Abstract
The presence of myocardial ischemia is the most important prognostic factor in patients with ischemic heart disease. Fractional flow reserve (FFR) is a gold standard invasive method used to detect the stenosis-specific myocardial ischemia. FFR-guided revascularization strategy is superior to angiography-guided strategy. The recently developed hyperemia-free index, instantaneous wave free ratio is being actively investigated. A non-invasive FFR derived from coronary CT angiography is now used in clinical practice. Due to rapid expansion of invasive and non-invasive physiologic assessment, comprehensive understanding of the role and potential pitfalls of each modality are required for its application. In this review, we focus on the basic and clinical aspects of physiologic assessment in ischemic heart disease.
Myocardial ischemia can occur when myocardial perfusion cannot meet the demands of the myocardium and is a key prognostic factor in patients with coronary artery disease (12). Numerous efforts are made to detect the presence of myocardial ischemia. Despite many available non-invasive tests, it is reported that about 60% of patients referred 320for invasive coronary angiography on suspicion of coronary artery disease from positive non-invasive tests do not have obstructive disease (3).
Current advances in the concept of physiologic assessment, and several newly developed invasive and non-invasive indices are being applied in clinical practice. Fractional flow reserve (FFR) is an invasive physiologic index that can be easily measured in the cardiac catheterization laboratory. In this review, we discuss the clinical aspects of coronary physiology through the concept, physiological background and clinical data of FFR. In addition, we further discuss resting physiologic indices, non-invasive FFR and comprehensive physiologic assessment in patients with ischemic heart disease.
Fractional flow reserve is defined as the ratio of maximal coronary blood flow in a diseased artery to maximal coronary blood flow in the same artery without stenosis (456). Flow is proportional to pressure in the case of minimal and constant resistance, thus pressure can be a surrogate of flow during maximal hyperemia (Fig. 1). As distal coronary artery pressure (Pd) in a normal coronary artery is almost the same as the aortic pressure (Pa), normal perfusion pressure can be replaced by Pa. As venous pressures are generally negligible compared to arterial pressure, FFR can be simply calculated as the ratio of Pd to Pa during maximal hyperemia (456). The definition and an example of FFR measurement are shown in Figure 2. FFR of 0.80 indicates that the diseased coronary artery supplies 80% of the normal maximal flow due to the stenosis. The strength of FFR is that it can assess the degree and presence of epicardial lesion-specific inducible myocardial ischemia, not only in cases with negative or ambiguous results of non-invasive functional tests, but also in the presence of multivessel disease (Figs. 3, 4). FFR also has excellent reproducibility, regardless of changes in hemodynamics or myocardial contractility (789).
As previously mentioned, a linear relationship between coronary flow and pressure is the fundamental assumption in the concept of FFR. As coronary blood flow and resistance are auto-regulated based on the myocardial demand, within the physiological range (10), induction of maximal hyperemia or minimizing microvascular resistance is mandatory for FFR measurement (56). Continuous intravenous infusion of adenosine (140 µg/kg/min) is considered as a gold standard method to orgachieve maximal hyperemia for FFR measurement (11). Intravenous infusion of adenosine can cause chest discomfort, atrioventricular conduction delay and bronchial hyper-reactivity, although their incidences and clinical significance are low. Intracoronary bolus administration of adenosine is a simple method for hyperemia induction, and injection of 50–200 µg of adenosine is considered adequate for FFR measurement (12). However, due to its short action time, steady state hyperemia for pressure pullback tracing cannot be maintained with a single bolus administration of adenosine. Besides adenosine, nicorandil and regadenoson are recently introduced as novel hyperemic agents. Nicorandil (Sigmart®, Chugai Pharmaceutical, Tokyo, Japan) is a nicotinamide ester with dual mechanisms of action on both macro- and microvascular systems (1314). Jang et al. (13) report similar hyperemic efficacy between intracoronary nicorandil injection (2 mg) and intravenous infusion of adenosine. Compared with adenosine, nicorandil causes less frequent adverse effects (pressure change, heart rate change, chest discomfort). The excellent diagnostic efficacy and safety of intracoronary bolus administration of nicorandil are confirmed at the patient-level pooled data from 429 patients with 480 coronary arteries (r = 0.941, intra-class correlation coefficient 0.980, classification agreement 90.8%, kappa = 0.814, area under curve of nicorandil 0.980, all p < 0.001) (15). Regadenoson is a direct A2A adenosine receptor agonist that can be administered as a single bolus intravenous injection. It has rapid onset but longer duration of action and fewer adverse effects, as compared with adenosine (1617). Lim et al. (18) compared intravenous infusion of adenosine, intracoronary bolus injection of adenosine, intracoronary bolus injection of nicorandil and regadenoson and reported that FFR values were not significantly different among the different hyperemic agents. The study results on the currently available hyperemic agents are summarized in Table 1.
The optimal cut-off value of FFR for defining inducible myocardial ischemia is extensively investigated using non-invasive stress tests. Pioneer work of Pijls et al. (6) proposed a cut-off value of 0.75, based on the comparison of invasive FFR and the results of sequential tests of exercise-stressed tests, thallium scintigraphy, and dobutamine-stress echocardiography (sensitivity 88%, specificity 100%, positive predictive value 100%, negative predictive value 88%, and accuracy 93%). Using the ischemic cut-off value of FFR, the first randomized study of FFR-guided percutaneous coronary intervention (PCI), the DEFER trial, tested the safety of deferral of functionally insignificant stenosis (19). The DEFER trial randomly assigned patients with functionally insignificant intermediate lesions (FFR ≥ 0.75) into the Perform group (n = 90) and the Defer group (n = 91). Patients with FFR < 0.75 were allocated into the Reference group and underwent PCI (n = 144). The 2- and 5-year follow-up data show that both the Defer and Perform groups have no difference in the incidence of mortality, myocardial infarction (MI), or revascularization (1920). Recently published, 15 year follow-up data further support the concept that the deferral of functionally insignificant lesions is safe, and stent implantation for these lesions cannot reduce the incidence of clinical events (21). In recent clinical practice, FFR binary cut-off value of 0.80 is in use, in order to minimize the chance of leaving an untreated functionally significant stenosis.
The Fractional Flow Reserve versus Angiography for Multivessel Evaluation (FAME) trial enrolled 1005 patients with multivessel disease and randomly assigned them to angiography-guided PCI group (n = 496) and FFR-guided PCI group (n = 509). In the FFR-guided group, stents were placed only for stenoses with FFR ≤ 0.80. The primary end-point was major adverse cardiac event (MACE, a composite of death, MI and any revascularization) at 1-year. At 1-year, the FFR-guided PCI group showed significantly lower rates of MACE (13.2% vs. 18.3%, p = 0.02) and combined death and MI (7.3% vs. 11%, p = 0.04), compared with the angiography-guided PCI group. Furthermore, the FFR-guided PCI group significantly enhanced the process-of-care index, including fewer stents per patient (1.9 ± 1.3 vs. 2.7 ± 1.2, p < 0.001), less contrast (272 mL vs. 302 mL, p < 0.001), lower procedural cost, and shorter hospital stay (22). FAME 2-year data shows similar benefit of FFR-guided PCI (23). Recently published 5-year results show that the MACE rate was similar between FFR-guided and angiography-guided groups (28% vs. 31%, relative risk 0.91, 95% confidential interval [CI] 0.75–1.10, p = 0.31) with significantly less number of stents at index procedure in the FFR-guided group (24).
