Abstract
Objective
To evaluate engraftment by visualizing the location of human bone marrow-derived mesenchymal stem cells (hBM-MSCs) three-dimensionally in photothrombotic cerebral infarction (PTCI) models of rats.
Materials and Methods
Magnetic resonance imaging (MRI) of an agarose block containing superparamagnetic iron oxide (SPIO)-labeled hBM-MSCs was performed using a 3.0-T MRI, T2-(T2WI), T2*-(T2*WI), and susceptibility-weighted images (SWI). PTCI was induced in 6 rats, and 2.5 × 105 SPIO-labeled hBM-MSCs were infused through the ipsilateral internal carotid artery (ICA group) or tail vein (IV group). MRI was performed on days 1, 3, 7, and 14 after stem cell injection. Dark signal regions were confirmed using histology. Three-dimensional MRI reconstruction was performed using the clinical workflow solution to evaluate the engraftment of hBM-MSCs. Volumetric analysis of the engraftment was also performed.
Results
The volumes of SPIO-labeled hBM-MSCs in the phantom MRI were 129.3, 68.4, and 25.9 µL using SWI, T2*WI, and T2WI, respectively. SPIO-labeled hBM-MSCs appeared on day 1 after injection, encircling the cerebral infarction from the ventral side. Dark signal regions matched iron positive cells and human origin (positive) cells. The volume of the engraftment was larger in the ICA group on days 1, 3, and 7, after stem cell injection (p < 0.05 on SWI). SWI was the most sensitive MRI pulse sequence (p < 0.05). The volume of infarction decreased until day 14.
Magnetic resonance imaging (MRI) is one of the best molecular imaging tools and has the advantage of providing high-resolution anatomical information. Superparamagnetic iron oxide (SPIO) nanoparticles have been used as a T2 contrast agent for cell tracking (1, 2, 3). SPIO-labeled stem cells appear as a dark signal region on MRI with T2-weighted images (T2WI), T2*-weighted images (T2*WI), and recently with susceptibility-weighted images (SWI) (4, 5, 6, 7, 8).
Several studies have been performed using MRI with SPIO nanoparticles to evaluate the effectiveness of stem cell transplantation using animal brain stroke models (7, 8, 9, 10). Some studies have compared the differences in engraftment using intravenous (IV) and internal carotid arterial (ICA) injections of stem cells in rats (7, 11, 12); however, results have been controversial.
Three-dimensional stereoscopic viewing of the location of stem cells relative to a lesion can improve our understanding of cell therapy after IV and ICA injections, especially regarding tracking, distribution, and homing. Three-dimensional imaging studies of mice and rat brains have been conducted using MRI and three-dimensional visualization software to determine normal anatomy and histology (13, 14). Recently, a three-dimensional study of stem cells position in relation to cerebral infarction in animals was performed (15).
The purpose of this study was to examine the feasibility of using three-dimensional MRI to visualize the location of SPIO-labeled mesenchymal stem cells (MSCs) in photothrombotic cerebral infarction (PTCI) models of rats, and to compare the engraftment differences between ICA and IV injection of stem cells.
This study was performed under the guidelines of the Institutional Animal Ethics Committee at Chung-Ang University, and approved by the Institutional Review Board.
Human bone marrow-derived mesenchymal stem cells (hBM-MSCs) (HMSC-bm 7500; ScienCell Research Laboratories, Carlsbad, CA, USA) were grown in Dulbecco's modified Eagle's medium containing 10% fetal bovine serum, 200 mM L-glutamine, and 1% gentamicin reagent (all Invitrogen reagents; Invitrogen, Carlsbad, CA, USA) at 37℃, 5% CO2 atmosphere. The culture medium was replaced every 3 days and the cells were sub-cultured when 80-90% confluency was reached. To identify the cells using MRI, hBM-MSCs were labeled with 50 µg Fe/mL SPIO nanoparticles (Feridex IV; Advanced Magnetics Inc., Cambridge, MA, USA) by using a transfection agent, 750 ng/mL poly-L-lysine hydrobromide (PLL; Sigma, St. Louis, MO, USA) (5). To determine whether the cells were effectively labeled with SPIO, Prussian blue (PB) staining for ferric (Fe3+) iron was performed. PB staining has been described in the latter part of text.
