporting and Data System, DWI = diffusion-weighted imaging, FAST = first post-contrast subtracted, MIP = maximum-intensity projection
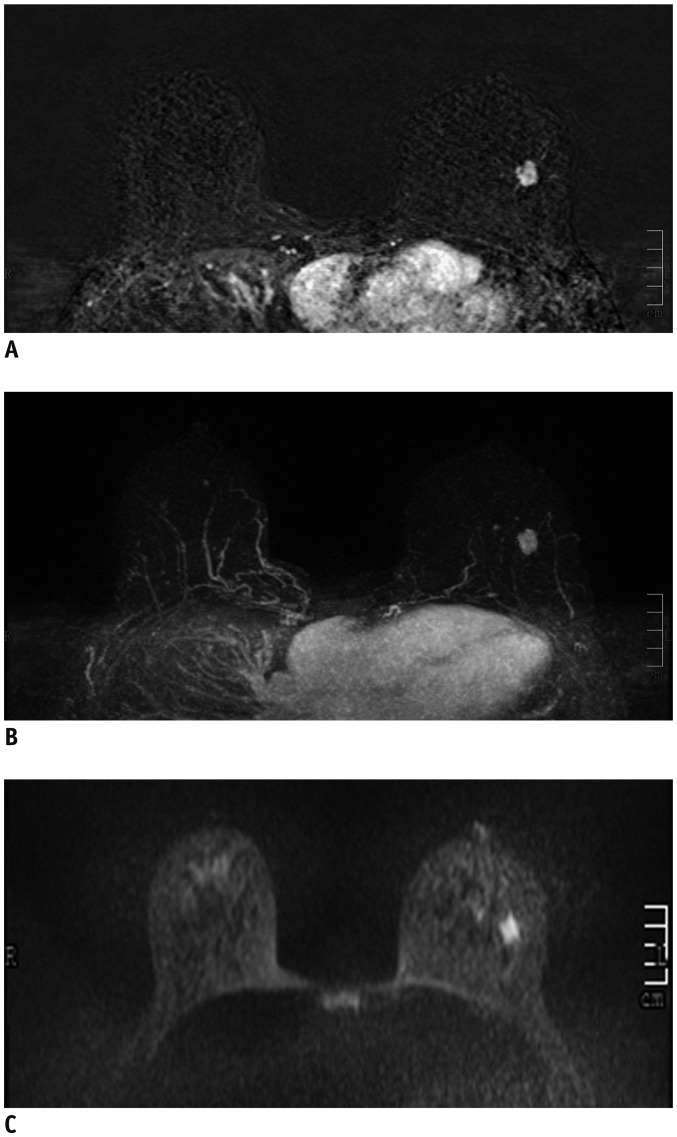
Table 1
Details of Study Protocol Pulse-Sequence Parameters
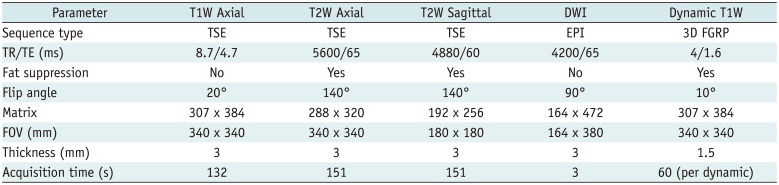
Table 2
Comparison of Capabilities of AP and FDP in Diagnosis of Breast Cancer