The Fractional Flow Reserve versus Angiography for Multivessel Evaluation 2 (FAME 2) trial compared FFR-guided PCI plus optimal medical therapy with optimal medical therapy alone in patients with functionally significant stenosis (FFR ≤ 0.80) (2526). In this study, patients with a functionally significant lesion (FFR ≤ 0.80) were randomly assigned to FFR-guided PCI plus optimal medical therapy group and only optimal medical therapy group, whereas patients with FFR > 0.80 in all stenoses received optimal medical therapy and were assigned as a registry group. The study was halted prematurely because of the significant difference of composite of death, MI or urgent revascularization (4.3% for FFR-guided PCI group vs. 12.7% for optimal medical therapy group, p < 0.001) (2526). Accordingly, European guidelines recommend FFR-guided revascularization with Class I (level of evidence A) in stable patients when evidence of ischemia is not available (27).
Recently, 5-year results of the Proper Fractional Flow Reserve Criteria for Intermediate Lesions in the Era of Drug-Eluting Stent (DEFER-DES) trial demonstrated the clinical relevance of the FFR-guided strategy in the drug-eluting stent era.
Routine DES group underwent DES implantation without FFR measurement. At 5-year follow-up, routine DES implantation could not reduce the incidence of MACE compared to FFR-guided DES implantation group. Comparison of the 3 groups shows that 5-year MACE rate was higher in the FFR-DES (low FFR and DES implantation) group (24%) than the Routine-DES group (14%, p = 0.193) and FFR-Defer (high FFR and medical treatment) group (7%, p = 0.012) (28). The clinical benefit of FFR-guided strategy over angiography-only guided strategy is also well established by the large scale registry data (293031). Park et al. (30) report clinical outcomes before (2008–2009) and after (2010–2011) the adoption of the routine use of FFR from the single-center ASAN PCI registry. Comparison of primary endpoint (a composite of any death, MI, or any revascularization at 1-year) was performed in propensity-score matched population (2178 pairs). The risk of primary endpoint was significantly lower in patients treated by FFR-guided strategy, compared with those who were managed before the adoption of routine FFR-guided strategy (4.8% vs. 8.6%, hazard ratio [HR] 0.55, 95% CI 0.43–0.70, p < 0.001). The significant reduction of the risk of primary endpoint was mainly due to a reduction in MI and revascularization. The number of stents per patient was also significantly decreased with the adoption of FFR-guided strategy. In addition, Li et al. (29) compared long-term 7-year clinical outcomes between FFR-guided strategy and angiography-guided strategy from 7358 consecutive patients in the Mayo Clinic registry (2002–2009). The Kaplan-Meier fraction of MACE at 7 years was 57.0% in the angiography-guided group vs. 50.0% in the FFR-guided group (p = 0.016). In addition to those clinical data, cost-effectiveness of FFR-guided strategy over angiography-guided strategy is also demonstrated (3233). These investigations collectively support the safety and effectiveness of an FFR-guided strategy, which reduces unnecessary stent implantation and enhances patient's clinical outcome.
In addition to the robust data supporting the FFR-guided decision making process, recent investigations increase our understanding of coronary physiology and FFR. In meta-analysis at the study-level (n = 9173) as well as individual patient-level (n = 6961), Johnson et al. (34) provide new insight on the prognostic importance of FFR value in terms of a continuous variable. Study-level meta-regression analysis showed the significant inverse relationship between FFR values and normalized 1-year rate of MACE, and this inverse relationship was also repeated with Cox regression analysis of patient-level pooled data. When the regression lines according to treatment modality (revascularization vs. medical treatment) were plotted, 2 regression lines crossed at the point FFR value 0.75 in the study-level analysis and 0.67 in the patients-level analysis. Thus, patients with low-normal range of FFR value (0.81–0.85) had higher risk of future events than those with higher or near normal FFR values. In addition, FFR measured after PCI also had an inverse relationship with prognosis (HR 0.86, 95% CI 0.80–0.93, p < 0.001).
The concept of instantaneous wave free ratio (iFR) was originally derived from wave-intensity analysis using both intracoronary pressure and flow velocity data. Davies et al. (35) report a certain period in the cardiac cycle when the resistance is low and stable (36). iFR is calculated by Pd/Pa ratio at the wave-free period during resting state and does not require hyperemia (Fig. 5). The ADenosine Vasodilator Independent Stenosis Evaluation (ADVISE) is the first study to evaluate the concept of iFR (37). In this study, iFR was closely correlated with FFR (r = 0.9, p < 0.001) and showed excellent diagnostic performance (C-statistics 0.93) to predict low FFR. In the ADVISE study, the optimal cut-off value of iFR to predict FFR < 0.80 was 0.83 with sensitivity, specificity, positive predictive value, and negative predictive value of 85%, 91%, 91%, and 85%, respectively (37). Subsequent to the introduction of iFR, its diagnostic accuracy and validity during resting period have been under debate (383940). In this regard, the large scale RESOLVE study was designed to investigated the data of 1768 patients from 15 international centers. In this study, iFR was measured using a uniform calculation algorithm in the independent physiologic core laboratory (41). As a result, the optimal cut-off value of iFR was 0.90 for FFR ≤ 0.80 and C-statistics was 0.81 (95% CI: 0.79 to 0.84). The optimal cut-off value of resting Pd/Pa was 0.92 for FFR ≤ 0.80 and C-statistics was 0.82 (95% CI: 0.80 to 0.84). There was no significant difference in diagnostic performance between resting Pd/Pa and iFR (41). Despite its convenience, more evidence is needed to support the routine use of resting index, such as iFR, in daily clinical practice. The currently ongoing randomized controlled trials, which compare the clinical outcomes between iFR- and FFR-guided strategy, will clarify the clinical relevance of an iFR-guided strategy (DEFINE-FLAIR NCT02053038, SWEDEHEART NCT02166736) (42).
