Abstract
Oncology is a rapidly evolving field with a shift toward personalized cancer treatment. The use of therapies targeted to the molecular features of individual tumors and the tumor microenvironment has become much more common. In this review, anti-angiogenic and other molecular targeted therapies are discussed, with a focus on typical and atypical response patterns and imaging manifestations of drug toxicities.
Oncology practice has dramatically changed in the last ten to fifteen years (1). Genomic assessment of tumors has become commonplace, and there has been a paradigm shift toward the use of targeted therapies directed against the specific molecular drivers of tumors in individual patients where possible. This personalized approach to cancer treatment has profoundly altered patient management, in terms of the available treatment armamentarium, logical sequencing of agents, and optimized use of the targeted therapies to maximize survival and limit toxicities. At many timepoints along the care continuum, imaging interpretation plays a critical role, especially in terms of assessment of patients' response to molecular targeted therapy (MTT) or lack thereof, and in detection of disease and treatment-related complications.
As oncology care becomes increasingly diverse and complex, it is a challenge to remain up-to-date on the state of the art and standards within the field. For example, there are now seven vascular endothelial growth factor (VEGF)-targeted agents approved for use in advanced or metastatic renal cell carcinoma (RCC) by the United States Food and Drug Administration (FDA), two of which were approved in the last six months. Innovations in genomic characterization of cancer, targeted drug discoveries and new approvals occur continuously, and it is difficult to keep abreast of changes directly impacting cancer patients treated in both academic and community practices.
To fully integrate into the multidisciplinary team and provide best care for patients, radiologists must communicate with oncologists using a common language. Therefore, we must be familiar with routinely employed MTTs and the range of imaging findings associated with their use. The radiologist may be the first provider to suggest an atypical response pattern or a subclinical toxicity, which can significantly alter study interpretation and contribute to patient management. The purpose of this review is to provide a summary of the commonly used MTTs relevant to imaging interpretation, including the rationale of the various anti-angiogenic and non-anti-angiogenic treatments, indications for use, response assessment methods and characteristic toxicities. Immune checkpoint blocker agents will not be addressed in this report since these represent a separate class of targeted immune therapies covered separately in this special issue.
Folkman (2) described the link between solid tumor growth and neovascularization in 1971, and he proposed the term “anti-angiogenesis,” referring to “the prevention of new vessel sprouts from penetrating into an early tumor implant” as a means of cancer treatment. Since that time, it has been shown that a number of cancers, including colon, renal and lung, are associated with increased VEGF expression, and that VEGF expression levels have prognostic significance in many instances (3456). A number of anti-angiogenic agents, also known as VEGF-targeted agents, have since become FDA-approved, and have been shown to prolong progression-free and overall survival in various malignancies. There are multiple subtypes of VEGF (A, B, C, D, and placental growth factor) and multiple VEGF-receptor (VEGFR) tyrosine kinases (VEGFR 1, 2, and 3), and antiangiogenic drugs may target one or more specific circulating VEGF subtypes or VEGFR.
The first angiogenesis inhibitor to be approved by the FDA, bevacizumab (Avastin®; Genentech Inc., South San Francisco, CA, USA), is a recombinant, humanized monoclonal antibody to circulating VEGF-A. Approved indications for use of bevacizumab include, but are not limited to: metastatic colorectal cancer, unresectable or metastatic non-squamous, non-small cell lung cancer, metastatic RCC, recurrrent glioma, recurrent or metastatic cervical cancer, as well as platinum-resistant ovarian or primary peritoneal cancer, often in combination with chemotherapy (789101112). Ziv-aflibercept (Zaltrap; Sanofi US and Regeneron Pharmaceuticals, Inc., Bridgewater, NJ and Tarrytown, NY, USA) is an agent which also binds VEGF, representing a humanized recombinant fusion protein comprised of portions of the extracellular domains of VEGF receptors fused to the Fc portion of human immunoglobulin G1 (13). Ziv-aflibercept is approved for use in metatstatic colorectal cancer in combination with 5-fluorouracil, leucovorin, and irinotecan following treatment with an oxaliplatin-based regimen (14). Small molecule inhibitors of receptor tyrosine kinases inhibit (TKI) the VEGF-receptor and other transmembrane kinases, and include sunitinib (Sutent®; Pfizer, New York, NY, USA), sorafenib (Nexavar®; Bayer Pharmaceuticals Corporation, Wayne, NJ and Onyx Pharmaceuticals Inc., Emeryville, CA, USA), pazopanib (Votrient®; GlaxoSmithKline, Middlesex, United Kingdom) and others (15). TKIs are approved in a variety of malignancies, including metastatic RCC, hepatocellular carcinoma and gastrointestinal stromal tumor, amongst others (161718).
Since anti-angiogenic agents exert their effects via distinct mechanisms of action, it follows that response patterns differ from those seen with traditional cytotoxic therapies (Fig. 1). Anti-angiogenic agents have been described as likely “cytostatic” as opposed to “cytocidal”, and tumor stabilization has been acknowledged as an endpoint of treatment (19). According to the Response Evaluation Criteria in Solid Tumors (RECIST), which was developed during the age of cytotoxic chemotherapy, a 30% decrease in the sum long axis diameter of target lesions is required for partial response (20). In the era of anti-angiogenic targeted therapy, response rates according to RECIST are sometimes low, ranging from 10–40% in RCC trials, for example (917). A rather large number of RCC patients achieve a best response of “stable disease” on treatment, a heterogeneous categorization including patients who may or may not experience prolonged progression free survival (21). Several drugs have gained FDA-approval for indications on the basis of prolonged progression-free survival or “clinical benefit” in treated patients.
Kerbel and Folkman (22) acknowledged the challenges in determining the most reliable end points indicative of therapeutic efficacy in anti-angiogenic treatments. Many investigators have examined a variety of alternative response criteria, aimed at optimizing response assessment in drug and cancer-specific treatment settings (23). For example, the Response Assessment in Neuro-Oncology (RANO) criteria have been developed to assess high grade gliomas treated with anti-angiogenic agents, and to address limitations of the previously used Macdonald criteria, including underestimation of non-enhancing tumor and overestimation of response related to changes in enhancement (24). Similarly, computed tomography (CT)-based morphologic criteria have been developed to address morphologic changes in liver metastases which correlate with pathologic changes and overall survival in patients with metastatic colorectal cancer treated with bevacizumab-containing regimens (Fig. 2) (25). Changes of cavitation have been incorporated into modified RECIST (mRECIST) assessment of non-small-cell lung cancer treated with an anti-angiogenic agent (26). In the setting of hepatocellular carcinoma treated with sorafenib, adjustments to RECIST include measurement of the arterially enhancing components of tumors in mRECIST and other changes in irregular tumors using Response Evaluation Criteria in Cancer of the Liver (Fig. 3) (2728). In RCC, a variety of alternative assessment methods have been tested to better differentiate responders and non-responders to anti-angiogenic treatments, including Choi criteria, Morphology, Attenuation, Size and Structure criteria, and alternate tumor shrinkage thresholds (21293031).
