Abstract
Objective
Hyperintense acute reperfusion marker (HARM) without diffusion abnormalities is occasionally found in patients with an acute stroke. This study was to determine the prevalence and clinical implications of HARM without diffusion abnormalities.
Materials and Methods
There was a retrospective review of magnetic resonance images 578 patients with acute strokes and identified those who did not have acute infarction lesions, as mapped by diffusion-weighted imaging (DWI). These patients were classified into an imaging-negative stroke and HARM without diffusion abnormalities groups, based on the DWI findings and postcontrast fluid attenuated inversion recovery images. The National Institutes of Health Stroke Scale (NIHSS) scores at admission, 1 day, and 7 days after the event, as well as clinical data and risk factors, were compared between the imaging-negative stroke and HARM without diffusion abnormalities groups.
Results
Seventy-seven acute stroke patients without any DWI abnormalities were found. There were 63 patients with an imaging-negative stroke (accounting for 10.9% of 578) and 13 patients with HARM without diffusion abnormalities (accounting for 2.4% of 578). The NIHSS scores at admission were higher in HARM without diffusion abnormalities group than in the imaging-negative stroke group (median, 4.5 vs. 1.0; p < 0.001), but the scores at 7 days after the event were not significantly different between the two groups (median, 0 vs. 0; p = 1). The patients with HARM without diffusion abnormalities were significantly older, compared with patients with an imaging-negative stroke (mean, 73.1 years vs. 55.9 years; p < 0.001).
The hyperintense acute reperfusion marker (HARM) is known as a delayed enhancement of cerebrospinal fluid (CSF) on fluid attenuated inversion recovery (FLAIR) images in an acute stroke, which are supposed to indicate a blood brain barrier (BBB) disruption (123). Classic HARM occurs at a mean time of 12.9 hours after the onset of ischemia, and has been observed on the second magnetic resonance image (MRI) followed by the first postcontrast MRI in acute stroke patients (45678). At the institution of this study, the postcontrast FLAIR images obtained in the initial brain MRI for an acute stroke allowed researchers to be cognizant of localized linear contrast leakage along the brain surface in patients with an acute stroke. Thus, it is hypothesized that this linear contrast enhancement on initial postcontrast FLAIR images is an early HARM sign in acute stroke patients.
In daily practice, medical scientists occasionally encounter early HARM signs without diffusion abnormalities, among patients with acute stroke-like symptoms. The neurological symptoms of these patients were transient and reversible, and a CSF enhancement was resolved on follow-up MRIs acquired several days after the onset. These patients seemed to follow a clinical course that was similar to that of patients with a transient ischemic attack (TIA). Hence, this study aimed to determine the prevalence of HARM without diffusion abnormalities in patients who underwent clinical pathways for an acute stroke. also sought to determine the clinical implications of HARM without diffusion abnormalities.
This retrospective study was approved by the appropriate Institutional Review Board. The requirement to obtain an informed consent was waived by the board. Over the last two years, there was a selection of 774 consecutive patients who had presented to this institution with symptoms of acute stroke and had undergone a standardized brain MRI according to the clinical pathway for acute stroke. There was a retrospective review of the brain MRIs of these patients and hence 117 patients were excluded, including those with dizziness, seizure, or systemic disorders such as drug intoxication or other metabolic diseases, and 64 patients with intracerebral hemorrhage, subdural hemorrhage, or brain tumor. In addition, 15 patients, with HARM mimicking imaging findings such as subarachnoid hemorrhage (n = 8), leptomeningeal seeding (n = 4), or meningitis (n = 3) were excluded. The remaining 578 patients with acute stroke or TIA were further analyzed.
The MRIs of the 578 patients were reviewed by two neuroradiologists who were blinded to the clinical information. An acute cerebral infarction was defined as the presence of a diffusion restriction lesion on initial or follow-up MRIs. An early HARM sign was defined as the linear contrast enhancement along the brain surface on the postcontrast FLAIR image of the initial brain MRI.
Among the 578 patients with acute stroke or TIA, 501 patients (86.7%) had acute infarction lesions on the diffusion-weighted images (DWIs). Of the 77 patients without acute infarction lesions on the DWIs, the presence of early HARM signs on FLAIR images motivated the researchers to divide the patients into two groups, namely the imaging-negative stroke group and the HARM without diffusion abnormalities group. In detail, an imaging-negative stroke was defined as the absence of acute infarction on the DWIs and early HARM signs on postcontrast FLAIR images, while HARM without diffusion abnormalities was defined as the absence of acute infarction on DWIs with the presence of early HARM signs on postcontrast FLAIR images. Figure 1 shows a flow chart of the selection process for patients in this study.