Coronary CT angiography (cCTA) provides accurate anatomical information. However, the discrepancy between anatomical severity and functional significance is well-known (84344454647484950). With the advancement of computational fluid dynamics (CFD) technology, CT-derived FFR (FFRCT) has been developed to provide a non-invasive estimate of FFR (Fig. 6). The FFRCT technology possesses a robust scientific basis that is well described in previous reviews (515253). Briefly, a three-dimensional patient specific anatomic model of coronary artery is first constructed from the cCTA data. For assigning boundary conditions of CFD simulation, the basal coronary outlet resistances at resting state are determined from the principle of an allometric scaling law, which allows the estimation of total coronary flow from myocardial mass; and a morphometry law, which relates the resistance of the downstream vessel to the vessel size at each outlet. A mathematical model of hyperemic condition is derived from the effect of adenosine on reducing the resistance of the coronary microcirculation. Lastly, on the basis of discretized model of patient-specific geometry and boundary conditions, CFD analysis is performed to numerically solve the governing equations of fluid dynamics, i.e., Navier Stokes equations, as a Newtonian fluid. The numerical solutions of coronary flow and pressure fields are used to compute a complete spatial distribution of FFRCT.
Table 2 summarizes the previous studies that evaluate the clinical relevance of FFRCT technology. Three prospective trials (DISCOVER-FLOW, DeFACTO, NXT) validate the efficacy of FFRCT technology and establish a role of FFRCT as a novel gate-keeper for patients with suspected coronary artery disease (545556). The obvious benefit in cost-effectiveness of a FFRCT-guided clinical decision making process is also presented using the previous trial populations, as compared with traditional clinical decision making process (5758).
Furthermore, the recently published Prospective LongitudinAl Trial of FFRCT: Outcome an Resource Impacts (PLATFORM) trial evaluates clinical outcomes of FFRCT-guided diagnostic strategy, compared with usual care of patients with suspected coronary artery disease in real-world practice (59). Among those with intended invasive coronary angiography (FFRCT-guided = 193; usual care = 187), no obstructive coronary artery disease was found at the time of invasive angiography in 24 (12%) in the cCTA/FFRCT arm and 137 (73%) in the usual care arm (risk difference 61%, 95% CI 53–69, p < 0.0001), with similar mean cumulative radiation exposure (9.9 mSv vs. 9.4 mSv, p = 0.20). In addition, invasive coronary angiography was ruled out in 61% after FFRCT (59). These results suggest the potential of FFRCT as a non-invasive diagnostic modality in the clinical decision-making process.
The investigators in DISCOVER-FLOW evaluate the potential of FFRCT technology in planning the treatment strategy using the so-called "Virtual PCI technology" (60). Modification of the computational model to restore the area of the target lesion according to the proximal and distal reference areas (i.e., virtual stenting), allows for estimation of post-interventional FFRCT values (60). Kim et al. (60) evaluated this novel strategy in 44 patients who had functionally significant coronary stenoses with available pre-intervention coronary cCTA and pre- and post-intervention FFR values. Both pre- and post-interventional values of invasive FFR and FFRCT showed an excellent correlation. The mean difference between FFRCT and FFR was 0.006 for pre-intervention (95% limit of agreement: -0.27 to 0.28) and 0.024 for post-intervention (95% limit of agreement: -0.08 to 0.13). Diagnostic accuracy of FFRCT to predict ischemia (FFR ≤ 0.8) prior to stenting was 77% (sensitivity: 85.3%, specificity: 57.1%, PPV: 83%, and NPV: 62%) and after stenting was 96% (sensitivity: 100%, specificity: 96%, PPV: 50%, and NPV: 100%). The value of FFRCT as a "treatment planner" is still under development and needs further investigation.
In addition to FFRCT, several investigators are working with new methodologies for non-invasive estimation of FFR using cCTA or angiograms (616263). Furthermore, the clinical relevance of comprehensive hemodynamic assessment using cCTA and CFD is under active investigation (64). However, any non-invasive FFR from cCTA requires adequate anatomic geometries and physiologic boundary conditions for CFD analysis. Adherence to established best image acquisition practices, including heart rate control and use of pre-scan nitroglycerin, is essential to improve cCTA image quality. Further refinement of this technology is expected to improve its diagnostic accuracy and contribute to better patient care in clinical practice.
Although FFR is now regarded as the gold-standard invasive method to assess the functional significance of coronary artery stenosis (65), there is still room for further improvement in the diagnosis and treatment of patients with high FFR. In the FAME 2 study, 14.6% of the registry arm (FFR > 0.80 and deferral of PCI) experienced persistent angina, and 9.0% of these patients had clinical events during a 2-year follow-up period (66). This observation suggests that the ischemic heart disease cannot be fully explained by epicardial stenosis alone. The coronary artery system has 3 components with different functions (conductive epicardial coronary arteries, arterioles, and capillaries), hence, failure of any one of these systems could result in myocardial ischemia. Thus, the presence of epicardial coronary artery stenosis is not the sole factor for ischemic heart disease (67).
In this regard, previous studies have suggested that the measurement of coronary flow reserve (CFR) could be helpful in risk stratification for patients with high FFR (> 0.80). Previous studies report that low CFR has worse clinical outcome than normal CFR in the setting of normal FFR patients, implying that dysfunction or disease in microvascular circulatory beds are also contributors to ischemic heart disease, especially in the case of functionally insignificant epicardial stenosis (686970). An index of microcirculatory resistance (IMR) is currently introduced, since CFR is largely influenced by variations in the resting coronary flow and not a microcirculatory bed-specific index. IMR is a pressure-temperature derived parameter for quantifying microcirculatory resistance (71). As distal coronary pressure is used in the calculation of IMR, this index can be used to interrogate selectively the microcirculation of vessels with a coronary stenosis, in contrast to CFR, which is a combined assessment of the macro- and microcirculation. Table 3 summarizes previous evidence regarding IMR (72737475767778798081). According to the evidence of CFR and IMR, high FFR, low CFR and high IMR suggest the presence of microvascular disease in the coronary circulatory bed. Figure 7 shows an example of a patient with microcirculatory disease.
Therefore, comprehensive evaluation using multiple physiologic indices should be regarded as a diagnostic approach to enhance the stratification of patients, according to major compartment(s) involved in the development of ischemic heart disease.
This review focuses on the invasive physiologic assessment of ischemic heart disease, and presents evidence for its clinical relevance and effectiveness in the enhancement of patient's clinical outcomes. Despite the low prevalence of invasive physiologic assessment in daily practice, recently developed novel indices and hyperemic agents are expected to reduce the current barriers. Furthermore, comprehensive assessment of both macro- and microvascular systems and practical application of cCTA-derived non-invasive FFR will further improve clinical outcomes of patients with ischemic heart disease.
Figures and Tables
Fig. 1
Relationship between pressure and flow during resting and hyperemic states.
In resting state, coronary artery is under autoregulation to maintain adequate coronary flow according to oxygen demand. Pressure and flow are in linear correlation when microvascular resistance is minimal and constant.
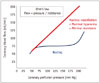
Fig. 2
Concept of fractional flow reserve.
Fractional flow reserve (FFR) is defined as ratio of maximal coronary blood flow in diseased artery (
) to normal maximal coronary blood flow in same artery (
). As venous pressure (Pv) is negligible compared to aortic (Pa) and distal coronary pressure (Pd), FFR can be calculated as ratio of Pd and Pa.