Anti-angiogenic or VEGF-targeted therapies are associated with several treatment-specific complications of which radiologists must be aware, since imaging manifestations of the drug toxicity may occur before a patient develops symptoms. Some of the most important, potentially life-threatening adverse events associated with anti-angiogenic therapy are bowel perforation, pneumatosis and/or tumor bowel fistula, which may have subtle or overt imaging appearances (Fig. 4). While these events are infrequent, occurring in 1.7% of 1442 patients treated with bevacizumab according to one report (32), prompt detection is important, as pneumatosis and perforation are often managed conservatively via discontinuation of therapy and supportive care. The radiologist may be the first provider to encounter these toxicities, as 70.8% of cancer patients with pneumatosis and/or bowel perforation were asymptomatic in one study (33). Other potential abdominal complications in anti-angiogenic treatment include colitis, cholecystitis, pancreatitis and hepatic steatosis (34).
Hemorrhage, thrombosis and hypertension are other potential toxicities related to VEGF-targeted therapy. Hemorrhage is reported in up to 30% of patients with various cancers treated with bevacizumab, severe in fewer than 5% of patients (35). While common presentations include epistaxis, gastrointestinal bleeding and hemoptysis, it is particularly relevant for radiologists to be cognizant of potential intratumoral hemorrhage, which can result in increased size of metastases and lead to erroneous interpretation of progression (pseudoprogression). A clue to potential pseudoprogression is development of fluid-fluid levels in metastases, and MRI is a helpful problem solving tool to detect hemorrhagic (T1-hyperintense) contents in challenging cases. Arterial thromboembolic events are increased in patients treated with VEGF-targeted therapies, with overall relative risk of 1.46 in bevacizumab treated patients and 3.03 in sunitinib or sorafenib treated patients, compared to controls (3637). Risk of venous thromboembolic events in this setting is considered controversial, with increased risk in one meta-analysis and no increased risk in another (3839). Hypertension is another adverse effect and potential biomarker in patients treated with anti-angiogenic agents, since improved response rates and survival parameters have been documented in patients who develop hypertension on treatment (40). Posterior reversible encephalopathy syndrome is a rare complication of anti-angiogenic therapy (41).
When reporting imaging studies of patients treated with anti-angiogenic or VEGF-targeted therapy, it is important for the radiologist to pay close attention to the study indication (including disease and specific therapy), to use appropriate cancer-specific and/or treatment-specific response criteria when available, to consider the possibility of an atypical response, and to evaluate for known toxicities of the treatment regimen on imaging. Reporting of cancer imaging studies can be facilitated by the use of cancer imaging radiology report templates (42). For example, in a patient with metastatic colon cancer to liver treated with a bevacizumab-containing regimen, it is relevant to consider response according to CT-based morphologic criteria. Atypical response patterns should be considered, including potential increased size but decreased density/greater homogeneity in liver metastases, and/or apparent new lesions which could have been isodense at baseline (43). In difficult cases, correlation may be made with tumor markers (e.g., carcinoembryonic antigen) and patient's performance status through discussion with the referring oncologist. In addition, it is important to scrutinize the imaging study for treatment related complications and toxicities, such as pneumatosis, thromboembolism and hepatic steatosis.
As reviewed, anti-angiogenic agents are approved for use in a variety of malignancies. Also during the last decade, multiple other MTTs have been developed to target molecular pathways other than/in addition to the VEGF-axis. Many of these are approved for use in cancer-specific treatment settings, with or without associated documentation of a cancer's driver mutation via FDA-approved genetic tests. Using lung, renal and breast cancer as representative examples, several commonly used MTTs will be discussed, including an overview of their treatment rationale, expected response patterns and imaging manifestations of toxicity.
Epidermal growth factor receptor (EGFR) tyrosine kinase is a protein involved in cell proliferation, apoptosis, angiogenesis and invasion, overexpressed in non-small-cell lung cancer (44). Activating mutations in the EGFR-tyrosine kinase domain have been documented in approximately 10% of non-small cell lung cancer patients of North American/Western European descent and 25–50% of non-small cell lung cancer patients of Asian descent (45). Presence of an activating mutation in EGFR has been associated with dramatic response to targeted EGFR TKI gefitinib (Iressa; AstraZeneca, Pharmaceuticals, Wilmington, DE, USA) and erlotinib (Tarceva; Genentech, South San Francisco, CA, USA) (Fig. 5) (464748). This discovery, reported in 2004, was a major advance in the lung cancer treatment paradigm; prior to this, all patients with advanced non-small cell lung cancer were treated with a platinum-based chemotherapy regimen. In the new era, patient's tumors are prospectively tested for sensitizing mutations amenable to targeted treatments such as EGFR TKIs. Clinical features associated with the sensitizing mutations and responsiveness to EGFR TKIs include Asian descent, female sex and never smoker status.
Another genomically characterized lung cancer involves anaplastic lymphoma kinase (ALK) rearrangement, occurring in 2–7% of non-small-cell lung cancer patients (49). In this setting, the echinoderm microtubule-associated protein-like 4 (EMF4) gene is fused to ALK gene, and this encodes a constitutively active kinase. Clinical features associated with ALK rearranged lung cancer include young age and never to light smoking history. Patients with ALK rearrangements are highly sensitive to ALK inhibitors such as crizotinib (Xalkori; Pfizer, New York, NY, USA) and ceritinib (Zykadia; Novartis, East Hanover, NJ, USA) (Fig. 6) (4950).
In lung cancer patients with selected driver mutations, response to targeted therapy is often dramatic. For example, response rates to EGFR TKIs are reportedly 55–75% in the first line setting, and median progression free survival is on the order of 9 months (515253). Similarly, the phase III overall response rate to crizotinib was 65% and median progression free survival approximately 8 months (54). Conventional size based response criteria (such as RECIST) are typically employed in these settings, which capture response in many cases, though other methods of assessment including CT tumor volumetry have been proposed (55).
As many patients demonstrate dramatic tumor shrinkage, the nadir target lesion diameter may be quite small and modest increases in diameter upon acquired resistance (for example, via acquired T790M mutation or another mechanism in EGFR mutant patients) may represent RECIST disease progression (23). If targeted therapy is withdrawn, a flare phenomenon can occur, with dramatic increase in tumor burden upon withdrawal of the targeted agent (Fig. 5C, D) (56). Many in the field realize the clinical benefit to continuing EGFR-targeted therapy beyond RECIST progression in some patients, either alone or in combination with chemotherapy. Alternative criteria are sought to objectively differentiate patients with slow progression amenable to targeted agent continuation from those without treatment benefit (5758). Tumor volume models to define tumor growth have been investigated in this regard (59). The appropriate time to initiate a treatment change will become even more complicated as therapies targeted to resistance mechanisms become available. For example, osimertinib (Tagrisso; AstraZenecae, Pharmaceuticals, Wilmington, DE, USA) was approved for patients with acquired EGFR T790M mutation-positive metastatic non-small cell lung cancer in November 2015 (60).
