Abstract
Objective
Our aim was to evaluate the cartilage cap of osteochondromas using T2 maps and to compare these values to those of normal patellar cartilage, from age and gender matched controls.
Materials and Methods
This study was approved by the Institutional Review Board and request for informed consent was waived. Eleven children (ages 5-17 years) with osteochondromas underwent MR imaging, which included T2-weighted fat suppressed and T2 relaxation time mapping (echo time = 9-99/repetition time = 1500 msec) sequences. Lesion origins were femur (n = 5), tibia (n = 3), fibula (n = 2), and scapula (n = 1). Signal intensity of the cartilage cap, thickness, mean T2 relaxation times, and T2 spatial variation (mean T2 relaxation times as a function of distance) were evaluated. Findings were compared to those of patellar cartilage from a group of age and gender matched subjects.
Results
The cartilage caps showed a fluid-like high T2 signal, with mean thickness of 4.8 mm. The mean value of mean T2 relaxation times of the osteochondromas was 264.0 ± 80.4 msec (range, 151.0-366.0 msec). Mean T2 relaxation times were significantly longer than the values from patellar cartilage (39.0 msec) (p < 0.0001). These findings were observed with T2 spatial variation plots across the entire distance of the cartilage cap, with the most pronounced difference in the middle section of the cartilage.
Osteochondroma is the most common skeletal neoplasm in children, and accounts for 20-50% of benign bone tumors, having an incidence of 1:200 (12). The lesion is characterized as a bony excrescence having a cartilaginous cap, and showing corticomedullary continuity with the underlying bone (3).
Normally, the cartilage cap of an osteochondroma has a characteristic hyperintense T2-weighted signal on magnetic resonance (MR) imaging. A cartilage cap thickness greater than 2 cm in adults and 3 cm in children, and/or renewed growth of a dormant lesion, have been recognized as warning signs of malignant degeneration. Malignant degeneration of osteochondromas has rarely been reported in solitary osteochondromas (less than 1%) (4), but has a higher incidence in patients with hereditary multiple osteochondromatosis (less than 5%) (3).
T2 relaxation time mapping (T2 map) is a quantitative MR imaging technique that has been widely used to probe early biochemical changes of articular and epiphyseal cartilage, such as the evaluation of compositional changes and anisotropy of collagen fibers (5). T2 relaxation times reflect the water mobility of cartilage; they are proportional to the water content, but inversely proportional to proteoglycan concentration (5). Application of this quantitative technique has been extended to the pediatric population for the evaluation of articular cartilage and physes (678). However, to our knowledge, the use of quantitative imaging to evaluate cartilage containing neoplasms has not been reported previously.
The purpose of our study was to characterize the T2 relaxation times of the cartilage caps of osteochondromas, and to compare these values to those derived from normal hyaline cartilage of patellae, in age and gender matched children. The study of these T2 maps may prove beneficial for possible application to other cartilage containing neoplasms. Additionally, this quantitative analysis might prove to be useful for the detection of malignant degeneration in these lesions.
This retrospective study was approved by the Institutional Review Board, and was Health Insurance Portability and Accountability Act compliant. The requirement for informed consent was waived.
A total of 11 osteochondromas from 11 children (7 boys, 4 girls; ages 5 to 17 years, mean age 12 years) were included in this study. Among the 11 cases, 6 lesions were surgically removed and pathologically confirmed as osteochondromas. The other 5 were presumed to be osteochondromas based on clinical evaluation and radiologic studies. Solitary lesions were seen in 7 children, and 4 had lesions that were in the setting of hereditary multiple osteochondromatosis. Locations of the lesions were 5 from the femur, 3 from the tibia, 2 from the fibula and 1 from the scapula.