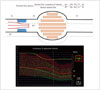
Fig. 3
Case with discrepancy between exercise stress test and fractional flow reserve (FFR).
76-year-old man with stable angina who underwent coronary CT angiography (A) and exercise stress test (B). There was intermediate stenosis in proximal left anterior descending coronary artery (LAD) and exercise stress test was negative. Invasive angiography revealed similar stenosis in LAD (C) and FFR measured in LAD was 0.70, which suggests presence of myocardial ischemia (D). Red arrows in (A) and (C) show same intermediate lesion in proximal LAD.
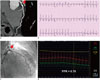
Fig. 4
Case with discrepancy between myocardial perfusion imaging and fractional flow reserve.
A. 72-year-old woman with stable angina who underwent myocardial SPECT. Reversible perfusion defect was absent. B. Invasive coronary angiography revealed severe 3-vessel disease (arrows). C. FFR was 0.69 in left anterior descending coronary artery and 0.73 in left circumflex artery, respectively. FFR = fractional flow reserve, LAD = left anterior descending coronary artery, LCX = left circumflex artery, SPECT = single photon emission computed tomography
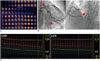
Fig. 5
Wave intensity analysis and concept of instantaneous wave-free ratio (iFR).
Upper panel shows example of wave intensity analysis. Different types of waves originating from proximal and distal (from microcirculatory beds) sites during entire cardiac cycle are presented. After beginning of diastole and before start of systole, there is wave free period in which microvascular resistance is minimized and constant. iFR is calculated by ratio of proximal and distal pressures during this period (lower panel).
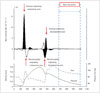
Fig. 6
Non-invasive hemodynamic assessment using coronary CT angiography and computational fluid dynamics.
With recent advancement of computational fluid dynamics, non-invasive hemodynamic assessment has become feasible. Adding physiological modeling to coronary CT angiography-derived 3-dimensional coronary artery geometry enables assessment of several hemodynamic parameters, such as wall shear stress (A), pressure gradient (B), and fractional flow reserve (FFRCT) (C).
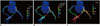
Fig. 7
Case example of microvascular disease.
69-year-old female patient presented with stable angina. Despite positive exercise stress test (ST segment depression in inferior and lateral leads) (A), there was no significant coronary artery stenosis (B). Invasive physiologic assessment was performed and fractional flow reserve, coronary flow reserve (CFR) and index of microcirculatory resistance (IMR) were 0.94, 1.4, and 39, respectively (C). As there was no significant epicardial disease (high fractional flow reserve), low CFR and high IMR indicate presence of microvascular disease.
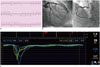
Table 1
Summary of Clinical Studies on Hyperemic Agents
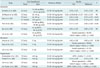
Study | Test (Vasodilator) | Reference Method | Results | P | ||
---|---|---|---|---|---|---|
Route | Dose | Test | Reference | |||
Adenosine | ||||||
Jeremias et al. (82) | IC bolus | 15–20 ug (RCA), 18–24 ug (LCA) | IV AD 140 ug/kg/min | 0.78 ± 0.15 | 0.78 ± 0.15 | NS |
De Bruyne et al. (83) | IC bolus | 20, 40 ug | IV AD 140 ug/kg/min | 0.62 ± 0.20/0.60 ± 0.19 | 0.61 ± 0.19 | NS |
Koo et al. (84) | IC bolus | 40, 80 ug | IV AD 140 ug/kg/min | 0.83 ± 0.06 | 0.79 ± 0.07 | < 0.01 |
IC infusion | 240 ug/min | IV AD 140 ug/kg/min | 0.78 ± 0.09 | 0.79 ± 0.07 | 0.40 | |
Yoon et al. (85) | IC bolus | 36–60 ug (RCA), 48–80 ug (LCA) | IV AD 140 ug/kg/min | 0.77 ± 0.10 | 0.80 ± 0.08 | < 0.05 |
Seo et al. (86) | IC bolus | 40 ug (RCA), 80 ug (LCA) | IV AD 140 ug/kg/min | 0.81 ± 0.10 | 0.80 ± 0.10 | NS |
Lim et al. (18) | IC bolus | 40 ug (RCA), 80 ug (LCA) | IV AD 140 ug/kg/min |
Overall agreement = 92.9%, Cohen's kappa = 0.887, p < 0.001 |
||
Nicorandil | ||||||
Jang et al. (13) | IC bolus | 2 mg | IV AD 140 ug/kg/min | 0.82 ± 0.09 | 0.82 ± 0.10 | 0.180 |
Kang et al. (15) | IC bolus | 2 mg | IV AD 140 ug/kg/min |
Overall agreement = 90.8%, Cohen's kappa = 0.814, p < 0.001 |
||
Lim et al. (18) | IC bolus | 2 mg | IV AD 140 ug/kg/min |
Overall agreement = 91.2%, Cohen's kappa = 0.817, p < 0.001 |
||
Regadenoson | ||||||
Arumugham et al. (87) | IV bolus | 400 ug | IV AD 140 ug/kg/min | ΔFFR = 0.0040, r2 = 0.933 | ||
Prasad et al. (88) | IV bolus | 400 ug | IV AD 140 ug/kg/min | 0.79 ± 0.09 | 0.79 ± 0.09 | NS |
Lim et al. (18) | IV bolus | 400 ug | IV AD 140 ug/kg/min |
Overall agreement = 100%, Cohen's kappa = 1.000, p < 0.001 |
||
van Nunen et al. (89) | IV bolus | 400 ug | IV AD 140 ug/kg/min | ΔFFR = 0.00 ± 0.01, r = 0.994, p < 0.001 |
Table 2
Summary of Clinical Studies on cCTA-Derived FFR
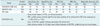
Study | Number of Lesions | Sensitivity (%) | Specificity (%) | PPV (%) | NPV (%) | Diagnostic Accuracy (%) | |||||
---|---|---|---|---|---|---|---|---|---|---|---|
FFRCT | cCTA | FFRCT | cCTA | FFRCT | cCTA | FFRCT | cCTA | FFRCT | cCTA | ||
DISCOVER-FLOW (54) | 103 | 93 | 94 | 82 | 25 | 85 | 58 | 91 | 80 | 84 | 61 |
DeFACTO (55) | 252 | 90 | 84 | 54 | 42 | 67 | 61 | 84 | 72 | 73 | 64 |
NXT (56) | 254 | 86 | 94 | 79 | 34 | 65 | 40 | 93 | 92 | 81 | 53 |
PLATFORM (59) | 584 | Study about impact of FFRCT on clinical practice | |||||||||
FFRCT guided group showed significantly lower portion of no obstructive CAD than usual group in ICA (12% vs. 73%) | |||||||||||
Also, cCTA/FFRCT guided strategy decreased ICA about 61% | |||||||||||
Early adverse events for 90 days were similar between cCTA/FFRCT guided group and usual group |
