Abstract
Objective
To compare the apparent diffusion coefficient (ADC) value using single-shot echo-planar imaging sequences at 3T and 1.5T for differentiation of benign fracture edema and tumor infiltration of the vertebral body.
Materials and Methods
A total of 46 spinal examinations were included in the 1.5T MRI group, and a total of 40 spinal examinations were included in the 3T MRI group. The ADC values of the lesion were measured and calculated. The diagnostic performance of the conventional MR image containing sagittal T2-weighted fat saturated image and each diffusion weighted image (DWI) with an ADC value with different b values were evaluated.
Results
The mean ADC value of the benign lesions was higher than that of the malignant lesions on 1.5T and 3T (p < 0.05). The sensitivity of the diagnostic performance was higher with an additional DWI in both 1.5T and 3T, but the sensitivities were similar with the addition of b values of 400 and 1000. The specificities of the diagnostic performances did not show significant differences (p value > 0.05). The diagnostic accuracies were higher when either of the DWIs (b values of 400 and 1000) was added to routine MR image for 1.5T and 3T. Statistical differences between 1.5T and 3T or between b values of 400 and 1000 were not seen.
Conclusion
The ADC values of the benign lesions were significantly higher than those of the malignant lesions on 1.5T and 3T. There was no statistically significant difference in the diagnostic performances when either of the DWIs (b values of 400 and 1000) was added to the routine MR image for 1.5T and 3T.
Benign marrow edema combined with compression fracture is as common as vertebral tumor infiltration due to multiple causes such as metastasis, multiple myeloma, and lymphoma. Differentiation between benign and malignant marrow pathology is difficult because the change of the signal intensity can be similar for fractures with both malignant and benign causes, although fat containing bone marrow of the spine is displaced by tumor cell in the malignant lesion (12). Diffusion weighted image (DWI) has been proposed as a solution to this problem. DWI is suitable for probing the structure of biologic tissue at a microscopic level and can be used in the differential diagnosis between benign and malignant marrow pathology (345). 3T MR imaging has the possibility of an almost doubled signal-to-noise ratio (SNR) than 1.5T MR and the possibility of decreased scan times without reduction in quality. Increased SNR can be used to improve contrast or spatial resolution, but the increased effects of the magnetic susceptibility differences of tissues scanned at higher fields can result in signal loss in regions of greater signal de-phasing such as tissue-bone interfaces and tissue-air interfaces (6). Diffusion imaging at 3T MR provides potentially useful information about bone abnormalities in the evaluation of the long bone tumor (7). To the best of our knowledge, a detailed and organized study concerning DWI of the vertebral body in the evaluation of benign marrow edema and malignant tumor infiltration at 3T MR with comparison to 1.5T MR has not been performed. In this study, we determined whether DWI of 3T MR is superior to 1.5T MR in the differential diagnosis between benign and malignant marrow lesion of the spine. The purpose of this study was to compare apparent diffusion coefficient (ADC) value using single-shot echo-planar imaging sequences (SSH-EPI) at 3T and 1.5T for differentiation of benign fracture edema and tumor infiltration of the vertebral body and assess whether the diagnostic performance of routine MR sequences is improved with the addition of DWI.