When reporting imaging studies of patients with genomically characterized lung cancer, it is often helpful for the radiologist to know the specific mutation and targeted therapy. Furthermore, it may be useful to know the date of the baseline scan, the tumor burden at baseline, as well as have an awareness of the nadir tumor burden, if reached. Familiarity with the treatment course and the expected time of resistance development may help to detect subtle changes in tumor size and shape indicative of early resistance.
It is also important to assess such patients for radiologic evidence of drug toxicity, including pneumonitis in those treated with EGFR TKI or ALK inhibitors. Four patterns of pneumonitis have been associated with EGFR TKI according to one report, including: 1) nonspecific area of groundglass attenuation, 2) multifocal airspace consolidation, 3) patchy ground-glass attenuation accompanied by interlobar septal thickening, and 4) extensive bilateral ground-glass attenuation or airspace consolidation with traction bronchiectasis (61). Pattern A was the most common in this study, involving 44% of 70 patients with gefitinib-related lung disease, while pattern D was associated with the highest mortality, involving 75% of patients in this category. In phase III clinical trials of erlotinib and crizotinib, interstitial lung disease/pneumonitis resulted in 5 deaths (1%) and 2 deaths (~1%) in the treatment groups, respectively (5462).
Radiologists should also be aware of other potential imaging manifestions of EGFR TKI toxicity, including diarrhea/colitis, pneumatosis and periportal edema related to hepatic failure (63). Diarrhea/colitis is commonly reported, evidenced by fluid filled colon, dilation, wall thickening and/or mesenteric hyperemia (63). In contrast, pneumatosis and periportal edema related to hepatic failure are rare events (63). It is worthwhile to mention that each of the EGFR TKIs toxicities can be seen in patients treated with these agents in other cancer settings (e.g., erlotinib treatment of pancreatic cancer; cetuximab (Erbitux; Bristol-Myers Squibb, New York, NY, USA) treatment of advanced squamous cell carcinoma of the head and neck) (6465). Development of complex renal cysts has been associated with crizotinib therapy, and cysts regress when therapy is discontinued (66). Rapid onset hypogonadism with low testosterone has also been reported with crizotinib therapy (Fig. 6C, D) (67). A drug-based imaging toxicity checklist or template may assist radiologists in reporting pertinent findings (42).
In RCC, the VEGF-axis is a common therapeutic target due to inactivation of the von Hippel Lindau gene in the majority of clear cell RCCs, leading to accumulation of hypoxia inducible factor and overexpression of VEGF. However, the phosphatidylinositol-3-kinase-Akt-mammalian target of rapamycin (mTOR) pathway is another active intracellular molecular signaling pathway in RCC amenable to targeted treatment. mTOR is a serine/threonine kinase, and its activation ultimately promotes protein synthesis and cell survival (6869). Everolimus (Afinitor®; Novartis, East Hanover, NJ, USA) and temsirolimus (Torisel® Pfizer, New York, NY, USA) are inhibitors of mTOR approved in metastatic RCC (7071). Both agents were associated with modest response rates ranging from 1–9% in phase III trials, though progression free survival was prolonged by both agents and overall survival prolonged by the latter (7071). Everolimus is also approved for use in subependymal giant cell astrocytoma, advanced hormone receptor–positive human epidermal growth factor receptor 2 (HER2)-negative breast cancer, and pancreatic neuroendocrine tumors. Clinical trials of everolimus in Waldenstrom's macroglobulinemia have been reported, with promising results (72).
Given the high prevalence of stable disease according to RECIST in RCC patients treated with mTOR inhibitors, alternative criteria have been sought to better differentiate responders and non-responders. According to an analysis of phase III study data in everolimus-treated patients, a reduction of greater than 5% in the sum long diameter measurements of target lesions predicted benefit in progression free survival better than partial response (30% reduction) (73). However, it is possible that 5% reduction in the sum long diameter measurements of target lesions is within the range of measurement variability, therefore, the clinical utility of this threshold is questionable (74). Modified Choi criteria have been applied to RCC patients treated with everolimus, and modified Choi assessments in three dimensions enabled identification of patients with progression free survival benefit from everolimus according to one study (75). Given the limitations and the complexity of these alternative criteria, response assessment remains largely according to RECIST in this clinical setting.
Imaging manifestations of mTOR inhibitor toxicity have been documented. Radiographic evidence of pneumonitis occurs in up to a third of patients, represented by ground-glass opacities and consolidation (Fig. 7) (767778). According to Maroto et al. (76), 31% of patients with radiographic evidence of pneumonitis were symptomatic (with cough, shortness of breath and/or oxygen requirement), highlighting the important role of the radiologist in detecting early/asymptomatic lung toxicity. Pneumonitis has been proposed as a marker of therapeutic benefit, since patients in one report with imaging evidence of pneumonitis more frequently demonstrated stable disease according to RECIST than those without pneumonitis (77). In patients with other malignancies treated with mTOR inhibitors, as many as 58% of patients with Waldenstrom's macroglobulinemia demonstrated imaging findings of pneumonitis, while 21% of patients with pancreatic neuroendocrine tumors did, most commonly manifesting as cryptogenic organizing pneumonia and non-specific interstitial pneumonia patterns (7980). Cholecystitis, pancreatitis and enteritis are less commonly seen mTOR toxicities on imaging (818283).
While molecular targeted therapies are commonly employed in a variety of advanced/metastatic cancers, targeted treatment in breast cancer may be applied in neoadjuvant and adjuvant settings, as well as in advanced/metastatic disease. According to the National Comprehensive Cancer Network (NCCN) Clinical Practice Guidelines in Oncology, adjuvant therapy is recommended in patients with tumors greater than 1 cm or with lymph node involvement (84). Traditional cytotoxic chemotherapies including taxanes and anthracyclines are commonly used in first line treatment (84). In addition to stage, hormone receptor status and HER2 status are also important factors influencing treatment, and hormonal and/or targeted agents are part of the armamentarium.
About two-thirds of breast cancers are hormone receptor positive cancers. These are amenable to treatment with hormonal agents, including selective estrogen receptor modulators (SERMs), like tamoxifen, and/or aromatase inhibitors (AIs), including anastrozole, letrozole, exemestane. SERMs act by an anti-estrogenic effect in the breast tissue and AIs reduce conversion of androgens to estrogens, the latter commonly used in post-menopausal patients. Given their relative safety profile, adjuvant hormonal therapy may be continued for 5–10 years after diagnosis. In metastatic disease, hormonal agents may be used in the first line setting in the absence of rapidly progressing visceral metastases, in light of the preferable side effect profiles of these drugs compared to cytotoxic chemotherapy.
