Abstract
Objective
Materials and Methods
Results
Figures and Tables
Fig. 1
Schematic picture of 2 orthogonal inversion bands localized geographically for displaying renal artery.
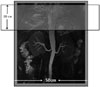
Fig. 2
Diagrams of relative signal change and respiratory triggered fat-sat 3D FIESTA sequence with SLEEK preparation.
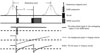
Fig. 3
Example of 26-year-old volunteer, who underwent SLEEK with various BSP TIs with 300 ms interval from 200 ms to 2600 ms.
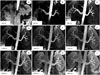
Fig. 4
Assessment of renal artery image quality in SLEEK MR angiography with various BSP TIs (800 ms, 1100 ms, 1400 ms) according to different median BRs and HRs in 3 hypertensive patients.
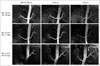
Fig. 5
Case 40-year-old hypertensive woman suspected of renal artery stenosis with optimal BSP TI of 800 ms at BR of 17-18/min and HR of 77-80 bpm.
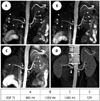
Fig. 6
Case of 28-year-old woman with hypertension for > 10 years and renal artery stenosis due to fibromuscular dysplasia with optimal BSP TI = 1100 ms at BR of 14-15/min and HR of 74-76 bpm.
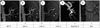
Fig. 7
Case of 54-year-old woman with hypertension for 8 years suspected of RAS with optimal BSP TI = 1100 ms at BR of 13-14/min and HR of 68-70 bmp.
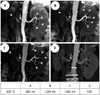
Table 1
Image Quality of Renal Arteries by SLEEK NCE-MRA with Different BR and HR for Each Volunteer
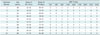
"3" indicated best image (most SNR, most delineation of main renal artery and segmental branches in renal parenchyma, least interference from venous system); "2" represented better images (more SNR, more delineation of main renal artery and segmental branches in renal parenchyma, less interference from venous system); "1" implied good images (good SNR, delineation of main renal artery, some artifact may be present from venous system); "0" hinted poor images (low inhomogeneous signal intensity, incomplete delineation of main renal artery border, more artifact from venous system). bpm = beats per minute, BR = breathing rate, BSP TI = blood suppression inversion time, HR = heart rate, NCE-MRA = non-contrast enhanced magnetic resonance angiography, SLEEK = spatial labeling with multiple inversion pulses, SNR = signal-to-noise ratio
Table 2
Image Quality of SLEEK for All Hypertension Patients by Two Readers
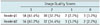
Image Quality Scores | ||||
---|---|---|---|---|
3 | 2 | 1 | 0 | |
Reader#1 | 58 (62.4%) | 30 (32.2%) | 2 (2.2%) | 3 (3.2%) |
Reader#2 | 56 (60.2%) | 32 (34.4%) | 3 (3.2%) | 2 (2.2%) |
Table 3
Assessment of Renal Arteries with SLEEK by Two Readers in Comparison with Results of CTA and DSA
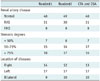
For those patients with FMD and bilateral renal artery disease, maximum stenotic degree was determined and recorded as stage. CTA = computed tomography angiography, DSA = digital subtraction angiography, FMD = fibromuscular dysplasia, RAS = renal artery stenosis, SLEEK = spatial labeling with multiple inversion pulses
Table 4
95% Confidence Intervals of BRs and HRs for Choosing Optimal BSP TI with Two Readers Respectively*
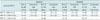