MRI was performed on patients who were suspected of presenting with a stroke using 1.5-T or 3-T MRI scanners (Intera Achieva; Philips Medical Systems, Best, the Netherlands). The initial MRI scanning protocols of the clinical pathway for an acute stroke were standardized for the sequence parameters and order of acquisition; the following procedures were performed in order: DWI, gradient-echo imaging, time of flight MR angiography (MRA) of intracranial arteries and neck vessels, perfusion-weighted imaging (PWI), axial postcontrast T1-weighted imaging (T1WI), and axial postcontrast FLAIR imaging. The researchers first performed diffusion-weighted imaging, time of flight MR angiography of intracranial arteries and neck vessels, and perfusion MRI, which are crucial for acute stroke patients when considering the possibility of performing an intra-arterial thrombolysis. Then, T1WI and FLAIR imaging are sequentially obtained to evaluate the parenchymal lesion of a brain after contrast injection. Hereinafter, there is a description of only a portion of the imaging results in detail.
A DWI was performed in the axial plane with the following parameters: repetition time (TR)/echo time (TE) = 3800-5000/45-60 ms; gradient echo image with TR/TE = 690-1100/15-24 ms. Both the intracranial and neck time-of-flight MRAs were performed in the axial plane with TR/TE = 20-25/3-7 ms. A dynamic susceptibility contrast PWI was performed in patients with glomerular filtration rate of more than 40 mL/min, as follows. A gadolinium-based agent (Gadovist; Bayer HealthCare, Leverkusen, Germany) was intravenously administered at a dose of 0.2 mM/kg of body weight at a rate of 4 mL/s, followed by a 20-mL saline flush. The total amount of contrast used was 15 mL. The standard echo-planar images were consecutively acquired with TR/TE = 1500/30-50 ms. Then, postcontrast axial T1WIs were obtained using a spin echo sequence with TR/TE = 400-600/9-12 ms, and postcontrast axial FLAIR images were obtained using a turbo-spin echo sequence with the following parameters: TR/TE = 11000/120-140 ms, flip angle = 90°, field of view = 216 × 240 mm, acquisition matrix = 256 × 162, slice thickness = 5 mm (1 mm gap between slices). The average time from contrast administration to postcontrast FLAIR acquisition was 5 minutes.
Follow-up MRIs for patients with an acute stroke were performed 3 to 7 days after the stroke event and were standardized with an axial DWI, axial noncontrast FLAIR imaging, axial gradient echo imaging, coronal T1WI, and contrast-enhanced MRA.
For all patients, a neurologist obtained the National Institutes of Health Stroke Scale (NIHSS) score at the time of emergency room admission, and 1 day and 7 days after the stroke event. In addition, 90 days after the stroke event, the scores from the modified Rankin scale (mRS) were analyzed to evaluate the disease severity and a patient's short-term outcome. To evaluate their associations with early HARM, demographic data and potential risk factors of acute stroke were obtained from the patients, including age, sex, hypertension, diabetes, hyperlipidemia, smoking, and atrial fibrillation. Initial vital signs, including systolic and diastolic blood pressure, heart rate, respiration rate, and body temperature, were also analyzed to evaluate associations with early HARM.
There was a comparison between the HARM without diffusion abnormalities group with the image-negative stroke group concerning their disease severity, prognosis, risk factors of stroke, and initial vital signs. A statistical analyses were performed using the PASW statistics 18 software (SPSS Inc., Chicago, IL, USA). Statistical differences among these groups were examined using the Pearson chi-square tests, t tests, and Mann-Whitney tests. The statistical significance was defined as p < 0.05.
In order to evaluate the HARM without diffusion abnormalities group more in depth, there was a review of their chief complaints, clinical findings, and the time interval between the stroke event and the initial MRI. The type (focal or diffuse) and location of HARM on initial MRIs were analyzed. Follow-up MRIs were also reviewed to determine if there were any significant changes.
