Abstract
Objective
To investigate the accuracy, efficiency and radiation dose of a novel laser navigation system (LNS) compared to those of free-handed punctures on computed tomography (CT).
Materials and Methods
Sixty punctures were performed using a phantom body to compare accuracy, timely effort, and radiation dose of the conventional free-handed procedure to those of the LNS-guided method. An additional 20 LNS-guided interventions were performed on another phantom to confirm accuracy. Ten patients subsequently underwent LNS-guided punctures.
Results
The phantom 1-LNS group showed a target point accuracy of 4.0 ± 2.7 mm (freehand, 6.3 ± 3.6 mm; p = 0.008), entrance point accuracy of 0.8 ± 0.6 mm (freehand, 6.1 ± 4.7 mm), needle angulation accuracy of 1.3 ± 0.9° (freehand, 3.4 ± 3.1°; p < 0.001), intervention time of 7.03 ± 5.18 minutes (freehand, 8.38 ± 4.09 minutes; p = 0.006), and 4.2 ± 3.6 CT images (freehand, 7.9 ± 5.1; p < 0.001). These results show significant improvement in 60 punctures compared to freehand. The phantom 2-LNS group showed a target point accuracy of 3.6 ± 2.5 mm, entrance point accuracy of 1.4 ± 2.0 mm, needle angulation accuracy of 1.0 ± 1.2°, intervention time of 1.44 ± 0.22 minutes, and 3.4 ± 1.7 CT images. The LNS group achieved target point accuracy of 5.0 ± 1.2 mm, entrance point accuracy of 2.0 ± 1.5 mm, needle angulation accuracy of 1.5 ± 0.3°, intervention time of 12.08 ± 3.07 minutes, and used 5.7 ± 1.6 CT-images for the first experience with patients.
Numerous devices to facilitate computed tomography (CT)-guided interventions have been developed recently (1234567) to allow for optimal needle placement at an exact angle (68). Compared to other imaging methods for punctures, such as magnetic resonance imaging (MRI) or ultrasound, CT offers fast image acquisition, widespread availability, and high resolution accurate image quality. CT-guided punctures can be facilitated using CT-fluoroscopy, robotic devices, mounted systems, hand-held devices, systems that require attachment to the patient's skin, or laser devices (1234567).
The intervention site can be determined reliably and precisely using a laser navigation system (LNS), which increases accuracy of the intervention, as since both the puncture point and puncture angle of the needle are exactly shown on the patient's skin surface according to CT-planning. Improved accuracy using LNS has been reported previously in a randomised study addressing spine interventions. The authors reported significant improvement during planning of the puncture point and needle angulation using a LNS compared to those of a freehand technique (1).
This study was conducted to compare a novel LNS for CT-guided punctures with a freehand approach, which is currently the standard procedure at our institution. Accuracy of the different puncture methods was the primary focus of this study, as precise needle placement based on pre-interventional planning is crucial (5). Accuracy of the puncture is influenced by the speed of the intervention and by the number of images taken during the procedure; thus, these factors were also considered. The overall goal of this study was to determine whether LNS is advantageous in terms of overall interventional time and accuracy for CT-guided punctures compared to those of the freehand puncture method.
This study was conducted under approval by the Institutional Review Board.
A third-generation 64-slice CT scanner (Somatom Definition AS, Siemens Healthcare, Erlangen, Germany) was used for this study. The CT-guided LNS device (Amedo STS GmbH, Bochum, Germany) consisted of a movable, rotating laser unit on a 220° circular rail surrounding the patient table in front of the CT gantry, an image processing unit to position the laser, and navigation software for interventional planning. The laser system was fixed to a carrying arm, which is attached to the CT room ceiling (Fig. 1). The LNS included the following components: 1) 220° gantry arc with motorised laser positioning unit, 2) support arm for mounting the gantry arc, and 3) a computer with operating terminal and planning software.
The physician generated the CT planning image, which was transferred to the planning software. Planning was performed by drawing a line on the CT image. The motorised laser unit navigated exactly to the correct position as determined by planning and showed the puncture site for the laser beam. The interventionist aligned the instrument with the laser beam to direct the beam axially to the instrument, which ensured a perfect puncture angle. Intervention depth was defined during planning and was accurately adjusted using needles or a depth stop.
All interventions were planned and performed in the axial plane, as the laser only allowed for translatory and rotatory movement; no double oblique needle interventions were possible with the current system.
The punctures were first made with two different phantoms using a standard puncture needle (SOMATEX Medical Technologies GmbH, Teltow, Germany, 1.5 × 150 mm, 17 gauge). After the phantom studies were completed, the first 10 patients were punctured using the LNS.