During skeletal maturation in children, epiphyseal and articular cartilage has a sequential age and gender dependent shortening of T2 relaxation times (6). Therefore, the control group was selected from age and gender matched subjects using the following criteria: 1) each child had undergone an MR imaging examination of the knee performed at our institution for clinical indications, 2) the MR imaging examination included at least fast spin echo fat suppressed T2-weighted and T2 mapping sequences, 3) the MR imaging report and images showed no abnormal signal intensity nor morphologic changes of the patellar cartilage, nor findings of joint instability or internal derangement. Of the 132 subjects imaged between 2011 and 2013 who met our inclusion criteria, age and gender matched subjects were randomly selected to fulfill the 11 subjects needed for the control group. The knee MR exam was selected since it is the study with the largest volume and T2 relaxation time mapping in our institution.
All MR imaging examinations of the osteochondromas were performed for clinical indications using a 1.5T MR imaging system (Signa Excite, GE Healthcare, Milwaukee, WI, USA). Imaging coils were selected based on the body part of interest and patient size. Each examination included at least a T2-weighted sequence with fat suppression, with the following parameters: repetition time (TR)/echo time (TE), 3000/60 msec; echo train length, 8; slice thickness, 4 mm; slice gap, 1 mm; matrix, 256 × 192, number of excitation, 2. The field of view varied based on the lesion size.
Similarly, the MR imaging examinations of the knees in the control group were performed for clinical indications with a 1.5T MR imaging system (Signa Excite, GE Healthcare, Milwaukee, WI, USA) using a knee coil. Each examination included an axial fast spin echo T2-weighted sequence with fat suppression. Any patella that showed abnormal signal intensity or morphology of the cartilage was excluded, upon evaluation by a single reviewer.
The T2 mapping sequence was performed with the same parameters for both groups of patients. For patients with osteochondromas, T2 maps were obtained in the sagittal, axial or coronal plane, whichever best showed the maximum thickness of the cartilage cap. In the control group, T2 maps were obtained in the axial plane only. The parameters for the T2 mapping included a multislice (at least 6 slices, to include the entire area of interest) multiecho sequence with TE = 9, 18, 27, 45, 54, 63, 72, 81, 90, and 99 msec; TR, 1500 msec; echo train length, 9; slice thickness, 3 mm; gap, 1.5 mm; matrix, 256 × 160; signal average, 1. The acquisition time for the T2 mapping sequence was approximately 5 minutes. No patient required sedation for the MR imaging examination. All the T2 maps were generated and reviewed by a single author, having both pediatric and musculoskeletal radiology fellowship training and 13 years of experience, to confirm appropriate image quality (Fig. 1).
All the 11 osteochondromas were evaluated with T2 maps in vivo. Six lesions were surgically resected.
T2 maps were generated and calculated on a pixel-by-pixel basis, using a linear least squares curve fitting algorithm and a mono-exponential function for each pixel. Data was analyzed sequentially by the same radiologist who had 9 years of experience in T2 map generation and evaluation. An institutional software program written in Interactive Data Language (IDL, ISS, Boulder, CO, USA) was used. The T2 maps were color coded pixel-by-pixel, with each color a reflection of a range of T2 values in msec (Fig. 1B). To avoid partial volume artifact, the first and last images were discarded, and the remaining images were utilized for placement of the regions of interest (ROI). On the gray scale map, the ROI was obtained by manually tracing the outline of the cartilage cap. Placement of the ROI on the cartilage cap automatically calculated a mean T2 relaxation time. Because articular cartilage has a spatial variation of T2 relaxation times as a function of distance, and the cartilage caps had different thicknesses, the ROI was normalized from distance 0 (osteochondral junction) to 1 (articular surface for the patella or external surface for the osteochondroma). The color scale ranges from 0 to 100 msec (Fig. 1A). Mean T2 relaxation time profiles at 20 normalized distances were generated and used to define the T2 spatial variation of each case.
In the control group, the ROI was placed on the axial T2 map of the patellar cartilage to include both articular and non-ossified epiphyseal cartilage. The mean T2 values and T2 spatial variation were obtained. This method has been utilized in several prior cartilage imaging studies with reliable results (89).
On T2-weighted sequence with fat suppression images, signal intensity of the cartilage cap was compared to the signal intensity of the cartilage of the patella and knee joint effusion from the age and gender matched control group. The cartilage cap was deemed hypo-, iso-, or hyperintense in signal, compared to the patellar cartilage. The thickness of the cartilage cap was measured on the T2 fat suppressed images.
