Abstract
Figures and Tables
![]() | Fig. 1Different delayed enhancement patterns in non-ischemic cardiomyopathy.Patient 1. 48-year-old female with history of dilated cardiomyopathy. Delayed enhancement images demonstrate intramural contrast enhancement in septum (midwall striae, arrowheads). A. Mid-ventricular short-axis view. B. 4-chamber-view. Patient 2. 50-year-old male with remote history of biopsy-proven viral myocarditis presented with left ventricular dysfunction. Delayed enhancement images demonstrate epicardial hyperenhancement localized at basal inferolateral wall (arrowheads). C. Basal-short axis view. D. 3-chamber-view. Patient 3. 68-year-old male presented with progressive left ventricular dysfunction found to have insignificant coronary stenosis (25% middle left anterior descending artery lesion) on invasive coronary angiography. Delayed enhancement images demonstrate subendocardial hyperenhancement with 75% transmurality (arrowheads) involving inferior and inferolateral walls from middle ventricular level extending through apex consistent with ischemic injury. E. Mid-ventricular short axis view. F. 3-chamber-view.
|
![]() | Fig. 2Individual and pooled hazard ratios from univariate Cox proportional hazards analysis for risk of major cardiovascular events.Forest plot comparing prognosis of NICM patients with and without scar, detected by delayed-enhancement CMR. CI = confidence interval, MACE = major adverse cardiovascular event, NICM = non-ischemic cardiomyopathy
|
![]() | Fig. 3Individual and pooled hazard ratios from univariate Cox proportional hazards analysis for risk of all-cause mortality.Forest plot comparing prognosis of NICM patients with and without scar, detected by delayed-enhancement MRI. CI = confidence interval, NICM = non-ischemic cardiomyopathy, SE = standard error
|
![]() | Fig. 4Individual and pooled hazard ratios from univariate Cox proportional hazards analysis for risk of arrhythmic events.Forest plot comparing prognosis of NICM patients with and without scar, detected by delayed-enhancement MRI. CI = confidence interval, NICM = non-ischemic cardiomyopathy, SE = standard error
|
Table 1
Studies Evaluating Relation of Myocardial Scar and Outcomes in Non-Ischemic Heart Disease and Cardiomyopathy
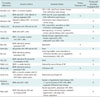
First Author (Reference) | Inclusion Criteria | Exclusion Criteria | Primary Prophylaxis ICD | Primary and Secondary Prophylaxis ICD* |
---|---|---|---|---|
Assomull (19) | DCM > 12 months duration | LVEF > 56%, significant valvular disease, HCM, infiltrative heart disease | O | |
Wu (27) | NICM and LVEF ≤ 35% referred to primary prophylaxis ICD | NYHA class IV, acute myocarditis, CHD, HCM, infiltrative heart disease | O | |
Hombach (28) | Idiopathic dilated CMP > 12 months duration | Inflammatory heart disease based on cardiac biopsy | O | |
Cheong (42) | All patients with CMR and no CAD | HCM, myocarditis, sarcoidosis or other infiltrative cardiomyopathy | O | |
Cho (32) | NICM with LVEF < 35% | Life expectancy < 6 months, significant primary valvular disease, prior myocarditis | O | |
Lehrke (29) | DCM > 12 months duration with LVEF < 50% | Valvular disease, hypertensive heart disease, myocarditis, CHD, endocardial scar, LVEF > 55% | O | |
Iles (46) | NICM referred to primary prophylaxis ICD | Myocarditis | O | |
Klem (43) | All patients with CMR and no CAD | None | O | |
Wu (44) | NICM referred to primary prophylaxis ICD | Acute myocarditis, acute sarcoidosis or infiltrative disorders such as amyloidosis, hemochromatosis, CHD, HCM | O | |
Gao (34) | NICM referred to EP service for consideration of ICD | None | O | |
Müller (41) | NICM < 4 weeks duration | Endocardial scar or history of MI | O | |
Neilan (30) | NICM referred to primary prophylaxis ICD | Infiltrative CMP | O | |
Gulati (23) | DCM > 6 months duration with LV end diastolic volume above and LVEF below normal values | Endocardial scar of prior MI | O | |
Perazzolo Marra (31) | NICM and LVEF < 50% | Valvular or hypertensive heart disease, CHD, < 1 month duration of CHF, HCM, ARVC, suspected infiltrative heart disease | O | |
Masci (35) | NICM with reduced LVEF and no history of CHF | Recent myocarditis, peripartum CMP, ARVC, severe primary valvular disease, untreated hypertension, end-stage HCM, cardiac amyloidosis | O |