A phantom was made to test the feasibility of obtaining MR images of SPIO-labeled hBM-MSCs. About 4 mL of 2% (W/V) agarose solution (Invitrogen, Carlsbad, CA, USA) was prepared at 60℃ and transferred into a well of a 12-well plate with a 2.2-mm diameter U-shaped polyethylene tube in the center. After solidification at room temperature, the tube was removed and 3.0 × 103/mL SPIO-labeled hBM-MSCs in media were infused into the U-shaped tunnel until it was completely full. A volume of 200-µL, equivalent to 6.0 × 102 cells was infused. An additional 2 mL of 2% agarose solution was added to the top and allowed to solidify. The phantom MRI was performed using a 3.0-T MRI system (Archieva; Philips Healthcare, Best, the Netherlands), T2WI, T2*WI, and SWI with a wrist coil (SENSE wrist 4 channel; Philips Healthcare). The sequence parameters were as follows: T2WI (turbo spin echo; repetition time [TR], 1637.5 ms; echo time [TE], 80 ms; flip angle, 90°; field of view [FOV], 150 x 150 mm; slice thickness, 1 mm; matrix size, 420 × 383; resolution, 0.36 × 0.40 × 1.0 mm); T2*WI (gradient echo; TR, 525.1 ms; TE, 9.2 ms; flip angle, 30°; FOV, 150 × 150 mm; slice thickness, 1 mm; matrix size, 404 × 408; resolution, 0.37 × 0.37 × 1.0 mm); and SWI (TR, 32.8 ms; TE, 42.8 ms, flip angle, 10°; FOV, 150 × 150 mm; slice thickness, 1 mm; matrix size, 288 × 294; resolution, 0.52 × 0.51 × 1.0 mm). Three-dimensional reconstruction of phantom MR images was performed in the same way as with in vivo MR images.
Prior to the study, animals were maintained at 21-24℃ for 1 week in a room with a 12-hour light-dark cycle. They were fed a standard rat food and had access to tap water. Six male Sprague-Dawley rats (DaehanBiolink, Eumseong, Korea), weighing 250-280 g, were used. Two methods were used to anesthetize the rats. Inhalation anesthesia was performed using 4-5% isoflurane (Aerane Solution; Ilsung, Seoul, Korea) to induce anesthesia and 1.5-2% isoflurane for maintenance. Intramuscular injection of anesthesia was administered using a combination of 100 mg/kg of ketamine hydrochloride (Ketara; Yuhan, Seoul, Korea) and 10 mg/kg of xylazine hydrochloride (Rompun; Bayer Korea, Ansan, Korea).
The rats were anesthetized using the inhalation method. The head was fixed to a stereotactic system (Stoelting Co., Wood Dale, IL, USA) in the prone position. The skull was exposed by making a midline incision on scalp. Rose Bengal solution (Sigma Aldrich Co., St. Louis, MO, USA) was injected at a concentration of 20 mg/kg through the tail vein and cold light with a 5-mm aperture was immediately applied to the skull for 15 minutes, 2.5 mm right lateral to the midline and 2.5 mm posterior to the bregma. The cold light was generated from an illuminator (Fiber Lite MI 150; Dolan Jenner Co., Boxborough, MA, USA) using a wavelength of 400-670 nm. The color temperature was 3200 K (16). After photoillumination, the scalp was sutured and the animals were maintained at 37℃ using a heating pad until they awoke from anesthesia.
The rats were randomly divided into 2 groups: an ICA group (n = 3) and an IV group (n = 3). The rats were anesthetized using the intramuscular injection method, and SPIO-labeled hBM-MSCs were injected on day 3 after inducing PTCI. For the ICA group, the ipsilateral right carotid artery was exposed and the external carotid artery was ligated with 6-0 silk. Then, 2.5 × 105 of SPIO-labeled hBM-MSCs in 200-µL media was infused slowly over 90 seconds into the right ipsilateral ICA through the right common carotid artery. After applying a cotton swab at the puncture site of carotid artery, the skin was sutured. For the IV group, 2.5 × 105 of SPIO-labeled hBM-MSCs in 500-µL media was injected through the tail vein.