Our Institutional Ethics Review board approved this retrospective study. We retrospectively evaluated consecutive 86 patients with back pain due to trauma or metastatic lesion who underwent diffusion MR through 1.5T or 3T between August 2011 and July 2012. Our study sample included 26 men and 20 women aged 31–85 years (mean 65 ± 12 years) in the 1.5T MRI group and 15 men and 25 women aged 23–87 years (mean 62 ± 16 years) in the 3T MRI group. A total of 46 spine examinations were included in the 1.5T MRI group (23 thoracic, 21 lumbar, and 2 sacral spine examinations), and a total of 40 spine examinations were included in the 3T MRI group (12 thoracic, 23 lumbar, and 5 sacral spine examinations). In the 1.5T MRI group, 23 cases of benign marrow edema combined with a compression fracture and 23 cases of malignant marrow infiltration due to metastasis were included (8 lung cancers, 5 nasopharyngeal carcinomas, 4 hepatocellular carcinomas, 4 renal cell carcinomas, and 2 cholangiocarcinomas). In the 3T MRI group, 19 cases of benign marrow edema combined with a compression fracture and 21 cases of malignant marrow infiltration due to metastasis were included (7 lung cancers, 4 nasopharyngeal carcinomas, 4 breast carcinomas, 3 colon carcinomas, 2 prostatic carcinomas, and 1 cervical carcinoma). Among malignant cases, 2 cases (8.6%) were osteoblastic metastasis in the 1.5T MRI group and 2 cases (9.5%) were osteoblastic metastasis in the 3T MRI group. Other malignant cases were osteolytic metastasis, which were confirmed through plain radiography and CT. Two fellowship-trained musculoskeletal radiologists with 12 and 9 years of experience made the diagnoses in consensus. Patients were considered to have benign marrow edema when they had no evidence of underlying malignancy and no aggravation of the lesion on the follow-up MR for more than 1 year, instead showing fatty change. Seventeen (40%) of total 42 cases of the benign lesion underwent whole body bone scan to exclude the possibility of the malignant lesion such as metastasis. Patients were diagnosed with malignant lesions when they had an underlying malignancy that showed aggravating clinical symptoms without inflammatory symptoms and an aggravating lesion (increased edema size with a paraspinal mass or a newly developed lesion) on the follow-up MR at 6 months or 1 year (3). Vertebral body metastases were confirmed pathologically through surgical or bone biopsy in 5 cases. All primary malignant lesions of the metastatic lesions were also proven pathologically. Patients who underwent vertebroplasty or surgical procedure of spine (2 cases) and patients with spondylitis (pyogenic or tuberculous, 5 cases) were excluded from the study. The spondylitis was defined when the patient showed elevated white blood cell or C-reactive protein count without trauma history and epidural abscess formation with combined discitis. The diagnoses were made by two musculoskeletal radiologists by consensus.
MR examinations were performed using the same protocol on a 1.5T and 3T MRI scanner (Intera and Achieva, Philips, Best, the Netherlands) using each 8-channel phased-array spine coil. The gradient strengths of each MR were 66 mT/m and 80 mT/m with a slew rate of 80 mT/m/ms and 200 mT/m/ms, respectively. The sequences and imaging parameters of the standard sagittal spin echo were summarized in Table 1. Diffusion images were acquired using a multi-slice fat saturation spin-echo type single shot echo-planar imaging sequence in the sagittal plane. The scan covered from lower cervical spine to upper lumbar spine in T spine MR and from lower thoracic spine to upper sacral spine in L spine with field of view of 30 cm. Sagittal DWIs were acquired in the same plane and orientation as used in the routine sequences with an SSH-EPI sequence (repetition time/echo time 1700 ms/70 ms; field of view 28 × 20 cm; section thickness 4 mm; intersection gap 0 mm; sections 12; matrix 150 × 120) with spectral pre-saturation and fat saturation. A diffusion-weighting gradient was applied along the section selection, readout, and phase encoding directions with a fixed gradient amplitude (fixed b value) to minimize the effects of diffusion anisotropy. The ADC value was calculated from the slope of the semi-log plot of the signal intensity as a function of the b factor from two diffusion-weighted images, according to the following equation: ADC = log (SI1 / SI2) / (b2 - b1), where SI1 and SI2 are the SIs of DWIs obtained with two b values. The b values used in our study were 0, 400, and 1000 (sec/mm2) based on the report of Oztekin et al. (1) that DWI with b value 300 (low b value) provides excellent distinction between metastatic tumor and benign edema; in addition, we thought that the b value of 400 is close to 300. The b value 1000 (high b value) is commonly used in neuroradiology for evaluation of stroke (8).