Mean T2 relaxation times and T2 spatial variation of cartilage cap were compared to the values of patellar cartilage from age and gender matched subjects. A mixed model repeated measures analysis of variance was utilized to compare mean values of mean T2 relaxation times between the two groups across all the 20 distances of the cartilage thickness. The p values less than 0.05 were considered statistically significant. No adjustments were made for multiple comparisons for the mixed model (this was unnecessary since the p values were well below the Bonferroni threshold). All statistical analyses were performed using SAS® statistical software package, version 9.3 (SAS Institute, Cary, NC, USA).
All 11 osteochondroma caps showed hyperintense T2-weighted signal intensity similar to that of knee joint effusion (Fig. 2). The signal intensity of each cartilage cap was hyperintense to that of the articular and epiphyseal cartilage of the patella in the corresponding control patients. The mean thickness of the cartilage cap was 4.8 mm (range, 2.3 to 11.1 mm; standard deviation, 2.3 mm) (Table 1).
The mean T2 relaxation times from the 11 cartilage caps was 264.4 ± 80.4 msec (range, 151.0 to 366.0 msec). Mean T2 relaxation time of the patellar cartilage from the control group was 39.0 ± 5.0 msec (range, 28.1 to 45.8 msec) (Table 1). The mean of the mean T2 relaxation times of the cartilage cap of osteochondromas was significantly longer than the values from patellar cartilage (p < 0.0001). Longer T2 relaxation times were observed across the entire distance of cartilage (p < 0.0001) (Figs. 2, 3). The difference was most pronounced in the middle portion of the cartilage (normalized distance between 0.4 and 0.8).
The etiology of an osteochondroma is thought to be due to an initial separation of a cartilage fragment from a physis. This cartilaginous focus subsequently herniates through the adjacent periosteum, which normally encircles the outer margin of the physis (10). Continuous growth occurs from enchondral ossification, which eventually forms a subperiosteal osseous excrescence with a cartilage cap (10). Enchondral ossification occurs at the base of the cartilage cap, which is equivalent to the physis of normally growing bone. Growth of an osteochondroma usually ceases with skeletal maturation (1341011).
The cartilage cap of an osteochondroma is composed of hyaline cartilage (12), which comprises of articular and epiphyseal cartilage as well as physes (1314). Hyaline cartilage is characterized by chondrocytes surrounded by a larger component of extra-cellular matrix (ECM). The ECM, produced by chondrocytes, is comprised primarily of water (70%), type II collagen, and proteoglycans (1415). Although articular, epiphyseal and physeal cartilage, and the cartilage caps of osteochondromas are made up of hyaline cartilage, their microscopic composition and organization of cellular components are different (101617).
A previous study that utilized T2 maps of the knee in children (age range 5 to 17 years) showed that the longest T2 relaxation time observed at the physis of the femur ranged from 70-87 msec. Shorter values were observed from the articular and epiphyseal cartilage (40-85 msec). The shortest values were seen within the patellar cartilage (37-54 msec) (8). In general, T2 values of patellar cartilage decrease with age, and the values are higher in boys than in girls (6). Even if we consider the published differences of patellar cartilage T2 values between genders and at different ages, all cartilage cap T2 values in our study are much longer than the previously published values of any cartilage in growing children (18).
Longer T2 relaxation times of the cartilage caps from osteochondromas may reflect a difference of the ECM of this cartilage, compared to articular, epiphyseal or physeal cartilage. We postulate that the greater water content present in the cartilage cap, than in other forms of hyaline cartilage, may be a substantial contributor. This also is supported by the observation of hyperintense T2-weighted signal intensity, consistent with fluid (11). T2 relaxation time is highly sensitive to the orientation of the collagen fibers. Different orientations of the collagen fiber in the cartilage cap may contribute to longer T2 relaxation time. However, contribution from the difference in the ECM microstructure between the cartilage cap and other hyaline-based structures, such as collagen fiber anisotropy and proteoglycan content, remains unknown.