MR images of the rat brains were obtained 2 days after inducing PTCI (1 day before cell injection) and on days 1, 3, 7, and 14 after stem cell injection. The rats were anesthetized using the intramuscular injection method. A 3.0-T MRI system (Archieva; Philips Healthcare) using T2WI, T2*WI, and SWI pulse sequences with a wrist coil (SENSE wrist 4 channel; Philips Healthcare) was used to obtain the MR images. The sequence parameters were as follows: T2WI (turbo spin echo; TR, 4709 ms; TE, 80 ms; flip angle, 90°; FOV, 50 x 50 mm; slice thickness, 1 mm; matrix size, 200 × 200; resolution, 0.25 × 0.25 × 1.0 mm); T2*WI (gradient echo; TR, 707 ms; TE, 23 ms; flip angle, 18°; FOV, 50 × 50 mm; slice thickness, 1 mm; matrix size, 200 × 200; resolution, 0.25 × 0.25 × 1.0 mm); and SWI (TR, 35 ms; TE, 45 ms; flip angle, 10°; FOV, 250 × 250 mm; slice thickness, 0.5 mm; matrix size, 485 × 485; resolution, 0.52 × 0.52 × 0.5 mm). An experienced radiologist (one of the authors) evaluated the images for cerebral infarction, accompanying changes, and dark signal regions surrounding the cerebral infarction.
The rats were sacrificed on day 14 after stem cell injection by trans-cardiac perfusion using 4% paraformaldehyde solution. The rats were anesthetized using the intramuscular injection method, and a thoracoabdominal incision was made to expose the heart. A 16-G needle was inserted into the left ventricle to the ascending aorta and 250-280 mL (1 mL/g body weight) of cold saline solution containing 2 U/mL heparin sodium (Green Cross Co., Yongin, Korea) was infused using an infusion pump (Master Flex; Cole-Parmer, Barrington, IL, USA) at a rate of 16.5 mL/min. Through an incision in the right atrium, the blood was discharged freely and change in color of discharge from blood-colored to clear was observed. A 4% paraformaldehyde solution (pH 7.4) was infused in succession using the same amount and rate. The brain was harvested and cut into 2 sections in the coronal plane (the same as the MRI plane) at the center of the infarction area using a rat brain matrix (RBM-4000C; ASI Instruments, Warrin, MI, USA). The tissue slices were re-fixed in 4% paraformaldehyde solution for 24 hours before preparing the paraffin blocks.
In order to localize the SPIO-labeled hBM-MSCs, immunohistochemistry (IHC) with anti-human mitochondria antibody for hBM-MSCs and PB staining for SPIO were performed sequentially on the same slide. Tissue sections (5-µm thick) were prepared in the coronal plane of the brain (the same as the MRI plane) at the center of the infarction area. An anti-human mitochondria monoclonal antibody (MAB1273; Millipore, Billerica, MA, USA), 1:50, was placed on the sections and kept overnight at 4℃. Goat anti-mouse secondary antibody (Alexa Fluor 488; Invitrogen, Carlsbad, CA, USA), 1:100, was applied for 2 hours at room temperature. FITC labeled secondary antibodies was detected by green fluorescein isothiocyanate. Anti-human mitochondria monoclonal antibody positive cells were observed using an inverted fluorescence microscope (Olympus IX71; Tokyo, Japan), and photographed. The same slides were re-stained with Perls' reagent to identify the SPIO iron particles. The sections were incubated with a 1:1 mixture of 2% potassium ferrocyanide and 1% hydrochloric acid for 30 minutes at room temperature. The slides were counterstained using nuclear fast red. Prussian blue positive cells were examined using a light microscope (Olympus BX51; Tokyo, Japan) and photographed. The two types of positive cells were identified by overlapping two photographs using commercial software (Photoshop 7.0; Adobe Systems Incorporated, San Jose, CA, USA). The histologic findings were simultaneously correlated with the MRI findings, focusing on the dark signal regions surrounding the cerebral infarction.
Additional tissue sections were prepared in the coronal plane of the brain at the center of the infarction area, and stained with hematoxylin solution for 8 minutes and with eosin solution for 1 minute. The histologic findings were correlated with the MRI findings, focusing on the areas of cerebral infarction, encephalomalacia, vessels, and hemorrhage.