Table 3
Clinical Evidences on IMR
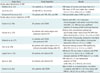
Study (Year) | Study Population | Results |
---|---|---|
Studies about distribution of IMR | ||
Melikian et al. (72) | 101 patients vs. 15 controls | IMR values of controls were lower than 25 U |
Luo et al. (74) | 18 with CXS vs. 18 controls | IMR values of CXS were higher than controls (33.1 ± 7.9 vs. 18.8 ± 5.6, p < 0.001) |
Echavarría-Pinto et al. (73) | 79 patients with FFR, CFR, and IMR | 75th percentile value of IMR was 29 U |
Studies about clinical implication of IMR | ||
Fearon et al. (76) | 29 patients with STEMI | Patients with IMR > 32 U had worse echocardiographic wall motion score than those with IMR ≤ 32. IMR was only significant predictor of recovery of left ventricular function |
Cuisset et al. (75) | 50 patients with stable angina who underwent elective PCI | Patients with conventional stenting had significantly higher value of post-PCI IMR than direct stenting (24 ± 14 U vs. 13 ± 3 U, p < 0.01) |
McGeoch et al. (77) | 57 patients with STEMI who underwent CMR | Patients with microvascular obstruction had higher IMR values than those without microvascular obstruction (38 U vs. 27 U, p = 0.003) |
Fujii et al. (78) | 80 patients with stable angina with or without pravastatin therapy after PCI | Pravastatin therapy lowered IMR significantly after PCI (12.6 U vs. 17.6 U, p = 0.007) |
Layland et al. (79) | 50 patients with elective PCI | IMR before PCI was higher in patients with PPMI (21.2 ± 2.1 vs. 15.6 ± 1.8, p = 0.02) and strongest predictor of PPMI (beta 0.7, p = 0.02) |
Ng et al. (80) | 50 patients with elective PCI | IMR value of > 27 was independent predictor of PPMI (odds ratio, 22.7; 95% CI, 3.8–133.9) |
Fearon et al. (81) | 254 patients with STEMI | Rate of death or re-hospitalization was higher in patients with IMR of > 40 U than those with IMR of ≤ 40 (17.1% vs. 6.6%, p = 0.027) |
CFR = coronary flow reserve, CMR = cardiac magnetic resonance imaging, CXS = cardiac X syndrome, FFR = fractional flow reserve, IMR = index of microcirculatory resistance, MACE = major adverse cardiovascular event, PCI = percutaneous coronary intervention, PPMI = peri-procedural myocardial infarction, STEMI = ST-elevation myocardial infarction
References
1. Shaw LJ, Berman DS, Maron DJ, Mancini GB, Hayes SW, Hartigan PM, et al. Optimal medical therapy with or without percutaneous coronary intervention to reduce ischemic burden: results from the Clinical Outcomes Utilizing Revascularization and Aggressive Drug Evaluation (COURAGE) trial nuclear substudy. Circulation. 2008; 117:1283–1291.
2. Shaw LJ, Iskandrian AE. Prognostic value of gated myocardial perfusion SPECT. J Nucl Cardiol. 2004; 11:171–185.
3. Patel MR, Peterson ED, Dai D, Brennan JM, Redberg RF, Anderson HV, et al. Low diagnostic yield of elective coronary angiography. N Engl J Med. 2010; 362:886–895.
4. Young DF, Cholvin NR, Kirkeeide RL, Roth AC. Hemodynamics of arterial stenoses at elevated flow rates. Circ Res. 1977; 41:99–107.
5. Pijls NH, van Son JA, Kirkeeide RL, De Bruyne B, Gould KL. Experimental basis of determining maximum coronary, myocardial, and collateral blood flow by pressure measurements for assessing functional stenosis severity before and after percutaneous transluminal coronary angioplasty. Circulation. 1993; 87:1354–1367.
6. Pijls NH, De Bruyne B, Peels K, Van Der Voort PH, Bonnier HJ, Bartunek J, et al. Measurement of fractional flow reserve to assess the functional severity of coronary-artery stenoses. N Engl J Med. 1996; 334:1703–1708.
7. Koo BK. The present and future of fractional flow reserve. Circ J. 2014; 78:1048–1054.
8. Kern MJ, Samady H. Current concepts of integrated coronary physiology in the catheterization laboratory. J Am Coll Cardiol. 2010; 55:173–185.
9. de Bruyne B, Bartunek J, Sys SU, Pijls NH, Heyndrickx GR, Wijns W. Simultaneous coronary pressure and flow velocity measurements in humans. Feasibility, reproducibility, and hemodynamic dependence of coronary flow velocity reserve, hyperemic flow versus pressure slope index, and fractional flow reserve. Circulation. 1996; 94:1842–1849.
10. Mosher P, Ross J Jr, Mcfate PA, Shaw RF. Control of coronary blood flow by an autoregulatory mechanism. Circ Res. 1964; 14:250–259.
11. Pijls NH, van Nunen LX. Fractional flow reserve, maximum hyperemia, adenosine, and regadenoson. Cardiovasc Revasc Med. 2015; 16:263–265.
12. Adjedj J, Toth GG, Johnson NP, Pellicano M, Ferrara A, Floré V, et al. Intracoronary adenosine: dose-response relationship with hyperemia. JACC Cardiovasc Interv. 2015; 8:1422–1430.
13. Jang HJ, Koo BK, Lee HS, Park JB, Kim JH, Seo MK, et al. Safety and efficacy of a novel hyperaemic agent, intracoronary nicorandil, for invasive physiological assessments in the cardiac catheterization laboratory. Eur Heart J. 2013; 34:2055–2062.
14. Kato D, Takashima H, Waseda K, Kurita A, Kuroda Y, Kosaka T, et al. Feasibility and safety of intracoronary nicorandil infusion as a novel hyperemic agent for fractional flow reserve measurements. Heart Vessels. 2015; 30:477–483.
15. Kang DY, Lee JM, Kato D, Oi M, Toyofuku M, Takashima H, et al. TCT-297 Safety and efficacy of intracoronary nicorandil as hyperemic agent for invasive physiological assessment: patient-level pooled analysis of current evidence. J Am Coll Cardiol. 2015; 66(15_S):DOI: 10.1016/j.jacc.2015.08.312.
16. Iskandrian AE, Bateman TM, Belardinelli L, Blackburn B, Cerqueira MD, Hendel RC, et al. Adenosine versus regadenoson comparative evaluation in myocardial perfusion imaging: results of the ADVANCE phase 3 multicenter international trial. J Nucl Cardiol. 2007; 14:645–658.