Hormonal agents may be used alone or in combination with other agents, including MTTs (Fig. 8). For example, in February 2015, the cyclin dependent kinase (CDK) inhibitor palbociclib (Ibrance; Pfizer) was approved in combination with letrozole for initial treatment of advanced hormone receptor positive, HER2 negative breast cancer in postmenopausal patients (85). Palbociclib is a small molecule inhibitor of CDK4 and 6, which blocks progression of cells from the G1 into S phase of the cell cycle, resulting in growth arrest. In the phase II study of letrozole plus palbociclib compared to letrozole alone, median progression free survival was doubled in patients treated with combination therapy (20.2 months vs. 10.2 months, p = 0.0004) and partial response rates were higher among patients with measurable disease treated with combination therapy (54% vs. 39%, p = 0.047) (85). In February 2016, palbociclib was approved in combination with fulvestrant, a complete estrogen receptor antagonist, for postmenopausal patients with hormone receptor positive, HER2 negative advanced breast cancer after progression on endocrine therapy (86). The mTOR inhibitor everolimus is also approved in combination with exemestane for patients with advanced, hormone receptor positive, HER2 negative breast cancer who have progressed on letrozole or anastrozole (87). Response to the combination of hormonal agents and MTTs is typically assessed according to RECIST criteria in breast cancer.
Toxicities must be considered according to the specific hormonal agent and/or MTT administered. For example, in the case of SERM or tamoxifen use, proestrogenic effects are observed in the uterus, including benign entities (endometrial polyps, hyperplasia, adenomyosis, and fibroids) and malignant disease (endometrial carcinoma, and sarcoma) (88). Tamoxifen use is also associated with hepatic steatosis. Bone loss is a well recognized side effect of AIs (89). Pneumonitis can be observed in patients treated with mTOR inhibitors.
Human epidermal growth factor receptor 2 positive breast cancer represents up to 20% of breast cancer cases. HER2 positive breast cancers overexpress HER2, also known as ErbB2, a receptor tyrosine kinase involved in cell growth and proliferation. HER2 positivity is a biomarker of disease aggressiveness but is also predictive of responsiveness to HER2 targeted treatments. Trastuzumab (Herceptin; Genentech Inc., South San Francisco, CA, USA) is a monoclonal antibody targeted to HER2 used in adjuvant and metastatic breast cancer treatment of HER2 positive tumors, often in combination with other drugs (90). Toxicities associated with trastuzumab therapy include cardiotoxicity which may be identified on echocardiogram or on multiple-gated acquisition scan. Pertuzumab (Perjeta; Genentech Inc.) is another HER2 targeted therapy, aimed at inhibiting HER2 dimerization. It is approved in combination with trastuzumab and docetaxel in the neoadjuvant and metastatic settings (91).
Other targeted therapies are FDA-approved for patients who have progressed on trastuzumab-containing regimens. In 2007, the dual HER2/EGFR inhibitor lapatinib (Tykerb; GlaxoSmithKline, Middlesex, United Kingdom) was approved in HER2 overexpressing breast cancer in combination with capecitabine in patients who have progressed on prior therapy containing trastuzumab (92). In 2010, lapatinib was approved in combination with letrozole in hormone receptor positive, HER2 positive disease. Lapatinib is a small molecule which may penetrate the blood brain barrier, an important feature in HER2 positive disease, since one third or more patients with metastatic HER2 positive breast cancer develop brain metastases (93). In 2013, the drug-antibody conjugate known as TDM-1 or ado-trastuzumab emtansine (Kadcyla; Genentech Inc.) was approved for patients with HER2 positive disease after progression on trastuzumab and a taxane, separately or in combination (94). In this therapy, DM-1 is an antimicrotubule agent which is linked to trastuzumab for selective delivery into cancer cells. Response assessment is according to RECIST in the various HER2 targeted treatments in breast cancer.
Interpretation of imaging studies in oncology patients has become progressively more complex in the context of evolving genomic cancer characterization and increasing number and types of therapies available to patients. Radiologists should be familiar with anti-angiogenic treatments and the wide variety of non-anti-angiogenic molecular targeted therapies associated with typical and atypical patterns of tumor response, resistance, growth and toxicity. It is a formidable task to keep abreast of developments in oncology, but it is necessary to provide optimal diagnoses, render appropriate response assessments, and identify important drug toxicities which may impact patient management. Cancer and/or drug specific radiology report templates may assist radiologists in this regard.
Figures and Tables
Fig. 1
Typical response of metastatic clear cell renal cell carcinoma to VEGF-targeted therapy and subsequent tumor growth off of treatment.
In this 55-year-old woman, baseline contrast-enhanced CT prior to start of treatment demonstrates vascularized right hilar metastasis (arrow, A), pelvic serosal deposit (arrow, B) and left iliac bone metastasis (arrowhead, B). After 10 weeks of axitinib treatment, each metastasis is decreased in size and density (C, D). Patient subsequently developed wound infection in her right lower extremity, resulting in hold in axitinib treatment. After 6 weeks of holding treatment (and 18 weeks from first follow-up CT), right hilar mass (E), and pelvic serosal metastasis (arrow, F) are increased in size and enhancement, representing tumor growth. In addition, rim enhancement is newly seen involving left iliac bone lesion (arrowhead), also representing increasing tumor at site. VEGF = vascular endothelial growth factor
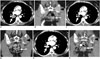
Fig. 2
Atypical response to VEGF-targeted therapy in colon cancer.
In this 57-year-old woman with metastatic colon cancer, baseline contrast-enhanced CT (A) demonstrates multiple heterogeneous liver metastases. Serum CEA at baseline measured 2049. After 12 weeks of therapy with folinic acid, fluorouracil, oxaliplatin (FOLFOX) and avastin, multiple liver metastases have increased in size but have decreased in density (B), showing greater homogeneity and better defined margins. Serum CEA at time of 12 week follow-up CT measured 1126. Findings at follow-up CT represent response according to CT-morphologic criteria. CEA = carcinoembryonic antigen, VEGF = vascular endothelial growth factor
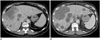
Fig. 3
Hepatocellular cancer (HCC) with response to VEGF-targeted therapy according to mRECIST.
Baseline contrast-enhanced CT (A) demonstrates vascularized, enhancing tumor thrombus in right anterior and posterior portal vein branches (arrows) in this 59-year-old man with multifocal HCC. Follow-up contrast-enhanced CT after 2 months of VEGF-targeted therapy shows loss of hypervascularity of tumor thrombus (B), in keeping with response to therapy according to mRECIST. mRECIST = modified Response Evaluation Criteria in Solid Tumors, VEGF = vascular endothelial growth factor
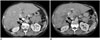
Fig. 4
Gastrointestinal stromal tumor with response to sunitinib and tumor bowel fistula development.
In this 76-year-old woman, baseline axial contrast-enhanced CT (A) demonstrates mass adjacent to small bowel in left lower quadrant (white arrow). Follow-up contrast-enhanced CT (B) demonstrates new oral contrast within tumor lesion, contiguous with small bowel lumen (black arrow).
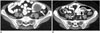
Fig. 5
EGFR-mutant lung cancer with response to treatment, development of resistant disease, flare off of therapy and subsequent response to second-line treatment.