The signals of the DWI and ADC values were obtained from a region of interest (ROI) placed within the lesion (Figs. 1, 2). The size of the ROI was matched to the size of the lesion. The average diameter of the ROI was 7.5 mm (minimum: 4 mm, maximum: 10.4 mm). Two fellowship-trained musculoskeletal radiologists with 12 and 9 years of experience performed each measurement twice and the average of the two values was used. The diagnostic performances of the routine MR image containing a sagittal T2-weighted fat saturated image and T1-weighted sagittal image and each DWI with an ADC value with a different b value were evaluated. The MR images were interpreted independently by two musculoskeletal radiologists who were unaware of radiologic diagnosis and clinical history. First, the readers evaluated routine MR images of all patients only on 1.5T and 3T MRI. During sessions spaced a week apart, they evaluated routine MR images with additional information of the DWI with b value 400 on 1.5T and 3T MRI, and evaluated routine MR images with additional information of the DWI with b value 1000 in another session at an interval of a week. The readers diagnosed the lesion as benign or malignant based on routine image and DWI, as well as tables of the ADC values that had been acquired during our study. The benign group and the malignant group were interpreted in the randomly selected order. The radiological morphologic criteria to diagnose the lesions as malignant on conventional MR included the presence of retro-pulsion of a posterior bone fragment, no preserved normal marrow signal on T1 and T2-weighted MR, involvement of the posterior element, and presence of paraspinal mass (3).
We used chi-square test to evaluate whether the statistical bias exists according to the age and sex. The intra-class correlation coefficient (ICC) was used in the analysis of interobserver agreement between the two radiologists. ICC values < 0.40 indicate poor reproducibility, ICC values of 0.40–0.75 indicate fair-to-good reproducibility, and ICC values > 0.75 indicate excellent reproducibility (9). The ADC values were calculated to the arithmetic mean and compared using the Mann-Whitney U test and Friedman's two-way analysis. Interobserver agreement of diagnostic performance was analyzed using kappa statistics. The kappa value interpretation was as follows: poor (k ≤ 0), slight (0 < k ≤ 0.2), fair (0.2 < k ≤ 0.4), moderate (0.4 < k ≤ 0.6), substantial (0.6 < k ≤ 0.8), and nearly perfect (0.8 < k ≤ 1) (10). The diagnostic performances of the various imaging methods were evaluated using the sensitivity, specificity, and accuracy for differentiating between benign and malignant pathology, as determined by the use of routine sequences with additional DWIs. The pathologic or clinical findings were used as reference standards. The values were analyzed using the McNemar test. Statistical analyses were performed using PASW software version 18.0 (IBM, Armonk, NY, USA). P values ≤ 0.05 were considered statistically significant.
The distributions of the age and sex were not significantly different across categories of the 1.5T and 3T (p value = 0.088, 0.832). The mean ADC value of the benign lesions was higher than that of the malignant lesions on 1.5T and 3T (p value < 0.05) (Table 2, Fig. 3). The mean ADC value with a b value of 0–400 was the highest and that with a b value of 0–400–1000 was the lowest on 1.5T and 3T. Although significant differences of ADC values were noted between benign and malignant lesions of both 1.5T and 3T MR (p value < 0.05), significant differences of ADC values were not noted between 1.5T and 3T MR (p value = 0.105–0.897), except for the mean ADC value of benign lesions between 1.5T and 3T with a b value of 0–400 (p value = 0.006/0.031, reader 1/reader 2) (Table 2).
The ICCs of the ADC values showed excellent reproducibility, except for benign lesions with a b value of 0–400 and malignant lesions with a b value of 0–400–1000 on 3T, which showed fair-to-good reproducibility. Interobserver agreement of the diagnostic performance between readers was substantial or nearly perfect (0.689–0.957). The number of false positive and false negative diagnoses by each reader ranged from 1 to 7 for benign and malignant lesions on 1.5T and 3T. There were 7 cases of false negative (i.e., that is diagnosed as benign but proved to be malignant) and 4 cases of false positive (diagnosed as malignant but proved to be benign) on 1.5T; and 7 cases of false negative and 3 cases of false positive on 3T by reader 1 and 5 cases of false negative and one case of false positive on both 1.5T and 3T by reader 2. The number of cases of misdiagnosis with the addition of the DWI was from 0 to 4. There were one false negative and one false positive case on 1.5T with b value 400 and one false negative case and 2 false positive cases on 3T with b value 1000 by reader 1. In addition, there were 2 false negative and one false positive case in 1.5T and 4 cases of false negative and one case of false positive on 3T with b values 400 and one false negative and false positive case with b value 1000 on both 1.5T and 3T. The sensitivity of the diagnostic performance of reader 1 was higher with an additional DWI in both 1.5T and 3T, but the sensitivities were similar with the addition of b values of 400 and 1000 (Table 3). The specificities of the diagnostic performances did not show significant differences (p value = 0.340–0.994). The diagnostic accuracies (of reader 1 but not of reader 2) were higher when either of the DWIs (b values of 400 and 1000) was added to routine MR image for 1.5T. Statistical differences between 1.5T and 3T or between b values of 400 and 1000 were not seen (p value = 0.097–1.000) (Table 4). Cut off values of ADC for diagnosing malignant compression fracture in 1.5T and 3T MR were described in Table 5.