17. Al Jaroudi W, Iskandrian AE. Regadenoson: a new myocardial stress agent. J Am Coll Cardiol. 2009; 54:1123–1130.
18. Lim WH, Koo BK, Nam CW, Doh JH, Park JJ, Yang HM, et al. Variability of fractional flow reserve according to the methods of hyperemia induction. Catheter Cardiovasc Interv. 2015; 85:970–976.
19. Bech GJ, De Bruyne B, Pijls NH, de Muinck ED, Hoorntje JC, Escaned J, et al. Fractional flow reserve to determine the appropriateness of angioplasty in moderate coronary stenosis: a randomized trial. Circulation. 2001; 103:2928–2934.
20. Pijls NH, van Schaardenburgh P, Manoharan G, Boersma E, Bech JW, van't Veer M, et al. Percutaneous coronary intervention of functionally nonsignificant stenosis: 5-year follow-up of the DEFER Study. J Am Coll Cardiol. 2007; 49:2105–2111.
21. Zimmermann FM, Ferrara A, Johnson NP, van Nunen LX, Escaned J, Albertsson P, et al. Deferral vs. performance of percutaneous coronary intervention of functionally non-significant coronary stenosis: 15-year follow-up of the DEFER trial. Eur Heart J. 2015; 36:3182–3188.
22. Tonino PA, De Bruyne B, Pijls NH, Siebert U, Ikeno F, van't Veer M, et al. Fractional flow reserve versus angiography for guiding percutaneous coronary intervention. N Engl J Med. 2009; 360:213–224.
23. Pijls NH, Fearon WF, Tonino PA, Siebert U, Ikeno F, Bornschein B, et al. Fractional flow reserve versus angiography for guiding percutaneous coronary intervention in patients with multivessel coronary artery disease: 2-year follow-up of the FAME (Fractional Flow Reserve Versus Angiography for Multivessel Evaluation) study. J Am Coll Cardiol. 2010; 56:177–184.
24. van Nunen LX, Zimmermann FM, Tonino PA, Barbato E, Baumbach A, Engstrøm T, et al. Fractional flow reserve versus angiography for guidance of PCI in patients with multivessel coronary artery disease (FAME): 5-year follow-up of a randomised controlled trial. Lancet. 2015; 386:1853–1860.
25. De Bruyne B, Pijls NH, Kalesan B, Barbato E, Tonino PA, Piroth Z, et al. Fractional flow reserve-guided PCI versus medical therapy in stable coronary disease. N Engl J Med. 2012; 367:991–1001.
26. De Bruyne B, Fearon WF, Pijls NH, Barbato E, Tonino P, Piroth Z, et al. Fractional flow reserve-guided PCI for stable coronary artery disease. N Engl J Med. 2014; 371:1208–1217.
27. Kolh P, Windecker S, Alfonso F, Collet JP, Cremer J, Falk V, et al. 2014 ESC/EACTS Guidelines on myocardial revascularization: the Task Force on Myocardial Revascularization of the European Society of Cardiology (ESC) and the European Association for Cardio-Thoracic Surgery (EACTS). Developed with the special contribution of the European Association of Percutaneous Cardiovascular Interventions (EAPCI). Eur J Cardiothorac Surg. 2014; 46:517–592.
28. Park SH, Jeon KH, Lee JM, Nam CW, Doh JH, Lee BK, et al. Long-term clinical outcomes of fractional flow reserve-guided versus routine drug-eluting stent implantation in patients with intermediate coronary stenosis: five-year clinical outcomes of DEFER-DES trial. Circ Cardiovasc Interv. 2015; 8:pii: e002442.
29. Li J, Elrashidi MY, Flammer AJ, Lennon RJ, Bell MR, Holmes DR, et al. Long-term outcomes of fractional flow reserve-guided vs. angiography-guided percutaneous coronary intervention in contemporary practice. Eur Heart J. 2013; 34:1375–1383.
30. Park SJ, Ahn JM, Park GM, Cho YR, Lee JY, Kim WJ, et al. Trends in the outcomes of percutaneous coronary intervention with the routine incorporation of fractional flow reserve in real practice. Eur Heart J. 2013; 34:3353–3361.
31. Fröhlich GM, Redwood S, Rakhit R, MacCarthy PA, Lim P, Crake T, et al. Long-term survival in patients undergoing percutaneous interventions with or without intracoronary pressure wire guidance or intracoronary ultrasonographic imaging: a large cohort study. JAMA Intern Med. 2014; 174:1360–1366.
32. Fearon WF, Yeung AC, Lee DP, Yock PG, Heidenreich PA. Cost-effectiveness of measuring fractional flow reserve to guide coronary interventions. Am Heart J. 2003; 145:882–887.
33. Siebert U, Arvandi M, Gothe RM, Bornschein B, Eccleston D, Walters DL, et al. Improving the quality of percutaneous revascularisation in patients with multivessel disease in Australia: cost-effectiveness, public health implications, and budget impact of FFR-guided PCI. Heart Lung Circ. 2014; 23:527–533.
34. Johnson NP, Tóth GG, Lai D, Zhu H, Açar G, Agostoni P, et al. Prognostic value of fractional flow reserve: linking physiologic severity to clinical outcomes. J Am Coll Cardiol. 2014; 64:1641–1654.
35. Davies JE, Whinnett ZI, Francis DP, Manisty CH, Aguado-Sierra J, Willson K, et al. Evidence of a dominant backward-propagating “suction” wave responsible for diastolic coronary filling in humans, attenuated in left ventricular hypertrophy. Circulation. 2006; 113:1768–1778.
36. Siebes M, Kolyva C, Verhoeff BJ, Piek JJ, Spaan JA. Potential and limitations of wave intensity analysis in coronary arteries. Med Biol Eng Comput. 2009; 47:233–239.
37. Sen S, Escaned J, Malik IS, Mikhail GW, Foale RA, Mila R, et al. Development and validation of a new adenosine-independent index of stenosis severity from coronary wave-intensity analysis: results of the ADVISE (ADenosine Vasodilator Independent Stenosis Evaluation) study. J Am Coll Cardiol. 2012; 59:1392–1402.
38. Berry C, van't Veer M, Witt N, Kala P, Bocek O, Pyxaras SA, et al. VERIFY (VERification of Instantaneous Wave-Free Ratio and Fractional Flow Reserve for the Assessment of Coronary Artery Stenosis Severity in EverydaY Practice): a multicenter study in consecutive patients. J Am Coll Cardiol. 2013; 61:1421–1427.
39. Park JJ, Petraco R, Nam CW, Doh JH, Davies J, Escaned J, et al. Clinical validation of the resting pressure parameters in the assessment of functionally significant coronary stenosis; results of an independent, blinded comparison with fractional flow reserve. Int J Cardiol. 2013; 168:4070–4075.
40. Sen S, Asrress KN, Nijjer S, Petraco R, Malik IS, Foale RA, et al. Diagnostic classification of the instantaneous wave-free ratio is equivalent to fractional flow reserve and is not improved with adenosine administration. Results of CLARIFY (Classification Accuracy of Pressure-Only Ratios Against Indices Using Flow Study). J Am Coll Cardiol. 2013; 61:1409–1420.