Among the 578 patients with acute stroke or TIA, 501 patients (86.7%) had acute infarction lesions on DWIs, and early HARM signs on postcontrast FLAIR images were found in 70 patients (12.1%). Of the 77 patients without diffusion abnormalities, the imaging-negative stroke group accounted for 63 patients (10.9% out of the 578 patients), who were all clinically classified to have TIA, while out of the other 14 cases (2.4% out of the 578 patients), 13 patients were part of the HARM without diffusion abnormalities group. HARM without diffusion abnormalities accounted for 19% of the 70 patients with early HARM signs.
Comparisons between the imaging-negative stroke group and the HARM without diffusion abnormalities group are shown in Table 1. The mean age of the HARM without diffusion abnormalities group was significantly higher than that of patients in the imaging-negative stroke group. The NIHSS scores at admission after the event were higher in the HARM without diffusion abnormalities group than in the imaging-negative stroke group. However, there were no significant differences in the NIHSS scores at 7 days after the event, in the mRS 3 months after the event, other demographic data (except age), potential risk factors, or the initial vital signs between these two groups.
Table 2 shows the detailed clinical information of all cases of HARM without diffusion abnormalities. In total, 14 episodes of HARM without diffusion abnormalities occurred in 13 patients. One patient had two episodes of HARM without diffusion abnormalities within 2 years, with the second episode occurring 3 months after a complete recovery from the first attack. None of the patients had undergone an intravenous tissue plasminogen activator treatment. The patients presented with various chief complaints and most showed vague symptoms that could not be localized neurologically. Furthermore, their symptoms did not seem to be relevant to the types or locations of HARM. The initial MRIs showed no significant abnormalities on DWI, PWI, or MRA, except for early HARM signs in these patients. No intracranial hemorrhages were found on gradient echo images in any of the patients. The follow-up MRI protocol was not standardized with respect to time. A total of nine patients out of 14 episodes with early HARM sign underwent follow-up MRIs; follow-up MRIs were performed within 24 hours after the stroke-like episode in three patients, after 24 hours in four patients, and both within 24 hours and after 24 hours in two patients. In patients with early HARM signs, focal linear contrast leakage on initial postcontrast FLAIR imaging became diffuse in follow-up MRIs performed within the next 24 hours (Fig. 2), while patients whose follow-up MRIs were obtained between 24 hours and 7 days after the initial MRIs showed a small amount of contrast leakage or no detectable contrast in the CSF space (Fig. 3). Contrast leakage was seen in every five patient who underwent follow-up MRI within 24 hours and remained in only one of the six patients whose follow-up MRIs were performed between 24 hours and 7 days. None of the patients had symptoms or signs suggesting meningitis or leptomeningeal seeding, which can show similar MRI features to those of early HARM, and three patients underwent CSF studies that yielded negative results. All patients of HARM without diffusion abnormalities reached full recovery with this conservative management.
Hyperintense acute reperfusion marker is thought to be a useful marker for indicating BBB disruption in an acute stroke, because gadolinium does not cross the intact BBB (123). The contrast medium is believed to cross the BBB injury site after reperfusion, enter the perivascular space, and then diffuse into the CSF via a perivascular pathway (9). A proposed mechanism for the BBB disruption after ischemia or reperfusion is the activation of inflammatory cascades and proteolytic enzymes (10).
Although HARM is known to be associated with hemorrhagic transformation and poor clinical outcome (6), HARM was not associated with the hemorrhagic transformation of an acute stroke, as in a recent study on the elderly (8). A few studies have reported that 18-23% of patients without DWI abnormalities had HARM (810). However, HARM without diffusion abnormalities was found not only in patients with an acute stroke, but also in patients with cardiac surgery, carotid stenting, or seizure and hemianopsia in nonketotic hyperglycemia (91112). It seems that BBB disruption may be associated with transient hemodynamic alterations, besides acute ischemia or reperfusion injury. Thus, the supposed course of HARM, without diffusion abnormalities, is that an indiscernible cortical infarction or sudden hemodynamic change causes a focal BBB injury, which leads to contrast leakage into the CSF space. This series of processes may lead to transient severe neurologic deterioration. In this study, an attempt was made to determine the prevalence and clinical characteristics of HARM without diffusion abnormalities in patients by comparing them to patients with imaging-negative stroke.