The PTCI and SPIO-labeled stem cell MR image findings have previously been reported (7, 8, 16). The PTCI was focal and well-demarcated with a high signal intensity area at the illumination site. SPIO-labeled stem cells engrafted the cerebral infarction and appeared as a dark signal region. The MR images (pre-stem cell vs. post-stem cell MR images; right vs. left hemispheres) and the histology (IHC, PB, and hematoxylin and eosin staining) results were compared and contrasted. The MR images were interpreted, focusing on the high signals of the infarction and the dark signals of the SPIO-labeled hBM-MSCs engrafting the infarction, and by searching for accompanying signs such as hemorrhage, encephalomalacia, and other confusing factors such as normal and angiogenic vessels.
For three-dimensional reconstruction of the MR images, all source data were sent to Philips' clinical workflow solution (Extended Brilliance Workspace V 4.5; Philips Healthcare, Best, the Netherlands). There were 20 images for T2WI and T2*WI, and 40 for the SWI sequence. The single sequence data from each rat was individually processed. First, the MRI data were accessed through the volume mode of the workflow solution. The infarction area and dark signal regions were selected by free hand using the tissue segmentation function on each image. The color yellow was assigned to the high signal areas of the infarction, and the color red to the dark signal regions from the SPIO (Fig. 1). Each segmented image was automatically processed using the tissue management function, and three-dimensional images were displayed using the volume-rendering mode. Subsequently, quantitative volumes (mm3) of the infarction and the SPIO were obtained using the calculate-volume tool. Using the three-dimensionally reconstructed image, the relationship between the location of the infarction and the SPIO-labeled hBM-MSCs was observed. In particular, the location differences of the stem cells' homing between the ICA and IV group were qualitatively observed.
Prussian blue staining of hBM-MSCs labeled with SPIO nanoparticles at 50 µg Fe/mL indicated that all cells were sufficiently labeled (Fig. 2). Iron labeling did not cause any abnormal changes in the morphology of the cells.
Superparamagnetic iron oxide-labeled hBM-MSCs were seen as a U-shaped column of dark signal intensity in the phantom on T2WI, T2*WI, and SWI. SPIO distinctly reduced the signal intensity of the cells in the phantom (Fig. 3). The column of dark signal intensity was largest and most prominent on SWI, followed by T2*WI, and then T2WI (Fig. 3A). On SWI, the U-shaped column was exaggerated in size (larger than 2.2 mm of the diameter of the polyethylene tube), indicating sensitivity to detection. On T2WI, the column was fairly weak and underestimated, indicating insensitivity to detection. T2*WI, detection of the SPIO-labeled hBM-MSCs was less sensitive than SWI; however, the internal architecture of the U-shaped cell column was more clearly observed. The phantom MR images were successfully visualized in three-dimensional view. In the three-dimensionally reconstructed images, the shape and distribution of the dark signal intensities were easily shown as a U-shaped column of SPIO-labeled hBM-MSCs (Fig. 3B, Supplementary Video 1A, B, C in the online-only Data Supplement). The volumes were 129.3, 68.4, and 25.9 µL by using SWI, T2*WI, and T2WI, respectively. The column was shown most prominently on SWI, followed by T2*WI. On T2WI, the clump of cells was barely displayed.
Focal and oval cerebral infarctions were successfully induced in the right parietal lobe in all 6 rats without any event of death. The signal intensities were higher than the surrounding normal parenchyma on all pulse sequence images, T2WI, T2*WI, and SWI (Fig. 4). Prior to pre-stem cell infusion, and on days 1, 3, 7, and 14 after stem cell infusion, the high signal area of infarction was reduced in size and intensity (Fig. 4). A dark signal region which was not seen on pre-stem cell infusion appeared adjacent to the infarction on days 1 to 14, indicating engraftment of the stem cells to the infarction. The dark signal region was most prominent on SWI followed by T2*WI; however, it was not prominent on T2WI in any of the 6 rats (Fig. 4). The dark signal regions adjacent to the infarction on MRI (day 14) corresponded to the histology findings in all 6 rats (Fig. 5). The observed low signal regions were verified as SPIO-labeled hBM-MSCs in all 6 rats using PB and anti-human mitochondria antibody staining. The PB staining showed iron positive cells exactly at the same location corresponding to the MR image's dark signal region, and IHC staining showed that iron positive cells corresponded to the human mitochondria positive cells (Fig. 6).