Although MRI is sensitive in the assessment of metastasis and benign bone marrow pathology, the specificity is low because of similar signal intensity changes (3). Ancillary findings such as complete replacement of the vertebral body marrow, a paravertebral soft tissue mass, involvement of the posterior elements, and an associated paraspinal mass are suggestive of malignant pathology (3). However, these signs are not detected in all patients, leading to difficulty in assessing the cause of the lesion (5). 3T MR provides an almost doubled SNR, as compared with 1.5T MR thus facilitating decrease in scan times without reduction in quality (6). DWI involves changes in motion of water molecules and is considered a powerful tool for the differential diagnosis of benign edema and tumorous involvement of bone marrow (11). Water in vital tumor cells shows lower mobility as a result of cellular structures (12). In the presence of diffusion sensitizing gradients, it results in a lower signal attenuation compared with the stronger de-phasing of higher mobility extracellular water with extensive signal attenuation. Therefore, extensive signal attenuation (38–52%) can be seen in benign edema, whereas tumor infiltration produces no significant signal reduction (13). Whether DWI offers an advantage over conventional MR for the differentiation of benign and malignant pathology in the spine, remains controversial (514).
The ADC values of the benign lesions were higher than those for malignant lesions with a b value of 0–400, 0–1000 and 0–400–1000 on both 1.5T and 3T (Table 2). Nevertheless, some overlap between the ranges of the ADC values for benign and malignant lesions was observed. Tang et al. (11) discussed the large overlap between the range of ADC values for benign and malignant compression fractures and suggested b values within a range around 300 as optimal to minimize overlap between benign and malignant lesions. However, Balliu et al. (15) reported that mean ADC value from benign edema (1.9 ± 0.39 × 10-3 mm2/sec) is significantly higher than malignant lesion (0.9 ± 1.3 × 10-3 mm2/sec) in the study of 1.5T MR with b value 0 and 500. We obtained similar results (2.5 ± 0.8 vs. 1.6 ± 0.6 × 10-3 mm2/sec) under the similar condition (ADC derived from b value 0 and 400). Recently, Geith et al. (16) reported opposing results in DWI on 46 patients who underwent 1.5T MR, with no significant differences of the ADC derived from b value 50, 250, and 500 between benign (1.2 ± 0.37 × 10-3 mm2/sec) and malignant lesion (1.1 ± 0.26 × 10-3 mm2/sec) of the spine. Dietrich et al. (17) reported that benign compression fractures exhibit generally between 1.0 and 2.0 × 10-3 mm2/sec and marrow infiltrating lesions of the vertebral body demonstrate generally between 0.7 and 1.0 × 10-3 mm2/sec. In our study, the difference of ADC between benign and malignant lesion showed consistent statistical significance, irrespective of magnetic strength and b value (Table 2), indicating that benign lesion shows higher ADC value than malignant lesion. The ADC values of each lesion were higher on 1.5T than on 3T, with statistical significance only in benign lesions with a b value of 0–400. We are unable to describe the exact etiology of this phenomenon. Nevertheless, ADC value is certainly affected by b value, as well as magnetic field strength (18). We investigated whether the addition of DWI (b value of 400 or 1000) to the routine MR image influenced the sensitivity, specificity, or accuracy of the diagnostic differentiation between benign and malignant lesions. Our results showed that additional DWIs tended to increase the sensitivity, specificity, and accuracy; however, significance was reached only for accuracies of reader 1 with 1.5T (Tables 3, 4).