Baseline CT in this 62-year-old never smoker demonstrates large left upper lobe perihilar mass with interlobular septal thickening emanating from mass (A), multistation lymphadenopathy is not shown. CT follow-up 5 months after erlotinib treatment shows dramatic shrinkage of left perihilar mass (B). Subsequent follow-up CT 8 months after start of treatment shows mild increase in left perihilar tumor indicative of resistant disease (not shown). Lymph node biopsy confirmed T790M secondary resistance mutation. Erlotinib therapy was discontinued in anticipation of clinical trial participation. One month after erlotinib discontinuation, patient presented with shortness of breath, cough and chest pain. CT chest revealed flare phenomenon, represented by marked increase in tumor burden in both lungs (C, D). Erlotinib was re-initiated with carboplatin and pemetrexed chemotherapy, and tumor burden significantly decreased 8 weeks later (E, F). EGFR = epidermal growth factor receptor
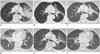
Fig. 6
EMF4-ALK rearranged lung cancer response to crizotinib and associated toxicity.
Baseline contrast-enhanced CT in this 50-year-old man demonstrates large right lateral chest wall mass (arrow, A) which responded dramatically to crizotinib therapy, with little if any residual mass after 3 months of treatment (B). Thoracic vertebral bodies were normal in height at baseline (C). Demineralization and compression deformities developed during course of treatment (arrow, D), known toxicity of crizotinib related to rapid onset hypogonadism and low testosterone. ALK = anaplastic lymphoma kinase, EMF4= echinoderm microtubule-associated protein-like 4
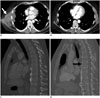
Fig. 7
Response to mTOR-inhibitor treatment in Waldenstrom's macroglobulinemia and lung toxicity.
Baseline contrast-enhanced coronal CT images in 59-year-old woman show retroperitoneal lymphadenopathy (black arrows) and mild splenomegaly (A). Five months after start of treatment, coronal contrast-enhanced CT shows decreased adenopathy and splenomegaly (B). At baseline, clear lung bases were present (C). On five month follow-up CT (D), new ground-glass and reticular opacities developed in lung bases (white arrows), in keeping with mTOR-associated pneumonitis. Patient was asymptomatic. mTOR = mammalian target of rapamycin
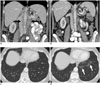
Fig. 8
Response to aromatase inhibitor and cyclin dependent kinase inhibitor in metastatic hormone receptor+, HER2 negative breast cancer.
In this 29-year-old woman, baseline coronal CT (A) demonstrates multiple liver metastases (arrow). Follow-up coronal contrast-enhanced CT (B) demonstrates decreased size of multiple liver lesions (arrow) representing response to treatment. HER2 = human epidermal growth factor receptor 2
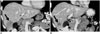
References
1. Mendelsohn J. Personalizing oncology: perspectives and prospects. J Clin Oncol. 2013; 31:1904–1911.
2. Folkman J. Tumor angiogenesis: therapeutic implications. N Engl J Med. 1971; 285:1182–1186.
3. Lee JC, Chow NH, Wang ST, Huang SM. Prognostic value of vascular endothelial growth factor expression in colorectal cancer patients. Eur J Cancer. 2000; 36:748–753.
4. Berger DP, Herbstritt L, Dengler WA, Marmé D, Mertelsmann R, Fiebig HH. Vascular endothelial growth factor (VEGF) mRNA expression in human tumor models of different histologies. Ann Oncol. 1995; 6:817–825.
5. Krieg M, Haas R, Brauch H, Acker T, Flamme I, Plate KH. Upregulation of hypoxia-inducible factors HIF-1alpha and HIF-2alpha under normoxic conditions in renal carcinoma cells by von Hippel-Lindau tumor suppressor gene loss of function. Oncogene. 2000; 19:5435–5443.
6. Ohta Y, Endo Y, Tanaka M, Shimizu J, Oda M, Hayashi Y, et al. Significance of vascular endothelial growth factor messenger RNA expression in primary lung cancer. Clin Cancer Res. 1996; 2:1411–1416.
7. Hurwitz H, Fehrenbacher L, Novotny W, Cartwright T, Hainsworth J, Heim W, et al. Bevacizumab plus irinotecan, fluorouracil, and leucovorin for metastatic colorectal cancer. N Engl J Med. 2004; 350:2335–2342.
8. Sandler A, Gray R, Perry MC, Brahmer J, Schiller JH, Dowlati A, et al. Paclitaxel-carboplatin alone or with bevacizumab for non-small-cell lung cancer. N Engl J Med. 2006; 355:2542–2550.
9. Yang JC, Haworth L, Sherry RM, Hwu P, Schwartzentruber DJ, Topalian SL, et al. A randomized trial of bevacizumab, an anti-vascular endothelial growth factor antibody, for metastatic renal cancer. N Engl J Med. 2003; 349:427–434.
10. Tewari KS, Sill MW, Long HJ 3rd, Penson RT, Huang H, Ramondetta LM, et al. Improved survival with bevacizumab in advanced cervical cancer. N Engl J Med. 2014; 370:734–743.
11. Perren TJ, Swart AM, Pfisterer J, Ledermann JA, Pujade-Lauraine E, Kristensen G, et al. A phase 3 trial of bevacizumab in ovarian cancer. N Engl J Med. 2011; 365:2484–2496.
12. Burger RA, Brady MF, Bookman MA, Fleming GF, Monk BJ, Huang H, et al. Incorporation of bevacizumab in the primary treatment of ovarian cancer. N Engl J Med. 2011; 365:2473–2483.
13. Ciombor KK, Berlin J, Chan E. Aflibercept. Clin Cancer Res. 2013; 19:1920–1925.
14. Van Cutsem E, Tabernero J, Lakomy R, Prenen H, Prausová J, Macarulla T, et al. Addition of aflibercept to fluorouracil, leucovorin, and irinotecan improves survival in a phase III randomized trial in patients with metastatic colorectal cancer previously treated with an oxaliplatin-based regimen. J Clin Oncol. 2012; 30:3499–3506.
15. Tirumani SH, Fairchild A, Krajewski KM, Nishino M, Howard SA, Baheti AD, et al. Anti-VEGF molecular targeted therapies in common solid malignancies: comprehensive update for radiologists. Radiographics. 2015; 35:455–474.
16. Llovet JM, Ricci S, Mazzaferro V, Hilgard P, Gane E, Blanc JF, et al. Sorafenib in advanced hepatocellular carcinoma. N Engl J Med. 2008; 359:378–390.
17. Motzer RJ, Hutson TE, Cella D, Reeves J, Hawkins R, Guo J, et al. Pazopanib versus sunitinib in metastatic renal-cell carcinoma. N Engl J Med. 2013; 369:722–731.
18. Goodman VL, Rock EP, Dagher R, Ramchandani RP, Abraham S, Gobburu JV, et al. Approval summary: sunitinib for the treatment of imatinib refractory or intolerant gastrointestinal stromal tumors and advanced renal cell carcinoma. Clin Cancer Res. 2007; 13:1367–1373.