Three-dimensionally reconstructed MR images were well displayed as yellow-colored infarctions and red-colored SPIO-labeled hBM-MSCs in brain tissues (Fig. 7, Supplementary Video 2 in the online-only Data Supplement). SPIO-labeled hBM-MSCs appeared on day 1 after injection, encircling the cerebral infarction from the ventral side, and increased in volume until day 3 (Fig. 8A). From days 3 to 14, the volume of SPIO-labeled hBM-MSCs was sustained (Fig. 8A). In the ICA group, the volume of engraftment was more than in the IV group on the days 1, 3, and 7 after stem cell injection (p < 0.05 on SWI) (Fig. 8A). On T2WI and T2*WI, the volume of engrafted SPIO-labeled hBM-MSCs showed no differences between the ICA and IV groups between days 1 to 14. SWI was observed to be the most sensitive of the pulse sequences (p < 0.05) (Fig. 8A). In the engraftment pattern of the SPIO-labeled hBM-MSCs for example, the location of engraftment, showed no discrimination point between the ICA and IV groups.
The volume of the infarction decreased over time in all 6 rats from day 2 after inducing PTCI (1 day before cell injection) until day 14 after stem cell injection (Fig. 8B). There were no differences in the volume of the cerebral infarction between the groups or between the MRI pulse sequences (p > 0.05).
In this three-dimensional MR image reconstruction study, longitudinal analyses made possible visualization of positional relationship between SPIO-labeled hBM-MSCs and the cerebral infarction, and to accurately size the engrafted stem cells and the cerebral infarction volume in 6 rats (Figs. 7, 8). The engraftment appeared while encircling the cerebral infarction from the ventral side from days 1 to 14. The cerebral infarction volumes decreased until day 14, while the engrafted cells increased from day 1 to early on day 3, and then sustained until day 14. There has been only one previous report on this topic. Daadi et al. (15) reported that three-dimensional reconstruction of the grafts and stroke allows an accurate representation of both the graft and stroke sizes and permits visualization of the relationship between small and large grafts to the stroke region.
There was more engraftment using the ICA injection than the IV injection initially from day 1 until day 7 with SWI (Figs. 4, 7, 8). The efficiency of the administration route is an important topic in stem cell therapy. Various MSC administration routes, including intracerebral, intra-arterial (IA), and IV injection, have been studied (7, 11, 12, 17, 18, 19). IV injection is non-invasive as compared to IA injection and relatively easy to administer. However, some studies have found unfavorable migration and distribution of injected cells after IV administration (20, 21, 22). These results were assumed to be caused by the first passage effect, which refers to the phenomenon in which the majority of administered MSCs are initially trapped in the pulmonary capillaries and removed from circulation (23, 24). IA administered cells result in a higher local concentration because of fewer mechanical stress factors (absence of the first passage effect) before cells reach the target site (21). A previous study also reported that stem cells injected by the IA route engrafted to the cerebrum significantly higher than IV administration in the early stage (under 48 hours) (7).
Among MRI pulse sequences, SWI was the most sensitive in detecting SPIO-labeled cells (Figs. 3, 4, 7, 8). In molecular imaging, detection ability is of utmost importance because the target cells and molecules are scarce. MRI is less sensitive than other techniques such as optical imaging and nuclear medicine. A few studies have similarly reported that SWI was the most sensitive among MRI pulse sequences (8, 25).
Despite the advantages of MRI for cell tracking, there is a drawback in using SPIO, i.e., the credibility of SPIO decreases as time passes. The amount of SPIO nanoparticles within the cell probably decreases because of cell division and hydrolytic digestion of SPIO in the cell. Jülke et al. (26) reported that the MRI signal detectability decreased as the SPIO-labeled stem cell's passage continued. Another factor is that SPIO-labeled cells can be taken up by local macrophages (27). However, in an vitro model study, the iron transfer rate from SPIO-labeled cells to the bystander macrophage was less than 10%, indicating that the uptake rate of the SPIO-labeled cells to the macrophage is fairly minor (27, 28). Our result on day 14 after stem cell injection showed that iron positive cells matched well with anti-human mitochondria positive cells. This indicates that SPIO-labeled cells were the implanted stem cells. Nevertheless, because the histological data were only obtained on day 14 after injection, and only 6 animals were used, additional studies should be performed to confirm these results at varied times and using more animals.