The first phantom ("Phantom 1") was the common torso Sawbones model (Siemens Healthcare) in the shape of a human trunk, which consisted of rubber-coated stiff foam and was prepared by placing several peripheral venous catheter needles as targets on the inside beneath its removable spine.
Phantom 1 was utilised for 60 punctures, divided into an equal number of interventions using the standard freehand method (n = 30) and the LNS-guided method (n = 30). Due to the stiffness of the body phantom we used the second soft sponge phantom ("Phantom 2") to evaluate the influence of the body phantom on accuracy. All phantom and patient interventions were performed by a radiologist with more than 10 years of experience with CT interventions.
Phantom 2 was prepared from commercially available sponge material (12 units, total dimensions: 28 cm length × 16 cm width × 18 cm height) and 10 crossways plastic targets (length, 1.7 cm; thickness, 2 mm; diameter of the centre, 2 mm) randomly positioned between the sponges. Phantom 2 was used for 20 punctures, and all were performed using the LNS to re-evaluate accuracy without interference. In addition, we evaluated the initial experience with LNS for CT-guided puncture of patients (n = 10).
The LNS was set-up before the intervention by automatically lowering the arc into its operating position (Fig. 1) and verifying its accuracy via two reference markers on the floor. The two reference markers on the floor were located in a pre-defined, saved location in the system. The calibration process entailed the software, so the laser could point and move to the points on the floor. When the laser beam exactly coincided with the point on the floor, the laser system was used or adjustments were made before using the system. This calibration check procedure was performed once per day to ensure system reliability. Thereafter, the LNS was used without any further calibration. The entire set-up procedure including the daily quality check took about 2 minutes.
A complete CT scan of Phantom 1 was acquired on the CT table with a slice thickness of 5 mm, and the image file (DICOM format) was transferred via a local network to the LNS workstation. The appropriate image plane and needle path for the puncture was selected by drawing a marker line selecting the needle entry point on the surface (representing the skin) of the phantom body and the target point using the LNS navigation software. The system automatically calculated puncture depth and table position. Once the corresponding table position was reached, the LNS automatically marked the needle entry point (Fig. 2A) and needle direction via the laser beam. The physician introduced the needle at the highlighted needle entry point and advanced the needle, ensuring that the laser spot was always visible on the distal end of the needle (Fig. 2B). The correct length of the intervention for needles without depth control was estimated from the residual part of the needle. Once the needle tip reached the target point, needle advancement was stopped, and the intervention was completed. As soon as the series of punctures were finished, another CT-scan of the whole phantom was performed, and the data were transferred to the LNS computer for the accuracy analysis (Fig. 3). The procedure was the same for Phantom 2, and the intervention was terminated when the needle reached the target. The first patients were punctured using the same technique.
Only Phantom 1 was used. The phantom was prepared and placed on the CT table in the same fashion as for the LNS-guided intervention. These image data were used to plan the needle path on the standard CT workstation.
First, the phantom was moved into the gantry, and the CT laser grid illuminated the targeted image plane. A customised CT reference marker made of paper clips was placed on the surface of the phantom at the estimated needle entry point position. The marker position was checked using single shot CT-images and adjusted until the planned needle entry point was accurately detected and marked with a pen. The physician introduced the needle and advanced it to the estimated target point. The position of the needle during the intervention was verified continuously by acquiring single slice images. The intervention was terminated by the physician once the needle tip was as close as possible to the target point.
Accuracy measurements for technique 1 were preformed with JiveX software (Visus Technology Transfer GmbH, Bochum, Germany) and those for technique 2 were preformed on a standard workstation (Siemens) using standard digital measurement in-plane.
Accuracy was measured as the mean difference between the planned and achieved needle entrance points (mm), and needle target point accuracy was calculated as the mean difference between the planned and achieved needle tip positions of the target (mm) in the x and y directions; needle angulation accuracy (degree) was determined as the mean difference between the planned and final angles of the puncture trajectory.
The complete procedure time was subdivided into preparation time and skin-to-target time. Preparation time for the laser-guided technique was the mean time between needle path planning at the computer and laser projection of the needle entry point on the phantom. Skin-to-target time was the mean time between first penetration at the needle entry point and successfully reaching the target point.
Preparation time for the freehand method was the mean time between needle path planning at the CT and marking the needle entry point. Skin-to-target time was the mean time between first penetration at the needle entry point and reaching the target point successfully.