Our study has several limitations. The sample size is small, and only 11 cases were evaluated in vivo. The thickness of the cartilage cap of an osteochondroma is variable. In young patients, it is typically between 1 and 3 cm, whereas in adults, it may be as thin as a few millimeters or even completely absent (3). The thicker cartilage cap in the younger children is likely to be a reflection of the process of endochondral ossification from an active physis (10). Therefore, the age factor is likely to affect the T2 relaxation time of the cartilage cap. In our study, only 2 subjects were older than 15 years, which is the age when physes are presumed to be closing (6). A future study with a larger sample size of osteochondromas would be needed to achieve an equal distribution of patients with open versus closed physes. Additionally, we did not differentiate between sporadic and syndromic osteochondromas.
Although 6 subjects underwent surgery to confirm the diagnosis of an osteochondroma, the remaining 5 were given the diagnosis based on clinical evaluation and imaging findings. None of the cartilage caps were compared histologically to normal hyaline articular, epiphyseal or physeal cartilage. Therefore, the contribution of various components to the lengthening of T2 values in the cartilage caps is unknown.
T2 relaxation time mapping is highly sensitive to water content and collagen microstructure changes of cartilage; however, it is relatively insensitive to proteoglycan changes. Future studies using other quantitative cartilage imaging techniques reflecting proteoglycan or microscopic evaluation of pathological specimens might be useful to explain the difference between cartilage cap and normal hyaline cartilage.
The cartilage cap of an osteochondroma can undergo malignant transformation to chondrosarcoma. A previously reported factor to suggest malignant transformation in adults is a cartilage cap thickness of greater than 2 cm (18). However, in children a cartilage cap can be up to 3 cm thick, and remain benign (18). Therefore, it might prove useful to evaluate with future longitudinal studies, whether quantitative T2 mapping can be a tool used to monitor cartilage cap microstructure and thus possibly herald transformation to a malignancy.
In conclusion, we found that the cartilage caps of osteochondromas have T2 relaxation times across the entire cartilage thicknesses significantly higher than the values obtained from other forms of hyaline cartilage. This longer T2 relaxation time should be considered a normal MR imaging finding for the cartilage cap of osteochondromas, and likely to be related to high water content and different microstructures.
Figures and Tables
Fig. 1
T2 relaxation time mapping from 12-year-old boy with osteochondroma of femur.
Gray scale map (A) and color-coded map (B) of distal femur. (A) Region of interest of cartilage cap was manually outlined on gray scale map. Mean T2 value of cartilage cap was 201.0 ± 35.4 msec, value of patellar cartilage was 40.4 ± 4.4 msec.
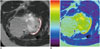
Fig. 2
9-year-old girl with osteochondroma of right proximal tibia (A, B) and 9-year-old girl in control group (C, D).
A. Axial T2-weighted image with fat suppression demonstrates hyperintense signal of cartilage cap (arrows), which is similar to signal intensity of joint effusion of knee in age and gender matched control (C). C. Axial fast spin echo T2-weighted image with fat suppression demonstrates other forms of hyaline cartilage: unossified epiphyseal cartilage with low signal intensity (arrowheads), and articular cartilage with intermediate signal intensity (arrows). Axial color coded T2 maps of osteochondroma (B) and patellar cartilage (D) demonstrate different range of T2 relaxation times. Mean T2 values of cartilage cap in osteochondroma (172.8 ± 23.1 msec) were significantly longer than values (38.2 ± 5.2 msec) of patellar cartilage in control group.
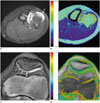
Fig. 3
T2 spatial variation of cartilage cap obtained from 11 subjects with osteochondromas, and from patellar cartilage of age and gender matched controls.
T2 relaxation times were significantly longer in cartilage caps across entire distance of cartilage (p < 0.0001). Difference is most prominent in middle portion of cartilage (at normalized distance between 0.4 and 0.8) where 0 represents osteochondral junction in osteochondroma and subchondral bone in patella; 1 represents external surface in osteochondroma and articular surface in patellar cartilage.