We are aware of limitations of this study. First, the number of animals in each group was small. Further studies using larger animal numbers is required to confirm the effectiveness of three-dimensional MRI visualization of engrafted cells. Second, the histological assessment of this study was performed with stained tissue from the center of the infarction only. The ideal progress of histological evaluation should be performed using sections through the entire cerebral infarction. Our results did not include complete infarction data. Third, all of the histology sections were obtained on day 14 after injection. Moreover, early time correlation with MRIs should be performed.
In conclusion, three-dimensional reconstruction of MR images is feasible and is helpful to understand the positional relationship between SPIO-labeled hBM-MSCs and the cerebral infarction as well as to determine their volumes. The engraftment appeared while encircling the cerebral infarction. There was more engraftment using the ICA injection than the IV injection initially. SWI was the most sensitive pulse sequence to detect SPIO-labeled cells.
Figures and Tables
Fig. 1
Three-dimensional reconstruction of MR images of photothrombotic cerebral infarction and superparamagnetic iron oxide (SPIO)-labeled stem cells in rat model.
A. Using Philips clinical workflow solution, infarction area was assigned color yellow, and dark region due to SPIO-labeled human bone marrow-derived mesenchymal stem cells was assigned color red. Three-dimensional reconstruction was performed using volume-rendering mode. B. Subsequently, volumes of infarction and SPIO were obtained (mm3) using calculate-volume tool.
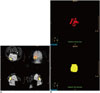
Fig. 2
Confirmation of labeling stem cells with superparamagnetic iron oxide (SPIO) nanoparticles.
Un-labeled human bone marrow-derived mesenchymal stem cells (hBM-MSCs) (A) and SPIO-labeled hBM-MSCs at 50 µg Fe/mL (B) stained with Perls' reagent. All cells in B were sufficiently labeled without any abnormal changes in morphology (× 400, Prussian blue staining).
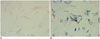
Fig. 3
Two-dimensional (2D) and three-dimensional (3D) reconstruction of phantom MR images.
Solidified agarose phantom had 2.2-mm diameter U-shaped column with 6.0 × 102 superparamagnetic iron oxide (SPIO)-labeled stem cells in total volume of 200-µL. A. Column is seen as U-shaped dark signal region on T2-weighted images (T2WI), T2*-weighted images (T2*WI), and susceptibility-weighted images (SWI). Dark signal region is large and prominent on SWI, followed by T2*WI, and then T2WI. B. In three-dimensionally reconstructed images, color red was assigned to dark signal region due to SPIO-labeled human bone marrow-derived mesenchymal stem cells. Volumes were 25.9, 68.4, and 129.3 µL on T2WI, T2*WI, and SWI, respectively.

Fig. 4
Time-elapsed MR images of photothrombotic cerebral infarction and superparamagnetic iron oxide (SPIO)-labeled stem cells in rat model.
MR images were obtained on day 2 after photothrombotic cerebral infarction (pre, 1 day before cell injection) and on days 1, 3, 7, and 14 after internal carotid arterial injection of SPIO-labeled human bone marrow-derived mesenchymal stem cells. As time progressed, high signal intensity of infarction (⊚) was reduced in size and intensity, and dark signal region (arrowheads) appeared by encircling infarction from day 1 until day 14, indicating engraftment of stem cells to infarction. Dark regions were large and prominent in susceptibility-weighted images (SWI), followed by T2*-weighted images (T2*WI), and then by T2-weighted images (T2WI).
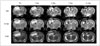
Fig. 5
Correlation between MR image and histology.