The number of CT slices for both techniques was documented to assess radiation exposure. A statistical analysis was conducted for the 60 Phantom 1 punctures. After applying the Shapiro-Wilk test to check normality, the data were compared using either the paired t test (normal distribution) or the Mann-Whitney U-test (non-normal distribution). A p value ≤ 0.1 was considered significant for the normality test. A p value < 0.05 was considered significant for the paired t test and the Mann-Whitney U-test. All analyses were conducted with SAS statistical software (SAS 9.2 for Windows, SAS Institute, Cary, NC, USA).
All LNS-supported interventions (n = 30) for the Phantom 1, Phantom 2 (n = 20) and the patients (n = 10) were performed without technical problems or complications. The results of the punctures are summarised in Table 1.
The needle path of the punctures conducted on Phantom 1 had a mean length of 94.2 ± 16.9 mm (± standard deviation) for the LNS-guided punctures and 93.0 ± 17.9 mm for the freehand punctures.
The LNS-guided punctures executed on the Phantom 1 had a mean difference of 1.3 ± 0.9° from the planned needle path, whereas the deviation for the freehand interventions from the planned needle path was 3.4 ± 3.1° (p = 0.006). Needle target point accuracy was 4.0 ± 2.7 mm, and needle entrance point accuracy was 0.8 ± 1.0 mm for the LNS-guided interventions, whereas the conventional punctures had a distance from the target of 6.3 ± 3.6 mm (p = 0.008) and needle entrance point accuracy of 6.1 ± 4.7 mm (p < 0.001).
A mean number of 4.2 ± 3.6 images were taken during the LNS-guided punctures compared to 7.9 ± 5.1 images acquired during the freehand punctures (p < 0.001). The interventions using the LNS required 0.43 ± 0.5 minutes for the preparation phase and 6.21 ± 5.17 minutes for the skin-to-target time. These durations resulted in a mean of 7.03 ± 5.18 minutes for the entire intervention. The preparation time for the freehand punctures was 1.44 ± 0.18 minutes (p < 0.01), and the skin-to-target time was 6.57 ± 4.15 minutes (p = 0.32). The cumulative duration was 8.38 ± 4.09 minutes (p = 0.027).
The mean needle path length was 73.2 ± 19.7 mm, and mean needle angulation accuracy was 1.0 ± 1.2°.
Needle entrance point accuracy was 1.4 ± 2.0 mm, and the distance from the planned target was 3.6 ± 2.5 mm. The punctures required a mean of 0.42 ± 0.05 minutes for preparation and 1.02 ± 0.22 minutes for the skin-to-target time. The complete procedure took a mean time of 1.44 ± 0.22 minutes.
Ten patients underwent LNS-guided punctures (Fig. 4). Table 2 summarises the interventional procedures performed in the patient group. Mean target access path within the patients in the LNS group was 61.3 ± 11.3 mm. Target point accuracy in the patient group was 5.0 ± 1.2 mm, and needle entrance point accuracy was 2.0 ± 1.5 mm. Needle angulation accuracy was 1.5 ± 0.3°.
A mean of 5.7 ± 1.6 images was acquired during the LNS punctures. The LNS interventions required a mean of 5.14 ± 1.13 minutes for preparation and 6.54 ± 2.04 minutes for the skin-to-target time. These interventions took a mean total time of 12.08 ± 3.07 minutes.
The learning curve for the LNS-guided interventions on Phantom 1 showed mean procedure duration of 11.55 ± 6.31 minutes on day 1 and 2.57 ± 0.42 minutes on the last day. The mean number of images acquired during the intervention was 1.5 ± 0.8 on the last day and 8 ± 3.5 on the first day.
The various modalities for guiding percutaneous interventions include ultrasound, fluoroscopy, MRI, and CT. Ultrasound-guided punctures can be performed with real time needle tracking and a view of the targeted tissue, which allows needle position to be adjusted immediately if necessary (e.g., due to respiratory movements). However, this method is limited to well-visualised regions of the body and may be insufficient for complex punctures in obese patients, those with special anatomic conditions, and in the absence of dilation of the renal collecting system (9). MRI-guided puncture offers an excellent imaging technique and free choice of the image plane (810) but suffers from disadvantages concerning accessibility to the patient, the device material used for the puncture (11) and restrictions to magnetic devices (e.g., implanted pacemakers). MR-compatible assistance systems compensate for poor patient access (1213).