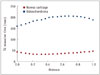
Table 1
Demographics and Summary of T2 Relaxation Times in Group of Osteochondromas and Control
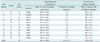
References
1. Giudici MA, Moser RP Jr, Kransdorf MJ. Cartilaginous bone tumors. Radiol Clin North Am. 1993; 31:237–259.
2. Scarborough MT, Moreau G. Benign cartilage tumors. Orthop Clin North Am. 1996; 27:583–589.
3. Murphey MD, Choi JJ, Kransdorf MJ, Flemming DJ, Gannon FH. Imaging of osteochondroma: variants and complications with radiologic-pathologic correlation. Radiographics. 2000; 20:1407–1434.
4. Woertler K, Lindner N, Gosheger G, Brinkschmidt C, Heindel W. Osteochondroma: MR imaging of tumor-related complications. Eur Radiol. 2000; 10:832–840.
5. Mosher TJ, Dardzinski BJ. Cartilage MRI T2 relaxation time mapping: overview and applications. Semin Musculoskelet Radiol. 2004; 8:355–368.
6. Kim HK, Shiraj S, Anton CG, Horn PS, Dardzinski BJ. Age and sex dependency of cartilage T2 relaxation time mapping in MRI of children and adolescents. AJR Am J Roentgenol. 2014; 202:626–632.
7. Kim HK, Laor T, Graham TB, Anton CG, Salisbury SR, Racadio JM, et al. T2 relaxation time changes in distal femoral articular cartilage in children with juvenile idiopathic arthritis: a 3-year longitudinal study. AJR Am J Roentgenol. 2010; 195:1021–1025.
8. Dardzinski BJ, Laor T, Schmithorst VJ, Klosterman L, Graham TB. Mapping T2 relaxation time in the pediatric knee: feasibility with a clinical 1.5-T MR imaging system. Radiology. 2002; 225:233–239.
9. Shiraj S, Kim HK, Anton C, Horn PS, Laor T. Spatial variation of T2 relaxation times of patellar cartilage and physeal patency: an in vivo study in children and young adults. AJR Am J Roentgenol. 2014; 202:W292–W297.
10. Milgram JW. The origins of osteochondromas and enchondromas. A histopathologic study. Clin Orthop Relat Res. 1983; (174):264–284.
11. Kitsoulis P, Galani V, Stefanaki K, Paraskevas G, Karatzias G, Agnantis NJ, et al. Osteochondromas: review of the clinical, radiological and pathological features. In Vivo. 2008; 22:633–646.
12. Mirra JM. Benign cartilaginous exostoses: osteochondroma and osteochondromatosis. In : Mirra JM, editor. Bone tumors: clinical, radiologic, and pathologic correlations. Vol 2. Philadelphia, PA: Lea & Febiger;1989. p. 1626–1659.
13. Maroudas A, Bullough P. Permeability of articular cartilage. Nature. 1968; 219:1260–1261.
14. Maroudas A, Bullough P, Swanson SA, Freeman MA. The permeability of articular cartilage. J Bone Joint Surg Br. 1968; 50:166–177.
15. Martel-Pelletier J, Boileau C, Pelletier JP, Roughley PJ. Cartilage in normal and osteoarthritis conditions. Best Pract Res Clin Rheumatol. 2008; 22:351–384.
16. de Andrea CE, Wiweger M, Prins F, Bovée JV, Romeo S, Hogendoorn PC. Primary cilia organization reflects polarity in the growth plate and implies loss of polarity and mosaicism in osteochondroma. Lab Invest. 2010; 90:1091–1101.
17. Huch K, Mordstein V, Stöve J, Nerlich AG, Amholdt H, Delling G, et al. Expression of collagen type I, II, X and Ki-67 in osteochondroma compared to human growth plate cartilage. Eur J Histochem. 2002; 46:249–258.
18. Bernard SA, Murphey MD, Flemming DJ, Kransdorf MJ. Improved differentiation of benign osteochondromas from secondary chondrosarcomas with standardized measurement of cartilage cap at CT and MR imaging. Radiology. 2010; 255:857–865.