19. Rehman S, Jayson GC. Molecular imaging of antiangiogenic agents. Oncologist. 2005; 10:92–103.
20. Therasse P, Arbuck SG, Eisenhauer EA, Wanders J, Kaplan RS, Rubinstein L, et al. New guidelines to evaluate the response to treatment in solid tumors. European Organization for Research and Treatment of Cancer, National Cancer Institute of the United States, National Cancer Institute of Canada. J Natl Cancer Inst. 2000; 92:205–216.
21. Thiam R, Fournier LS, Trinquart L, Medioni J, Chatellier G, Balvay D, et al. Optimizing the size variation threshold for the CT evaluation of response in metastatic renal cell carcinoma treated with sunitinib. Ann Oncol. 2010; 21:936–941.
22. Kerbel R, Folkman J. Clinical translation of angiogenesis inhibitors. Nat Rev Cancer. 2002; 2:727–739.
23. Nishino M, Jagannathan JP, Krajewski KM, O'Regan K, Hatabu H, Shapiro G, et al. Personalized tumor response assessment in the era of molecular medicine: cancer-specific and therapy-specific response criteria to complement pitfalls of RECIST. AJR Am J Roentgenol. 2012; 198:737–745.
24. Wen PY, Macdonald DR, Reardon DA, Cloughesy TF, Sorensen AG, Galanis E, et al. Updated response assessment criteria for high-grade gliomas: response assessment in neuro-oncology working group. J Clin Oncol. 2010; 28:1963–1972.
25. Chun YS, Vauthey JN, Boonsirikamchai P, Maru DM, Kopetz S, Palavecino M, et al. Association of computed tomography morphologic criteria with pathologic response and survival in patients treated with bevacizumab for colorectal liver metastases. JAMA. 2009; 302:2338–2344.
26. Crabb SJ, Patsios D, Sauerbrei E, Ellis PM, Arnold A, Goss G, et al. Tumor cavitation: impact on objective response evaluation in trials of angiogenesis inhibitors in non-small-cell lung cancer. J Clin Oncol. 2009; 27:404–410.
27. Lencioni R, Llovet JM. Modified RECIST (mRECIST) assessment for hepatocellular carcinoma. Semin Liver Dis. 2010; 30:52–60.
28. Arizumi T, Ueshima K, Takeda H, Osaki Y, Takita M, Inoue T, et al. Comparison of systems for assessment of post-therapeutic response to sorafenib for hepatocellular carcinoma. J Gastroenterol. 2014; 49:1578–1587.
29. van der Veldt AA, Meijerink MR, van den Eertwegh AJ, Haanen JB, Boven E. Choi response criteria for early prediction of clinical outcome in patients with metastatic renal cell cancer treated with sunitinib. Br J Cancer. 2010; 102:803–809.
30. Smith AD, Shah SN, Rini BI, Lieber ML, Remer EM. Morphology, attenuation, size, and structure (MASS) criteria: assessing response and predicting clinical outcome in metastatic renal cell carcinoma on antiangiogenic targeted therapy. AJR Am J Roentgenol. 2010; 194:1470–1478.
31. Krajewski KM, Guo M, Van den Abbeele AD, Yap J, Ramaiya N, Jagannathan J, et al. Comparison of four early posttherapy imaging changes (EPTIC; RECIST 1.0, tumor shrinkage, computed tomography tumor density, Choi criteria) in assessing outcome to vascular endothelial growth factor-targeted therapy in patients with advanced renal cell carcinoma. Eur Urol. 2011; 59:856–862.
32. Badgwell BD, Camp ER, Feig B, Wolff RA, Eng C, Ellis LM, et al. Management of bevacizumab-associated bowel perforation: a case series and review of the literature. Ann Oncol. 2008; 19:577–582.
33. Shinagare AB, Howard SA, Krajewski KM, Zukotynski KA, Jagannathan JP, Ramaiya NH. Pneumatosis intestinalis and bowel perforation associated with molecular targeted therapy: an emerging problem and the role of radiologists in its management. AJR Am J Roentgenol. 2012; 199:1259–1265.
34. Howard SA, Krajewski KM, Thornton E, Jagannathan JP, O'Regan K, Cleary J, et al. Decade of molecular targeted therapy: abdominal manifestations of drug toxicities--what radiologists should know. AJR Am J Roentgenol. 2012; 199:58–64.
35. Hapani S, Sher A, Chu D, Wu S. Increased risk of serious hemorrhage with bevacizumab in cancer patients: a meta-analysis. Oncology. 2010; 79:27–38.
36. Schutz FA, Je Y, Azzi GR, Nguyen PL, Choueiri TK. Bevacizumab increases the risk of arterial ischemia: a large study in cancer patients with a focus on different subgroup outcomes. Ann Oncol. 2011; 22:1404–1412.
37. Choueiri TK, Schutz FA, Je Y, Rosenberg JE, Bellmunt J. Risk of arterial thromboembolic events with sunitinib and sorafenib: a systematic review and meta-analysis of clinical trials. J Clin Oncol. 2010; 28:2280–2285.
38. Nalluri SR, Chu D, Keresztes R, Zhu X, Wu S. Risk of venous thromboembolism with the angiogenesis inhibitor bevacizumab in cancer patients: a meta-analysis. JAMA. 2008; 300:2277–2285.
39. Hurwitz HI, Saltz LB, Van Cutsem E, Cassidy J, Wiedemann J, Sirzén F, et al. Venous thromboembolic events with chemotherapy plus bevacizumab: a pooled analysis of patients in randomized phase II and III studies. J Clin Oncol. 2011; 29:1757–1764.
40. Dienstmann R, Braña I, Rodon J, Tabernero J. Toxicity as a biomarker of efficacy of molecular targeted therapies: focus on EGFR and VEGF inhibiting anticancer drugs. Oncologist. 2011; 16:1729–1740.
41. Singer S, Grommes C, Reiner AS, Rosenblum MK, DeAngelis LM. Posterior reversible encephalopathy syndrome in patients with cancer. Oncologist. 2015; 20:806–811.
42. Tirumani S, Baheti A, Nishino M, Krajewski K, Rosenthal M, Ramaiya N. The cancer imaging radiology report template in the era of molecular-targeted therapy (MTT): what should be included in the radiology interpretation and why. Chicago, IL: Radiological Society of North America 2014 Scientific Assembly and Annual Meeting;Accessed June 29, 2016. Web site. http://archive.rsna.org/2014/14009488.html.
43. Shinagare AB, Jagannathan JP, Krajewski KM, Ramaiya NH. Liver metastases in the era of molecular targeted therapy: new faces of treatment response. AJR Am J Roentgenol. 2013; 201:W15–W28.
44. Herbst RS, Heymach JV, Lippman SM. Lung cancer. N Engl J Med. 2008; 359:1367–1380.
45. Sequist LV, Bell DW, Lynch TJ, Haber DA. Molecular predictors of response to epidermal growth factor receptor antagonists in non-small-cell lung cancer. J Clin Oncol. 2007; 25:587–595.
46. Paez JG, Jänne PA, Lee JC, Tracy S, Greulich H, Gabriel S, et al. EGFR mutations in lung cancer: correlation with clinical response to gefitinib therapy. Science. 2004; 304:1497–1500.