While CT-guided puncture results in considerable exposure to radiation, it offers good image quality in complex targeted areas and is widely available. Different strategies have been developed to improve the accuracy of CT-guided interventions, including CT-fluoroscopy, robotic devices, electromagnetic targeting systems and laser guidance devices (1234567). As each modality has advantages and disadvantages, a particular modality for guidance during percutaneous intervention should be chosen for each case and may also be influenced by the physician's preference and experience. However, most of these systems often require additional and time consuming registration and manual adjustments to determine the needle entrance point. CT-guided puncture using fluoroscopic guidance provides high accuracy at the cost of increased radiation exposure (11415).
The main advantage of any laser guidance system is haptic control of the intervention needles and contact-free guidance. The needle can be moved freely, as the laser source can be relatively far away from the patient's skin. Laser guidance systems can be hand-held (7), mounted on a CT gantry (16) or fixed elsewhere in the CT room (2). In our study, the system was permanently attached to the CT-room ceiling on a motorised traversable arm and could be removed when necessary. This situation is even more distinct for laser devices, particularly when performing three-dimensional-reconstruction of the area of interest using fluoroscopic needle control (9). However, different robotic devices have been developed for image-guided punctures to minimise the loss of accuracy caused by human factors while preventing the physician and patient from receiving unnecessary radiation exposure (5). Static robotic devices have the disadvantage of restricting the needle path to the planned trajectory; therefore, they prevent the physician from spontaneous adjustments in the case of an altered target position (e.g., breathing or movement). As most devices do not provide constant information on needle position, several electromagnetic devices have been developed recently to facilitate CT-guided puncture (171819). These devices increase accuracy and lower radiation exposure during CT-guided puncture without restricting patient accessibility.
Most laser-guided systems for CT have the advantage of not obstructing the puncture site as much as other devices (e.g., robotic devices) and are in direct contact with the needle (2). Subsequently, the needle path can be altered according to body movement, which is important for thoracic and abdominal targets. Laser needle guidance mechanisms as described by Magnusson et al. (20) do not determine skin entry points, and manual registration can be time-consuming. Hand-held laser devices require sterilisation after each use and gantry-mounted systems reduce access to the patient because of their limited working area (4), but LNS-guided interventions can be executed with the puncture site at a certain distance from the gantry and from the laser beam source without the need to sterilise any of the components. This distance increases patient accessibility because the physician can move the needle in a wider area without touching either the gantry or the device. Mounting the device on the ceiling requires some installation costs and the system cannot be moved to another room or scanner. However, gantry-mounted devices face the same disadvantages, and this positioning allows the correct position to be confirmed easily by targeting some fixed spots on the floor of the room. Furthermore, such a design may be useful for MR-guided interventions.
Our results indicate an advantage when a LNS is employed for CT-guided puncture comparison to that of freehand puncture.
All values measured except skin-to-target time were significantly more precise with shorter treatment duration and fewer images acquired during the puncture. Thus, radiation exposure was reduced compared to that of a conventional guidance system.
Our results were confirmed in the first clinical patient application of the LNS.
The LNS enables the physician to adjust needle position to any target position while using the laser as a precise guidance device to show the originally intended needle path compared to those of other devices for CT-guided puncture. However, double oblique punctures are a challenge for every radiologist. A new device is currently in development to improve applicability, which will provide double oblique trajectories for routine clinical practice.
In conclusion, our results indicate that the LNS improves accuracy, speed and safety of CT-guided interventions. The needle can be placed more accurately and quickly while requiring fewer images, thereby reducing radiation exposure during the procedure.
Figures and Tables
Fig. 1
Laser navigation system (LNS) is fixed to carrying arm attached to computed tomography (CT) room ceiling.
C-arm of LNS is positioned in front of gantry (arrow). System does not impair regular use of CT room.
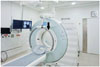
Fig. 2
Marking needle entry point with laser navigation system (LNS).
A. Image illustrates placing needle tip on surface of Phantom 2 with laser spot marked by LNS during intervention. B. Needle is adjusted in direction of laser beam denoted by projecting laser beam as point on centre of upper end of puncture needle during lesion targeting in Phantom 1.
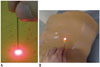
Fig. 3
Phantom 1 computed tomography (CT) image.
Axial CT image of Phantom 1 using laser navigation system showing needle reaching its target position (arrow).
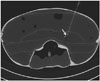
Fig. 4
Computed tomography image of patient with pleural effusion left.
Confirmation of needle position in comparison to planned needle path for intervention using laser navigation system in patient with pleural effusion left.
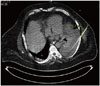
Table 1
Results of Punctures Performed with Phantom 1, 2 and in Patients
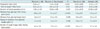
References
1. Moser C, Becker J, Deli M, Busch M, Boehme M, Groenemeyer DH. A novel Laser Navigation System reduces radiation exposure and improves accuracy and workflow of CT-guided spinal interventions: a prospective, randomized, controlled, clinical trial in comparison to conventional freehand puncture. Eur J Radiol. 2013; 82:627–632.