"O" indicates included patient. In four of studies, only patients with NICM referred to ICD implantation for primary prophylaxis of SCD were included. *Patients with prior sudden cardiac arrest, ventricular tachycardia or other indications for secondary ICD prophylaxis were included, or not specified. ARVC = arrhythmogenic right ventricular cardiomyopathy, CAD = coronary artery disease, CHD = congenital heart disease, CHF = congestive heart failure, CMP = cardiomyopathy, CMR = cardiac magnetic resonance, DCM = non-ischemic dilated cardiomyopathy, HCM = hypertrophic cardiomyopathy, ICD = implantable cardioverter-defibrillator, ICM = ischemic cardiomyopathy, LVEF = left ventricular ejection fraction, MI = myocardial infarction, NICM = non-ischemic cardiomyopathy, NYHA = New York Heart Association
Table 2
Study Characteristics and Results
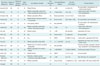
First Author (Reference) | Number of Patients | Follow-up Duration (Months)* | Mean LVEF (%) | Scar Patterns Included | Scar Prevalence (%) | Scar Quantification Method† | Scar Size (% LV Mass)* | Primary Endpoint |
---|---|---|---|---|---|---|---|---|
Assomull (19) | 101 | 22 | 35 | Midwall fibrosis | 35 | > 2SD | 4.6 (0.8-21) | All cause death, hospitalization for cardiovascular disease |
Wu (27) | 65 | 17 | 24 | Midwall, endocardial, patchy foci often including RV insertion sites | 42 | > Peak remote | 10 (3-16) | Cardiovascular death, ICD shock, CHF hospitalization |
Hombach (28) | 141 | 45 | 32 | Midwall, epicardial, diffuse | 26 | Manual planimetry | 5.6 (1.2-25.6) | Cardiac death |
Cheong (42) | 215 | 53 | 52 | Midwall fibrosis and other patterns not specified | 18 | Visual scoring | [Scar index: 1.00] | All cause death, heart transplant |
Cho (32) | 79 | 33 | 33 | Focal patchy, diffuse | 53 | Not quantified | - | All cause death, CHF hospitalization, transplant |
Lehrke (29) | 184 | 22 | 38 | Midwall, epicardial, patchy/foci, diffuse; endocardial excluded | 39 | > 2SD | 2.9 | Cardiac death, ICD shock, CHF hospitalization |
Iles (46) | 61 | 19 | 25 | Midwall, patchy | 51 | > 2SD | Not reported | Appropriate ICD shock |
Klem (43) | 554 | 29 | 56 | Any | 26 | Visual scoring | [4 segments] | All cause death |
Wu (44) | 98 | 43 | 25 | Any | 41 | FWHM | [0 (IQR 0-5.4) g] | Cardiac death, appropriate ICD shock |
Gao (34) | 65 | 21 | 27 | Midwall, epicardial, endocardial | 71 | > 2, 3, and 5SD‡ | [34.5 g] | Appropriate ICD shock, SCD, survived cardiac arrest |
Müller (41) | 185 | 21 | 43 | Midwall and non-midwall, excluding endocardial | 51 | Not quantified | - | All cause mortality, transplant, aborted SCD, sustained VT, CHF hospitalization |
Neilan (30) | 162 | 29 | 28 | Midwall, epicardial, RV insertion, diffuse | 50 | > 2SD/FWHM§ | 9 (2SD)/6 (FWHM) | Cardiovascular death, ICD shock |
Gulati (23) | 472 | 64 | 37 | Midwall fibrosis | 30 | FWHM | 2.5 (1.2-4.8) | All-cause death |
Perazzolo Marra (31) | 137 | 36 | 32 | Epicardial, midwall, patchy/junctional; endocardial excluded | 56 | > 2SD | 9 (4-15) | SCD, ICD shock, VF, sustained VT |
Masci (35) | 228 | 23 | 43 | Midwall, epicardial | 27 | Visual scoring∥ | [3 segments (1-10)] | Cardiac death, CHF onset, aborted SCD |
Averaged | 183 | 32 | 35 | 41 | 6.2 |
*Mean or median, range as reported in study, if other measure of scar placed in parenthesis and not included in summary analysis, †Presence or absence of scar was determined by visual interpretation of experienced readers first in all studies, ‡Various thresholds were tested, optimal threshold were > 2SD in ischemic and > 5SD in non-ischemic subgroups, §Both methods used, showing similar and robust results with respect to prognostic association, ∥Visual scoring only of segments when hyperintense myocardium involved > 50% of its circumferential extent. CHF = congestive heart failure, FWHM = full width at half maximum, ICD = implantable cardioverter-defibrillator, IQR = interquartile range, LV = left ventricle, LVEF = left ventricular ejection fraction, RV = right ventricle, SCD = sudden cardiac death, SD = standard deviation, VF = ventricular fibrillation, VT = ventricular tachycardia