41. Jeremias A, Maehara A, Généreux P, Asrress KN, Berry C, De Bruyne B, et al. Multicenter core laboratory comparison of the instantaneous wave-free ratio and resting Pd/Pa with fractional flow reserve: the RESOLVE study. J Am Coll Cardiol. 2014; 63:1253–1261.
42. Götberg M, Christiansen EH, Gudmundsdottir I, Sandhall L, Omerovic E, James SK, et al. Instantaneous Wave-Free Ratio versus Fractional Flow Reserve guided intervention (iFR-SWEDEHEART): rationale and design of a multicenter, prospective, registry-based randomized clinical trial. Am Heart J. 2015; 170:945–950.
43. Johnson NP, Kirkeeide RL, Gould KL. Coronary anatomy to predict physiology: fundamental limits. Circ Cardiovasc Imaging. 2013; 6:817–832.
44. Kern MJ. Seeing and not believing: understanding the visual-functional mismatch between angiography and FFR. Catheter Cardiovasc Interv. 2014; 84:414–415.
45. Tonino PA, Fearon WF, De Bruyne B, Oldroyd KG, Leesar MA, Ver Lee PN, et al. Angiographic versus functional severity of coronary artery stenoses in the FAME study fractional flow reserve versus angiography in multivessel evaluation. J Am Coll Cardiol. 2010; 55:2816–2821.
46. Park SJ, Kang SJ, Ahn JM, Shim EB, Kim YT, Yun SC, et al. Visual-functional mismatch between coronary angiography and fractional flow reserve. JACC Cardiovasc Interv. 2012; 5:1029–1036.
47. Waksman R, Legutko J, Singh J, Orlando Q, Marso S, Schloss T, et al. FIRST: Fractional Flow Reserve and Intravascular Ultrasound Relationship Study. J Am Coll Cardiol. 2013; 61:917–923.
48. Chantadansuwan T, Kehasukcharoen W, Kanoksilp A, Saejueng B, Plainetr V, Sukhavasharin N, et al. Visual-functional mismatch and results of fractional flow reserve guided percutaneous coronary revascularization. J Med Assoc Thai. 2014; 97:1064–1076.
49. Cho YK, Nam CW, Han JK, Koo BK, Doh JH, Ben-Dor I, et al. Usefulness of combined intravascular ultrasound parameters to predict functional significance of coronary artery stenosis and determinants of mismatch. EuroIntervention. 2015; 11:163–170.
50. Cho HO, Nam CW, Cho YK, Yoon HJ, Park HS, Kim H, et al. Characteristics of function-anatomy mismatch in patients with coronary artery disease. Korean Circ J. 2014; 44:394–399.
51. Taylor CA, Fonte TA, Min JK. Computational fluid dynamics applied to cardiac computed tomography for noninvasive quantification of fractional flow reserve: scientific basis. J Am Coll Cardiol. 2013; 61:2233–2241.
52. Kochar M, Min JK. Physiologic assessment of coronary artery disease by cardiac computed tomography. Korean Circ J. 2013; 43:435–442.
53. Koo BK, Lee JM, Taylor C. Chapter 35. Computational fluid dynamics applied to multidetector coronary angiography (FFR-CT). In : Escaned J, Serruys PW, editors. Coronary stenosis: imaging, structure, and physiology. 2nd ed. EUROPA Digital & Publishing;2015.
54. Koo BK, Erglis A, Doh JH, Daniels DV, Jegere S, Kim HS, et al. Diagnosis of ischemia-causing coronary stenoses by noninvasive fractional flow reserve computed from coronary computed tomographic angiograms. Results from the prospective multicenter DISCOVER-FLOW (Diagnosis of Ischemia-Causing Stenoses Obtained Via Noninvasive Fractional Flow Reserve) study. J Am Coll Cardiol. 2011; 58:1989–1997.
55. Min JK, Leipsic J, Pencina MJ, Berman DS, Koo BK, van Mieghem C, et al. Diagnostic accuracy of fractional flow reserve from anatomic CT angiography. JAMA. 2012; 308:1237–1245.
56. Nørgaard BL, Leipsic J, Gaur S, Seneviratne S, Ko BS, Ito H, et al. Diagnostic performance of noninvasive fractional flow reserve derived from coronary computed tomography angiography in suspected coronary artery disease: the NXT trial (Analysis of Coronary Blood Flow Using CT Angiography: Next Steps). J Am Coll Cardiol. 2014; 63:1145–1155.
57. Hlatky MA, Saxena A, Koo BK, Erglis A, Zarins CK, Min JK. Projected costs and consequences of computed tomography-determined fractional flow reserve. Clin Cardiol. 2013; 36:743–748.
58. Kimura T, Shiomi H, Kuribayashi S, Isshiki T, Kanazawa S, Ito H, et al. Cost analysis of non-invasive fractional flow reserve derived from coronary computed tomographic angiography in Japan. Cardiovasc Interv Ther. 2015; 30:38–44.
59. Douglas PS, Pontone G, Hlatky MA, Patel MR, Norgaard BL, Byrne RA, et al. Clinical outcomes of fractional flow reserve by computed tomographic angiography-guided diagnostic strategies vs. usual care in patients with suspected coronary artery disease: the prospective longitudinal trial of FFRCT: outcome and resource impacts study. Eur Heart J. 2015; 36:3359–3367.
60. Kim KH, Doh JH, Koo BK, Min JK, Erglis A, Yang HM, et al. A novel noninvasive technology for treatment planning using virtual coronary stenting and computed tomography-derived computed fractional flow reserve. JACC Cardiovasc Interv. 2014; 7:72–78.
61. Coenen A, Lubbers MM, Kurata A, Kono A, Dedic A, Chelu RG, et al. Fractional flow reserve computed from noninvasive CT angiography data: diagnostic performance of an on-site clinician-operated computational fluid dynamics algorithm. Radiology. 2015; 274:674–683.
62. Kwon SS, Chung EC, Park JS, Kim GT, Kim JW, Kim KH, et al. A novel patient-specific model to compute coronary fractional flow reserve. Prog Biophys Mol Biol. 2014; 116:48–55.
63. Yang DH, Kim YH, Roh JH, Kang JW, Han D, Jung J, et al. Stress myocardial perfusion CT in patients suspected of having coronary artery disease: visual and quantitative analysis-validation by using fractional flow reserve. Radiology. 2015; 276:715–723.
64. Choi G, Lee JM, Kim HJ, Park JB, Sankaran S, Otake H, et al. Coronary artery axial plaque stress and its relationship with lesion geometry: application of computational fluid dynamics to coronary CT angiography. JACC Cardiovasc Imaging. 2015; 8:1156–1166.