2. Nitta N, Takahashi M, Tanaka T, Takazakura R, Sakashita Y, Furukawa A, et al. Laser-guided computed tomography puncture system: simulation experiments using artificial phantom lesions and preliminary clinical experience. Radiat Med. 2007; 25:187–193.
3. Yang CL, Yang BD, Lin ML, Wang YH, Wang JL. A patient-mount navigated intervention system for spinal diseases and its clinical trial on percutaneous pulsed radiofrequency stimulation of dorsal root ganglion. Spine (Phila Pa 1976). 2010; 35:E1126–EE113.
4. Krombach GA, Schmitz-Rode T, Wein BB, Meyer J, Wildberger JE, Brabant K, et al. Potential of a new laser target system for percutaneous CT-guided nerve blocks: technical note. Neuroradiology. 2000; 42:838–841.
5. Tovar-Arriaga S, Tita R, Pedraza-Ortega JC, Gorrostieta E, Kalender WA. Development of a robotic FD-CT-guided navigation system for needle placement-preliminary accuracy tests. Int J Med Robot. 2011; 7:225–236.
6. Kloeppel R, Weisse T, Deckert F, Wilke W, Pecher S. CT-guided intervention using a patient laser marker system. Eur Radiol. 2000; 10:1010–1014.
7. Palestrant AM. Comprehensive approach to CT-guided procedures with a hand-held guidance device. Radiology. 1990; 174:270–272.
8. Bale R, Widmann G. Navigated CT-guided interventions. Minim Invasive Ther Allied Technol. 2007; 16:196–204.
9. Ritter M, Rassweiler MC, Häcker A, Michel MS. Laser-guided percutaneous kidney access with the Uro Dyna-CT: first experience of three-dimensional puncture planning with an ex vivo model. World J Urol. 2013; 31:1147–1151.
10. Zangos S, Müller C, Mayer F, Naguib NN, Nour-Eldin NE, Hansmann ML, et al. [Retrospective 5-year analysis of MR-guided biopsies in a low-field MR system]. Rofo. 2009; 181:658–663.
11. Schell B, Eichler K, Mack MG, Müller C, Kerl JM, Czerny C, et al. [Robot-assisted biopsies in a high-field MRI system - first clinical results]. Rofo. 2012; 184:42–47.
12. Moche M, Zajonz D, Kahn T, Busse H. MRI-guided procedures in various regions of the body using a robotic assistance system in a closed-bore scanner: preliminary clinical experience and limitations. J Magn Reson Imaging. 2010; 31:964–974.
13. Zangos S, Melzer A, Eichler K, Sadighi C, Thalhammer A, Bodelle B, et al. MR-compatible assistance system for biopsy in a high-field-strength system: initial results in patients with suspicious prostate lesions. Radiology. 2011; 259:903–910.
14. Becker HC, Meissner O, Waggershauser T. [C-arm CT-guided 3D navigation of percutaneous interventions]. Radiologe. 2009; 49:852–855.
15. Proschek D, Kafchitsas K, Rauschmann MA, Kurth AA, Vogl TJ, Geiger F. Reduction of radiation dose during facet joint injection using the new image guidance system SabreSource: a prospective study in 60 patients. Eur Spine J. 2009; 18:546–553.
16. Jacobi V, Thalhammer A, Kirchner J. Value of a laser guidance system for CT interventions: a phantom study. Eur Radiol. 1999; 9:137–140.
17. Penzkofer T, Bruners P, Isfort P, Schoth F, Günther RW, Schmitz-Rode T, et al. Free-hand CT-based electromagnetically guided interventions: accuracy, efficiency and dose usage. Minim Invasive Ther Allied Technol. 2011; 20:226–233.
18. Appelbaum L, Sosna J, Nissenbaum Y, Benshtein A, Goldberg SN. Electromagnetic navigation system for CT-guided biopsy of small lesions. AJR Am J Roentgenol. 2011; 196:1194–1200.
19. Penzkofer T, Isfort P, Bruners P, Wiemann C, Kyriakou Y, Kalender WA, et al. Robot arm based flat panel CT-guided electromagnetic tracked spine interventions: phantom and animal model experiments. Eur Radiol. 2010; 20:2656–2662.
20. Magnusson A, Radecka E, Lönnemark M, Raland H. Computed-tomography-guided punctures using a new guidance device. Acta Radiol. 2005; 46:505–509.