In this study, HARM without diffusion abnormalities was found in 2.4% of patients with acute stroke or TIA, and it accounted for 19% of the 70 patients with early HARM signs. The patients, with HARM without diffusion abnormalities, showed favorable short-term outcomes similar to the outcomes of patients with an imaging-negative stroke, but showed more severe neurologic deterioration at the time of admission than the imaging-negative stroke group. In addition, the patients, with HARM without diffusion abnormalities, were significantly older compared with patients with an imaging-negative stroke. HARM without diffusion abnormalities appears to be reversible, but patients may have recurrent episodes. Thus, it is suggested that HARM without diffusion abnormalities is a specific type of TIA or stroke-mimicker, which is frequently seen in the elderly. Previous studies have shown that HARM cohorts were significantly older in age, implying that normal aging was an independent predictor of HARM (46891013). In addition, Farrall and Wardlaw (14) suggested that the BBB becomes more permeable with normal aging. Together with these results, this study may account for the HARM without diffusion abnormalities that are often seen in elderly patients.
There are several limitations to this study due to its retrospective cross-sectional nature. Firstly, initially the researchers had little experience with patients showing HARM without diffusion abnormalities. Thus, the patients with HARM without diffusion abnormalities had not been diagnosed or managed properly. Some of them were regarded as having meningitis or leptomeningeal seeding, and the others were underdiagnosed. It may be a potential confounder in the actual prevalence of vascular events in this cohort. Secondly, clinical work-ups and follow-up MRIs were not consistent across the HARM without diffusion abnormalities group. Lastly, early HARM signs on postcontrast FLAIR images were not specific for acute stroke, as they also look like meningitis or leptomeningeal seeding. These mimickers were excluded based on their clinical profiles and the CSF findings in this study. Unfortunately only 3 patients underwent CSF examination; therefore SAH was not entirely excluded in the rest of the patients. Although this retrospective study has some limitations, it showed that patients with HARM without diffusion abnormalities follow a clinical course that was similar to that of patients with TIA, and they can be mis- or under-diagnosed without the use of postcontrast FLAIR imaging. Therefore, it is hypothesized that adding postcontrast FLAIR images to an initial brain MRI in acute stroke patients will allow physicians to reach a clinical diagnosis and help in the decision making process regarding patient care.
In conclusion, patients with HARM without diffusion abnormalities were found in 2.4% of the patients with an acute stoke or TIA, using initial postcontrast FLAIR imaging after stroke. These lesions could be a specific type of TIA or stroke-mimicker, which causes BBB disruption with an unknown mechanism. These patients were older than and had similarly favorable short-term neurological outcomes compared with the patients with imaging-negative stroke.
Figures and Tables
![]() | Fig. 1Flow chart shows patients' selection process in this study.CE-FLAIR = contrast-enhanced FLAIR images, DWI = diffusion-weighted imaging, FLAIR = fluid attenuated inversion recovery, HARM = hyperintense acute reperfusion marker on contrast-enhanced FLAIR, ICH = intracerebral hemorrhage, SAH = subarachnoid hemorrhage, SDH = subdural hemorrhage
|
![]() | Fig. 275-year-old woman with global aphasia.Initial brain MRI shows linear contrast enhancement (arrows) on brain surface on postcontrast FLAIR imaging, which is early hyperintense acute reperfusion marker sign (A). There is no evidence of acute infarction on diffusion-weighted image (B), and no intracranial hemorrhage on gradient echo image (C). There is contrast present from previous images performed 7 hours prior in cerebrospinal fluid space on follow-up FLAIR image, which is feature of classic HARM (D-F). FLAIR = fluid attenuated inversion recovery
|
![]() | Fig. 387-year-old man with right lower extremity weakness.
A. Initial brain MRI shows focal linear contrast enhancement at both of inferior occipital sulci (arrows) on postcontrast FLAIR image, which is early hyperintense acute reperfusion marker sign. B. There is no evidence of acute infarction on diffusion-weighted image. C, D. Contrast is still present in cerebrospinal fluid space from previous images performed 23 hours prior on first follow-up FLAIR images, which is feature of classic HARM. E, F. Second follow-up MRI obtained 48 h after initial MRI shows no contrast present on FLAIR images. FLAIR = fluid attenuated inversion recovery
|
Table 1
Comparisons between Imaging-Negative Stroke Group and HARM without Diffusion Abnormalities Group Regarding Their Demographic and Clinical Characteristics
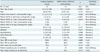
Table 2
Clinical and Radiological Characteristics of Patients with HARM without Diffusion Abnormalities
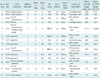
*One patient had two separate episodes of stroke-like symptoms and underwent MRI, which showed early HARM signs (7 and 8), †NIHSS at time of admission, at 1 day after event, and at 7 days after event, respectively, ‡Time interval between stroke-like episode and first MR imaging study (in hours). CSF = cerebrospinal fluid, FLAIR = fluid attenuated inversion recovery, h = hours, HARM = hyperintense acute reperfusion marker, L = left, NIHSS = National Institutes of Health Stroke Scale, N/A = not applicable, R = right, WBC = white blood cell
Acknowledgments
We thank the Medical Research Collaborating Center at Seoul National University Bundang Hospital for statistical analyses.