Dark signal region (A) encircling lateral margin of cerebral infarction (⊚) on MR image (day 14) was correlated with hematoxylin and eosin (HE) staining (B, × 12.5), Prussian blue (PB) staining (C, × 100), and immunohistochemistry (IHC) with anti-human mitochondria antibody (D, × 100). On HE staining, infarction (⊚) and cystic encephalomalacia (**) were seen in right parietal lobe (A, B). On PB staining and IHC, dark regions on MR image were confirmed as superparamagnetic iron oxide-labeled human bone marrow-derived mesenchymal stem cells (A, C, D).
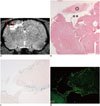
Fig. 6
Correlation between Prussian blue and immunohistochemistry (IHC) staining.
Two images of Prussian blue staining (A, × 200) and IHC with anti-human mitochondria antibody (B, × 200) of same slide are superimposed (C). Positive cells can be seen at periphery of cerebral infarction. Blue-color stained iron positive cells (A) were thoroughly matched to green fluorescent human mitochondria positive cells (B). Engraftment of implanted superparamagnetic iron oxide-labeled human bone marrow-derived mesenchymal stem cells was confirmed by matching.
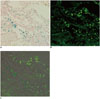
Fig. 7
Ventral perspective of three-dimensional reconstruction of MR images of photothrombotic cerebral infarction and superparamagnetic iron oxide (SPIO)-labeled human bone marrow-derived mesenchymal stem cells (hBM-MSCs) in rats.
Color yellow was assigned to cerebral infarction, and color red was assigned to SPIO-labeled hBM-MSCs surrounding infarction. Internal carotid arterial (ICA) injection (A) of SPIO-labeled hBM-MSCs shows engraftment of more cells in earlier days than intravenous (IV) injection (B). SWI = susceptibility-weighted images, T2WI = T2-weighted images, T2*WI = T2*-weighted images
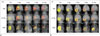
Fig. 8
Volumetric analysis of superparamagnetic iron oxide (SPIO)-labeled human bone marrow-derived mesenchymal stem cells (hBM-MSCs) and cerebral infarction.
A. Volumes of engrafted SPIO-labeled hBM-MSCs in internal carotid arterial (ICA) group were larger than those in intravenous (IV) group on days 1, 3, and 7 (not on day 14) after injection on susceptibility-weighted images (SWI) (p < 0.05), but not on T2*-weighted images (T2*WI) and T2-weighted images (T2WI) (p > 0.05). SWI was most sensitive in detecting SPIO-labeled cells among MRI pulse sequences (p < 0.05). B. Volume of infarction decreased as time progressed, i.e., from day 2 after photothrombotic cerebral infarction (1 day before cell injection) until day 14 after stem cell injection in all 6 rats. Between ICA and IV groups, there were no differences in volume of cerebral infarction (p > 0.05).
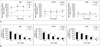
References
1. Yang Y, Zhang J, Qian Y, Dong S, Huang H, Boada FE, et al. Superparamagnetic iron oxide is suitable to label tendon stem cells and track them in vivo with MR imaging. Ann Biomed Eng. 2013; 41:2109–2119.
2. Bulte JW. In vivo MRI cell tracking: clinical studies. AJR Am J Roentgenol. 2009; 193:314–325.
3. Richards JM, Shaw CA, Lang NN, Williams MC, Semple SI, MacGillivray TJ, et al. In vivo mononuclear cell tracking using superparamagnetic particles of iron oxide: feasibility and safety in humans. Circ Cardiovasc Imaging. 2012; 5:509–517.
4. Hu SL, Lu PG, Zhang LJ, Li F, Chen Z, Wu N, et al. In vivo magnetic resonance imaging tracking of SPIO-labeled human umbilical cord mesenchymal stem cells. J Cell Biochem. 2012; 113:1005–1012.
5. Reddy AM, Kwak BK, Shim HJ, Ahn C, Cho SH, Kim BJ, et al. Functional characterization of mesenchymal stem cells labeled with a novel PVP-coated superparamagnetic iron oxide. Contrast Media Mol Imaging. 2009; 4:118–126.
6. Wang L, Deng J, Wang J, Xiang B, Yang T, Gruwel M, et al. Superparamagnetic iron oxide does not affect the viability and function of adipose-derived stem cells, and superparamagnetic iron oxide-enhanced magnetic resonance imaging identifies viable cells. Magn Reson Imaging. 2009; 27:108–119.