65. Authors/Task Force members. Windecker S, Kolh P, Alfonso F, Collet JP, Cremer J, et al. 2014 ESC/EACTS Guidelines on myocardial revascularization: the task force on myocardial revascularization of the European Society of Cardiology (ESC) and the European Association for Cardio-Thoracic Surgery (EACTS) developed with the special contribution of the European Association of Percutaneous Cardiovascular Interventions (EAPCI). Eur Heart J. 2014; 35:2541–2619.
66. Toth G, Hamilos M, Pyxaras S, Mangiacapra F, Nelis O, De Vroey F, et al. Evolving concepts of angiogram: fractional flow reserve discordances in 4000 coronary stenoses. Eur Heart J. 2014; 35:2831–2838.
67. Camici PG, Crea F. Coronary microvascular dysfunction. N Engl J Med. 2007; 356:830–840.
68. van de Hoef TP, Bax M, Damman P, Delewi R, Hassell ME, Piek MA, et al. Impaired coronary autoregulation is associated with long-term fatal events in patients with stable coronary artery disease. Circ Cardiovasc Interv. 2013; 6:329–335.
69. van de Hoef TP, van Lavieren MA, Damman P, Delewi R, Piek MA, Chamuleau SA, et al. Physiological basis and long-term clinical outcome of discordance between fractional flow reserve and coronary flow velocity reserve in coronary stenoses of intermediate severity. Circ Cardiovasc Interv. 2014; 7:301–311.
70. Meuwissen M, Chamuleau SA, Siebes M, de Winter RJ, Koch KT, Dijksman LM, et al. The prognostic value of combined intracoronary pressure and blood flow velocity measurements after deferral of percutaneous coronary intervention. Catheter Cardiovasc Interv. 2008; 71:291–297.
71. Yong AS, Ho M, Shah MG, Ng MK, Fearon WF. Coronary microcirculatory resistance is independent of epicardial stenosis. Circ Cardiovasc Interv. 2012; 5:103–108. S1–S2.
72. Melikian N, Vercauteren S, Fearon WF, Cuisset T, MacCarthy PA, Davidavicius G, et al. Quantitative assessment of coronary microvascular function in patients with and without epicardial atherosclerosis. EuroIntervention. 2010; 5:939–945.
73. Echavarría-Pinto M, Gonzalo N, Ibañez B, Petraco R, Jimenez-Quevedo P, Sen S, et al. Low coronary microcirculatory resistance associated with profound hypotension during intravenous adenosine infusion: implications for the functional assessment of coronary stenoses. Circ Cardiovasc Interv. 2014; 7:35–42.
74. Luo C, Long M, Hu X, Huang Z, Hu C, Gao X, et al. Thermodilution-derived coronary microvascular resistance and flow reserve in patients with cardiac syndrome X. Circ Cardiovasc Interv. 2014; 7:43–48.
75. Cuisset T, Hamilos M, Melikian N, Wyffels E, Sarma J, Sarno G, et al. Direct stenting for stable angina pectoris is associated with reduced periprocedural microcirculatory injury compared with stenting after pre-dilation. J Am Coll Cardiol. 2008; 51:1060–1065.
76. Fearon WF, Shah M, Ng M, Brinton T, Wilson A, Tremmel JA, et al. Predictive value of the index of microcirculatory resistance in patients with ST-segment elevation myocardial infarction. J Am Coll Cardiol. 2008; 51:560–565.
77. McGeoch R, Watkins S, Berry C, Steedman T, Davie A, Byrne J, et al. The index of microcirculatory resistance measured acutely predicts the extent and severity of myocardial infarction in patients with ST-segment elevation myocardial infarction. JACC Cardiovasc Interv. 2010; 3:715–722.
78. Fujii K, Kawasaki D, Oka K, Akahori H, Iwasaku T, Fukunaga M, et al. The impact of pravastatin pre-treatment on periprocedural microcirculatory damage in patients undergoing percutaneous coronary intervention. JACC Cardiovasc Interv. 2011; 4:513–520.
79. Layland JJ, Whitbourn RJ, Burns AT, Somaratne J, Leitl G, Macisaac AI, et al. The index of microvascular resistance identifies patients with periprocedural myocardial infarction in elective percutaneous coronary intervention. Heart. 2012; 98:1492–1497.
80. Ng MK, Yong AS, Ho M, Shah MG, Chawantanpipat C, O'Connell R, et al. The index of microcirculatory resistance predicts myocardial infarction related to percutaneous coronary intervention. Circ Cardiovasc Interv. 2012; 5:515–522.
81. Fearon WF, Low AF, Yong AS, McGeoch R, Berry C, Shah MG, et al. Prognostic value of the Index of Microcirculatory Resistance measured after primary percutaneous coronary intervention. Circulation. 2013; 127:2436–2441.
82. Jeremias A, Whitbourn RJ, Filardo SD, Fitzgerald PJ, Cohen DJ, Tuzcu EM, et al. Adequacy of intracoronary versus intravenous adenosine-induced maximal coronary hyperemia for fractional flow reserve measurements. Am Heart J. 2000; 140:651–657.
83. De Bruyne B, Pijls NH, Barbato E, Bartunek J, Bech JW, Wijns W, et al. Intracoronary and intravenous adenosine 5’-triphosphate, adenosine, papaverine, and contrast medium to assess fractional flow reserve in humans. Circulation. 2003; 107:1877–1883.
84. Koo BK, Kim CH, Na SH, Youn TJ, Chae IH, Choi DJ, et al. Intracoronary continuous adenosine infusion. Circ J. 2005; 69:908–912.
85. Yoon MH, Tahk SJ, Yang HM, Park JS, Zheng M, Lim HS, et al. Comparison of the intracoronary continuous infusion method using a microcatheter and the intravenous continuous adenosine infusion method for inducing maximal hyperemia for fractional flow reserve measurement. Am Heart J. 2009; 157:1050–1056.
86. Seo MK, Koo BK, Kim JH, Shin DH, Yang HM, Park KW, et al. Comparison of hyperemic efficacy between central and peripheral venous adenosine infusion for fractional flow reserve measurement. Circ Cardiovasc Interv. 2012; 5:401–405.
87. Arumugham P, Figueredo VM, Patel PB, Morris DL. Comparison of intravenous adenosine and intravenous regadenoson for the measurement of pressure-derived coronary fractional flow reserve. EuroIntervention. 2013; 8:1166–1171.
88. Prasad A, Zareh M, Doherty R, Gopal A, Vora H, Somma K, et al. Use of regadenoson for measurement of fractional flow reserve. Catheter Cardiovasc Interv. 2014; 83:369–374.
89. van Nunen LX, Lenders GD, Schampaert S, van't Veer M, Wijnbergen I, Brueren GR, et al. Single bolus intravenous regadenoson injection versus central venous infusion of adenosine for maximum coronary hyperaemia in fractional flow reserve measurement. EuroIntervention. 2015; 11:905–913.