References
1. Köhrmann M, Struffert T, Frenzel T, Schwab S, Doerfler A. The hyperintense acute reperfusion marker on fluid-attenuated inversion recovery magnetic resonance imaging is caused by gadolinium in the cerebrospinal fluid. Stroke. 2012; 43:259–261.
2. Bonzano L, Roccatagliata L, Levrero F, Mancardi GL, Sardanelli F. In vitro investigation of poor cerebrospinal fluid suppression on fluid-attenuated inversion recovery images in the presence of a gadolinium-based contrast agent. Magn Reson Med. 2008; 60:220–223.
3. Ewing JR, Knight RA, Nagaraja TN, Yee JS, Nagesh V, Whitton PA, et al. Patlak plots of Gd-DTPA MRI data yield blood-brain transfer constants concordant with those of 14C-sucrose in areas of blood-brain opening. Magn Reson Med. 2003; 50:283–292.
4. Warach S, Latour LL. Evidence of reperfusion injury, exacerbated by thrombolytic therapy, in human focal brain ischemia using a novel imaging marker of early blood-brain barrier disruption. Stroke. 2004; 35:11 Suppl 1. 2659–2661.
5. Dechambre SD, Duprez T, Grandin CB, Lecouvet FE, Peeters A, Cosnard G. High signal in cerebrospinal fluid mimicking subarachnoid haemorrhage on FLAIR following acute stroke and intravenous contrast medium. Neuroradiology. 2000; 42:608–611.
6. Latour LL, Kang DW, Ezzeddine MA, Chalela JA, Warach S. Early blood-brain barrier disruption in human focal brain ischemia. Ann Neurol. 2004; 56:468–477.
7. Henning EC, Latour LL, Warach S. Verification of enhancement of the CSF space, not parenchyma, in acute stroke patients with early blood-brain barrier disruption. J Cereb Blood Flow Metab. 2008; 28:882–886.
8. Rozanski M, Ebinger M, Schmidt WU, Hotter B, Pittl S, Heuschmann PU, et al. Hyperintense acute reperfusion marker on FLAIR is not associated with early haemorrhagic transformation in the elderly. Eur Radiol. 2010; 20:2990–2996.
9. Ogami R, Nakahara T, Hamasaki O, Araki H, Kurisu K. Cerebrospinal fluid enhancement on fluid attenuated inversion recovery images after carotid artery stenting with neuroprotective balloon occlusions: hemodynamic instability and blood-brain barrier disruption. Cardiovasc Intervent Radiol. 2011; 34:936–941.
10. Barr TL, Latour LL, Lee KY, Schaewe TJ, Luby M, Chang GS, et al. Blood-brain barrier disruption in humans is independently associated with increased matrix metalloproteinase-9. Stroke. 2010; 41:e123–e128.
11. Merino JG, Latour LL, Tso A, Lee KY, Kang DW, Davis LA, et al. Blood-brain barrier disruption after cardiac surgery. AJNR Am J Neuroradiol. 2013; 34:518–523.
12. Kim DW, Moon Y, Gee Noh H, Choi JW, Oh J. Blood-brain barrier disruption is involved in seizure and hemianopsia in nonketotic hyperglycemia. Neurologist. 2011; 17:164–166.
13. Kidwell CS, Latour L, Saver JL, Alger JR, Starkman S, Duckwiler G, et al. Thrombolytic toxicity: blood brain barrier disruption in human ischemic stroke. Cerebrovasc Dis. 2008; 25:338–343.
14. Farrall AJ, Wardlaw JM. Blood-brain barrier: ageing and microvascular disease--systematic review and meta-analysis. Neurobiol Aging. 2009; 30:337–352.