We found poor ICCs for the ADC values at 3T, possibly due to severe image distortions with higher strength magnets. Although 3T MR provides increased SNR compared with lower strength magnets, this increase is at the expense of greater susceptibility to various artifacts due to higher B0 (19). Furthermore, when a larger b value is adopted, the quality of the DWI is seriously degraded and the SNR decreases (20). The quality of the DWI decreases greatly when the b value is above 400; consequently, calculation of the ADC value is unreliable due to interference of the apparent background noise (10).
There are a few limitations to this study. First, most of the diagnoses were based on clinical and radiologic evidence such as underlying malignancy, recent trauma history, or changes on the follow-up MRI. Pathologic confirmation of the marrow pathology was only performed in 5 cases. The second limitation was the small size of the study population. Thirdly, patients with other benign pathology such as spondylitis (pyogenic or tuberculous) were not included in our study.
In conclusion, the ADC values of the benign lesions were significantly higher than those of the malignant lesions on 1.5T and 3T. There was no statistically significant difference in the diagnostic performances when either of the DWIs (b values of 400 and 1000) was added to the routine MR image for 1.5T and 3T.
Figures and Tables
![]() | Fig. 1Diffusion weighted image with b value of 0 in 60-year-old woman with lung cancer metastasis.Regions of interest were placed within lesion of vertebral body.
|
![]() | Fig. 258 years old man with oropharyngeal carcinoma metastasis.
A. 3T MRI T2-weighted sagittal image (TR/TE, 2500/120) shows metastatic lesion in S1 (arrow). B. Diffusion weighted image with b value of 0. Regions of interest were placed within lesion (lower circle) and normal marrow (upper circle) of vertebral body. C. Diffusion weighted image with b value of 400. D. Diffusion weighted image with b value of 1000. E. ADC map with b value of 0–400. Region of interest were placed within lesion. F. ADC map with b value of 0–1000. G. ADC map with b value of 0–400–1000. First, both readers diagnosed lesion as malignant based on routine MR images. During second session, both readers diagnosed lesion as malignant based on routine MR images with additional information of DWI with b value 400. During third session, readers (both reader 1 and reader 2) diagnosed lesion as malignant based on routine MR images with additional information of DWI with b value 1000. ADC = apparent diffusion coefficient, DWI = diffusion weighted image, TE = echo time, TR = repetition time
|
![]() | Fig. 3Graph shows mean apparent diffusion coefficient (ADC) values (× 10-6 mm2/sec).
A. Benign lesion. B. Malignant lesion. R1 = reader 1, R2 = reader 2
|
Table 1
Imaging Parameters for 1.5T and 3T Conventional Sagittal MR Sequences
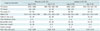
Table 2
Mean Apparent Diffusion Coefficient Values (× 10-6 mm2/sec) and P Values
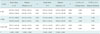
Table 3
Sensitivity, Specificity, and Accuracy of Diagnostic Performance Using Routine MR Image Only (A), Addition of DWI with b Value of 400 (B), and Addition of DWI with b Value of 1000 (C)
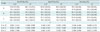
Table 4
P-Values of Diagnostic Performances between 1.5T and 3T Using Routine MR Image Only (A), Addition of DWI with b Value of 400 (B), and Addition of DWI with b Value of 1000 (C)

Table 5
Cut Off Values (× 10-6 mm2/sec) of Apparent Diffusion Coefficient (ADC) for Diagnosing Malignant Compression Fracture in 1.5T and 3T MR

References
1. Oztekin O, Ozan E, Hilal Adibelli Z, Unal G, Abali Y. SSH-EPI diffusion-weighted MR imaging of the spine with low b values: is it useful in differentiating malignant metastatic tumor infiltration from benign fracture edema? Skeletal Radiol. 2009; 38:651–658.
2. Kim YP, Kannengiesser S, Paek MY, Kim S, Chung TS, Yoo YH, et al. Differentiation between focal malignant marrow-replacing lesions and benign red marrow deposition of the spine with T2*-corrected fat-signal fraction map using a three-echo volume interpolated breath-hold gradient echo Dixon sequence. Korean J Radiol. 2014; 15:781–791.