47. Lynch TJ, Bell DW, Sordella R, Gurubhagavatula S, Okimoto RA, Brannigan BW, et al. Activating mutations in the epidermal growth factor receptor underlying responsiveness of non-small-cell lung cancer to gefitinib. N Engl J Med. 2004; 350:2129–2139.
48. Pao W, Miller V, Zakowski M, Doherty J, Politi K, Sarkaria I, et al. EGF receptor gene mutations are common in lung cancers from “never smokers” and are associated with sensitivity of tumors to gefitinib and erlotinib. Proc Natl Acad Sci U S A. 2004; 101:13306–13311.
49. Kwak EL, Bang YJ, Camidge DR, Shaw AT, Solomon B, Maki RG, et al. Anaplastic lymphoma kinase inhibition in non-small-cell lung cancer. N Engl J Med. 2010; 363:1693–1703.
50. Shaw AT, Kim DW, Mehra R, Tan DS, Felip E, Chow LQ, et al. Ceritinib in ALK-rearranged non-small-cell lung cancer. N Engl J Med. 2014; 370:1189–1197.
51. Sequist LV, Martins RG, Spigel D, Grunberg SM, Spira A, Jänne PA, et al. First-line gefitinib in patients with advanced non-small-cell lung cancer harboring somatic EGFR mutations. J Clin Oncol. 2008; 26:2442–2449.
52. Inoue A, Suzuki T, Fukuhara T, Maemondo M, Kimura Y, Morikawa N, et al. Prospective phase II study of gefitinib for chemotherapy-naive patients with advanced non-small-cell lung cancer with epidermal growth factor receptor gene mutations. J Clin Oncol. 2006; 24:3340–3346.
53. Nishino M, Jackman DM, Hatabu H, Jänne PA, Johnson BE, Van den Abbeele AD. Imaging of lung cancer in the era of molecular medicine. Acad Radiol. 2011; 18:424–436.
54. Shaw AT, Kim DW, Nakagawa K, Seto T, Crinó L, Ahn MJ, et al. Crizotinib versus chemotherapy in advanced ALK-positive lung cancer. N Engl J Med. 2013; 368:2385–2394.
55. Nishino M, Dahlberg SE, Fulton LE, Digumarthy SR, Hatabu H, Johnson BE, et al. Volumetric tumor response and progression in EGFR-mutant NSCLC patients treated with erlotinib or gefitinib. Acad Radiol. 2016; 23:329–336.
56. Chaft JE, Oxnard GR, Sima CS, Kris MG, Miller VA, Riely GJ. Disease flare after tyrosine kinase inhibitor discontinuation in patients with EGFR-mutant lung cancer and acquired resistance to erlotinib or gefitinib: implications for clinical trial design. Clin Cancer Res. 2011; 17:6298–6303.
57. Nishino M, Cardarella S, Dahlberg SE, Jackman DM, Ramaiya NH, Hatabu H, et al. Radiographic assessment and therapeutic decisions at RECIST progression in EGFR-mutant NSCLC treated with EGFR tyrosine kinase inhibitors. Lung Cancer. 2013; 79:283–288.
58. Park K, Yu CJ, Kim SW, Lin MC, Sriuranpong V, Tsai CM, et al. First-line erlotinib therapy until and beyond response evaluation criteria in solid tumors progression in asian patients with epidermal growth factor receptor mutation-positive non-small-cell lung cancer: the ASPIRATION study. JAMA Oncol. 2016; 2:305–312.
59. Nishino M, Dahlberg SE, Cardarella S, Jackman DM, Rabin MS, Ramaiya NH, et al. Volumetric tumor growth in advanced non-small cell lung cancer patients with EGFR mutations during EGFR-tyrosine kinase inhibitor therapy: developing criteria to continue therapy beyond RECIST progression. Cancer. 2013; 119:3761–3768.
60. Yang J, Ramalingam SS, Jänne PA, Cantarini M, Mitsudomi T. LBA2_PR: Osimertinib (AZD9291) in pre-treated pts with T790M-positive advanced NSCLC: updated Phase 1 (P1) and pooled Phase 2 (P2) results. J Thorac Oncol. 2016; 11:4 Suppl. S152–S153.
61. Endo M, Johkoh T, Kimura K, Yamamoto N. Imaging of gefitinib-related interstitial lung disease: multi-institutional analysis by the West Japan Thoracic Oncology Group. Lung Cancer. 2006; 52:135–140.
62. Herbst RS, Prager D, Hermann R, Fehrenbacher L, Johnson BE, Sandler A, et al. TRIBUTE: a phase III trial of erlotinib hydrochloride (OSI-774) combined with carboplatin and paclitaxel chemotherapy in advanced non-small-cell lung cancer. J Clin Oncol. 2005; 23:5892–5899.
63. Howard SA, Rosenthal MH, Jagannathan JP, Krajewski KM, Shinagare AB, Ramaiya NH, et al. Beyond the vascular endothelial growth factor axis: update on role of imaging in nonantiangiogenic molecular targeted therapies in oncology. AJR Am J Roentgenol. 2015; 204:919–932.
64. Hughes B, Mileshkin L, Townley P, Gitlitz B, Eaton K, Mitchell P, et al. Pertuzumab and erlotinib in patients with relapsed non-small cell lung cancer: a phase II study using 18F-fluorodeoxyglucose positron emission tomography/computed tomography imaging. Oncologist. 2014; 19:175–176.
65. Miller JA, Ford DJ, Ahmed MS, Loree TR. Two cases of pneumatosis intestinalis during cetuximab therapy for advanced head and neck cancer. Case Rep Oncol Med. 2015; 2015:214236.
66. Lin YT, Wang YF, Yang JC, Yu CJ, Wu SG, Shih JY, et al. Development of renal cysts after crizotinib treatment in advanced ALK-positive non-small-cell lung cancer. J Thorac Oncol. 2014; 9:1720–1725.
67. Weickhardt AJ, Rothman MS, Salian-Mehta S, Kiseljak-Vassiliades K, Oton AB, Doebele RC, et al. Rapid-onset hypogonadism secondary to crizotinib use in men with metastatic nonsmall cell lung cancer. Cancer. 2012; 118:5302–5309.
68. Gibbons JJ, Abraham RT, Yu K. Mammalian target of rapamycin: discovery of rapamycin reveals a signaling pathway important for normal and cancer cell growth. Semin Oncol. 2009; 36:Suppl 3. S3–S17.
69. Shinagare AB, Krajewski KM, Jagannathan JP, Ramaiya NH. Genitourinary imaging: part 2, role of imaging in medical management of advanced renal cell carcinoma. AJR Am J Roentgenol. 2012; 199:W554–W564.
70. Hudes G, Carducci M, Tomczak P, Dutcher J, Figlin R, Kapoor A, et al. Temsirolimus, interferon alfa, or both for advanced renal-cell carcinoma. N Engl J Med. 2007; 356:2271–2281.