3. Herneth AM, Philipp MO, Naude J, Funovics M, Beichel RR, Bammer R, et al. Vertebral metastases: assessment with apparent diffusion coefficient. Radiology. 2002; 225:889–894.
4. Baur A, Stäbler A, Brüning R, Bartl R, Krödel A, Reiser M, et al. Diffusion-weighted MR imaging of bone marrow: differentiation of benign versus pathologic compression fractures. Radiology. 1998; 207:349–356.
5. Castillo M. Diffusion-weighted imaging of the spine: is it reliable? AJNR Am J Neuroradiol. 2003; 24:1251–1253.
6. Phalke VV, Gujar S, Quint DJ. Comparison of 3.0 T versus 1.5 T MR: imaging of the spine. Neuroimaging Clin N Am. 2006; 16:241–248. ix
7. Ahlawat S, Khandheria P, Subhawong TK, Fayad LM. Differentiation of benign and malignant skeletal lesions with quantitative diffusion weighted MRI at 3T. Eur J Radiol. 2015; 84:1091–1097.
8. Kuhl CK, Textor J, Gieseke J, von Falkenhausen M, Gernert S, Urbach H, et al. Acute and subacute ischemic stroke at high-field-strength (3.0-T) diffusion-weighted MR imaging: intraindividual comparative study. Radiology. 2005; 234:509–516.
9. Rosner B. Fundamentals of biostatistics. 6th ed. Belmont, CA: Duxbury Press;2005.
10. Viera AJ, Garrett JM. Understanding interobserver agreement: the kappa statistic. Fam Med. 2005; 37:360–363.
11. Tang G, Liu Y, Li W, Yao J, Li B, Li P. Optimization of b value in diffusion-weighted MRI for the differential diagnosis of benign and malignant vertebral fractures. Skeletal Radiol. 2007; 36:1035–1041.
12. Lang P, Wendland MF, Saeed M, Gindele A, Rosenau W, Mathur A, et al. Osteogenic sarcoma: noninvasive in vivo assessment of tumor necrosis with diffusion-weighted MR imaging. Radiology. 1998; 206:227–235.
13. Spuentrup E, Buecker A, Adam G, van Vaals JJ, Guenther RW. Diffusion-weighted MR imaging for differentiation of benign fracture edema and tumor infiltration of the vertebral body. AJR Am J Roentgenol. 2001; 176:351–358.
14. Castillo M, Arbelaez A, Smith JK, Fisher LL. Diffusion-weighted MR imaging offers no advantage over routine noncontrast MR imaging in the detection of vertebral metastases. AJNR Am J Neuroradiol. 2000; 21:948–953.
15. Balliu E, Vilanova JC, Peláez I, Puig J, Remollo S, Barceló C, et al. Diagnostic value of apparent diffusion coefficients to differentiate benign from malignant vertebral bone marrow lesions. Eur J Radiol. 2009; 69:560–566.
16. Geith T, Schmidt G, Biffar A, Dietrich O, Dürr HR, Reiser M, et al. Comparison of qualitative and quantitative evaluation of diffusion-weighted MRI and chemical-shift imaging in the differentiation of benign and malignant vertebral body fractures. AJR Am J Roentgenol. 2012; 199:1083–1092.
17. Dietrich O, Biffar A, Reiser MF, Baur-Melnyk A. Diffusion-weighted imaging of bone marrow. Semin Musculoskelet Radiol. 2009; 13:134–144.
18. Lee SY, Jee WH, Jung JY, Park MY, Kim SK, Jung CK, et al. Differentiation of malignant from benign soft tissue tumours: use of additive qualitative and quantitative diffusion-weighted MR imaging to standard MR imaging at 3.0 T. Eur Radiol. 2016; 26:743–754.
19. Subhawong TK, Jacobs MA, Fayad LM. Diffusion-weighted MR imaging for characterizing musculoskeletal lesions. Radiographics. 2014; 34:1163–1177.
20. Yuan YH, Xiao EH, He Z, Xiang J, Tang KL, Yan RH, et al. MR diffusion-weighed imaging of rabbit liver. World J Gastroenterol. 2005; 11:5506–5511.