71. Motzer RJ, Escudier B, Oudard S, Hutson TE, Porta C, Bracarda S, et al. Efficacy of everolimus in advanced renal cell carcinoma: a double-blind, randomised, placebo-controlled phase III trial. Lancet. 2008; 372:449–456.
72. Ghobrial IM, Gertz M, Laplant B, Camoriano J, Hayman S, Lacy M, et al. Phase II trial of the oral mammalian target of rapamycin inhibitor everolimus in relapsed or refractory Waldenstrom macroglobulinemia. J Clin Oncol. 2010; 28:1408–1414.
73. Oudard S, Thiam R, Fournier LS, Medioni J, Lamuraglia M, Scotte F, et al. Optimisation of the tumour response threshold in patients treated with everolimus for metastatic renal cell carcinoma: analysis of response and progression-free survival in the RECORD-1 study. Eur J Cancer. 2012; 48:1512–1518.
74. Krajewski KM, Nishino M, Franchetti Y, Ramaiya NH, Van den Abbeele AD, Choueiri TK. Intraobserver and interobserver variability in computed tomography size and attenuation measurements in patients with renal cell carcinoma receiving antiangiogenic therapy: implications for alternative response criteria. Cancer. 2014; 120:711–721.
75. Lamuraglia M, Raslan S, Elaidi R, Oudard S, Escudier B, Slimane K, et al. mTOR-inhibitor treatment of metastatic renal cell carcinoma: contribution of Choi and modified Choi criteria assessed in 2D or 3D to evaluate tumor response. Eur Radiol. 2016; 26:278–285.
76. Maroto JP, Hudes G, Dutcher JP, Logan TF, White CS, Krygowski M, et al. Drug-related pneumonitis in patients with advanced renal cell carcinoma treated with temsirolimus. J Clin Oncol. 2011; 29:1750–1756.
77. Dabydeen DA, Jagannathan JP, Ramaiya N, Krajewski K, Schutz FA, Cho DC, et al. Pneumonitis associated with mTOR inhibitors therapy in patients with metastatic renal cell carcinoma: incidence, radiographic findings and correlation with clinical outcome. Eur J Cancer. 2012; 48:1519–1524.
78. Atkinson BJ, Cauley DH, Ng C, Millikan RE, Xiao L, Corn P, et al. mTOR inhibitor associated noninfectious pneumonitis in patients with renal cell cancer: management, predictors, and outcomes. BJU Int. 2014; 113:376–382.
79. Nishino M, Boswell EN, Hatabu H, Ghobrial IM, Ramaiya NH. Drug-related pneumonitis during mammalian target of rapamycin inhibitor therapy: radiographic pattern-based approach in Waldenström macroglobulinemia as a paradigm. Oncologist. 2015; 20:1077–1083.
80. Nishino M, Brais LK, Brooks NV, Hatabu H, Kulke MH, Ramaiya NH. Drug-related pneumonitis during mammalian target of rapamycin inhibitor therapy in patients with neuroendocrine tumors: a radiographic pattern-based approach. Eur J Cancer. 2016; 53:163–170.
81. Tirumani SH, Krajewski KM, Shinagare AB, Jagannathan JP, Ramaiya NH. Gallbladder complications associated with molecular targeted therapies: clinical and imaging features. Clin Imaging. 2014; 38:50–55.
82. Tirumani SH, Jagannathan JP, Shinagare AB, Kim KW, Krajewski KM, Ramaiya NH. Acute pancreatitis associated with molecular targeted therapies: a retrospective review of the clinico-radiological features, management and outcome. Pancreatology. 2013; 13:461–467.
83. Parithivel K, Ramaiya N, Jagannathan JP, O'Regan K, Krajewski K, Fisher D, et al. Everolimus- and temsirolimus-associated enteritis: report of three cases. J Clin Oncol. 2011; 29:e404–e406.
84. National Clinical Practice Guidelines in Oncology (NCCN guidelines): Breast cancer-version 3. 2015. Accessed November 29, 2016. Web site. http://www.consensocancermamario.com/guias/NCCN_2015.pdf.
85. Finn RS, Crown JP, Lang I, Boer K, Bondarenko IM, Kulyk SO, et al. The cyclin-dependent kinase 4/6 inhibitor palbociclib in combination with letrozole versus letrozole alone as first-line treatment of oestrogen receptor-positive, HER2-negative, advanced breast cancer (PALOMA-1/TRIO-18): a randomised phase 2 study. Lancet Oncol. 2015; 16:25–35.
86. Cristofanilli M, Turner NC, Bondarenko I, Ro J, Im SA, Masuda N, et al. Fulvestrant plus palbociclib versus fulvestrant plus placebo for treatment of hormone-receptor-positive, HER2-negative metastatic breast cancer that progressed on previous endocrine therapy (PALOMA-3): final analysis of the multicentre, double-blind, phase 3 randomised controlled trial. Lancet Oncol. 2016; 17:425–439.
87. Baselga J, Campone M, Piccart M, Burris HA 3rd, Rugo HS, Sahmoud T, et al. Everolimus in postmenopausal hormone-receptor-positive advanced breast cancer. N Engl J Med. 2012; 366:520–529.
88. Cooley C, Nishino M, Jagannathan J, Ramaiya N, Di Salvo D, Krajewski KM. Clinical problem solving: utility of sonography in oncologic patients. J Ultrasound Med. 2014; 33:9–22.
89. Hadji P, Aapro MS, Body JJ, Bundred NJ, Brufsky A, Coleman RE, et al. Management of aromatase inhibitor-associated bone loss in postmenopausal women with breast cancer: practical guidance for prevention and treatment. Ann Oncol. 2011; 22:2546–2555.
90. Pegram MD, Lipton A, Hayes DF, Weber BL, Baselga JM, Tripathy D, et al. Phase II study of receptor-enhanced chemosensitivity using recombinant humanized anti-p185HER2/neu monoclonal antibody plus cisplatin in patients with HER2/neu-overexpressing metastatic breast cancer refractory to chemotherapy treatment. J Clin Oncol. 1998; 16:2659–2671.
91. Baselga J, Cortés J, Kim SB, Im SA, Hegg R, Im YH, et al. Pertuzumab plus trastuzumab plus docetaxel for metastatic breast cancer. N Engl J Med. 2012; 366:109–119.
92. Geyer CE, Forster J, Lindquist D, Chan S, Romieu CG, Pienkowski T, et al. Lapatinib plus capecitabine for HER2-positive advanced breast cancer. N Engl J Med. 2006; 355:2733–2743.
93. Lin NU, Diéras V, Paul D, Lossignol D, Christodoulou C, Stemmler HJ, et al. Multicenter phase II study of lapatinib in patients with brain metastases from HER2-positive breast cancer. Clin Cancer Res. 2009; 15:1452–1459.
94. Krop IE, LoRusso P, Miller KD, Modi S, Yardley D, Rodriguez G, et al. A phase II study of trastuzumab emtansine in patients with human epidermal growth factor receptor 2-positive metastatic breast cancer who were previously treated with trastuzumab, lapatinib, an anthracycline, a taxane, and capecitabine. J Clin Oncol. 2012; 30:3234–3241.