7. Byun JS, Kwak BK, Kim JK, Jung J, Ha BC, Park S. Engraftment of human mesenchymal stem cells in a rat photothrombotic cerebral infarction model: comparison of intra-arterial and intravenous infusion using MRI and histological analysis. J Korean Neurosurg Soc. 2013; 54:467–476.
8. Ha BC, Jung J, Kwak BK. Susceptibility-weighted imaging for stem cell visualization in a rat photothrombotic cerebral infarction model. Acta Radiol. 2015; 56:219–227.
9. Xu HS, Ma C, Cao L, Wang JJ, Fan XX. Study of co-transplantation of SPIO labeled bone marrow stromal stem cells and Schwann cells for treating traumatic brain injury in rats and in vivo tracing of magnetically labeled cells by MRI. Eur Rev Med Pharmacol Sci. 2014; 18:520–525.
10. Detante O, Valable S, de Fraipont F, Grillon E, Barbier EL, Moisan A, et al. Magnetic resonance imaging and fluorescence labeling of clinical-grade mesenchymal stem cells without impacting their phenotype: study in a rat model of stroke. Stem Cells Transl Med. 2012; 1:333–341.
11. Vasconcelos-dos-Santos A, Rosado-de-Castro PH, Lopes de Souza SA, da Costa Silva J, Ramos AB, Rodriguez de Freitas G, et al. Intravenous and intra-arterial administration of bone marrow mononuclear cells after focal cerebral ischemia: is there a difference in biodistribution and efficacy? Stem Cell Res. 2012; 9:1–8.
12. Walczak P, Zhang J, Gilad AA, Kedziorek DA, Ruiz-Cabello J, Young RG, et al. Dual-modality monitoring of targeted intraarterial delivery of mesenchymal stem cells after transient ischemia. Stroke. 2008; 39:1569–1574.
13. Johnson GA, Calabrese E, Badea A, Paxinos G, Watson C. A multidimensional magnetic resonance histology atlas of the Wistar rat brain. Neuroimage. 2012; 62:1848–1856.
14. Seki F, Hikishima K, Nambu S, Okanoya K, Okano HJ, Sasaki E, et al. Multidimensional MRI-CT atlas of the naked mole-rat brain (Heterocephalus glaber). Front Neuroanat. 2013; 7:45.
15. Daadi MM, Li Z, Arac A, Grueter BA, Sofilos M, Malenka RC, et al. Molecular and magnetic resonance imaging of human embryonic stem cell-derived neural stem cell grafts in ischemic rat brain. Mol Ther. 2009; 17:1282–1291.
16. Jung J, Kwak BK, Reddy AM, Ha BC, Shim HJ, Byun JS, et al. Characterization of photothrombotic cerebral infarction model at sensorimotor area of functional map in rat. J Neurol Sci-Turk. 2013; 30:617–628.
17. Shen LH, Li Y, Chen J, Zhang J, Vanguri P, Borneman J, et al. Intracarotid transplantation of bone marrow stromal cells increases axon-myelin remodeling after stroke. Neuroscience. 2006; 137:393–399.
18. Chen J, Zhang ZG, Li Y, Wang L, Xu YX, Gautam SC, et al. Intravenous administration of human bone marrow stromal cells induces angiogenesis in the ischemic boundary zone after stroke in rats. Circ Res. 2003; 92:692–699.
19. Guzman R, Choi R, Gera A, De Los Angeles A, Andres RH, Steinberg GK. Intravascular cell replacement therapy for stroke. Neurosurg Focus. 2008; 24:E15.
20. Pendharkar AV, Chua JY, Andres RH, Wang N, Gaeta X, Wang H, et al. Biodistribution of neural stem cells after intravascular therapy for hypoxic-ischemia. Stroke. 2010; 41:2064–2070.
21. Lundberg J, Le Blanc K, Söderman M, Andersson T, Holmin S. Endovascular transplantation of stem cells to the injured rat CNS. Neuroradiology. 2009; 51:661–667.
22. Li L, Jiang Q, Ding G, Zhang L, Zhang ZG, Li Q, et al. Effects of administration route on migration and distribution of neural progenitor cells transplanted into rats with focal cerebral ischemia, an MRI study. J Cereb Blood Flow Metab. 2010; 30:653–662.
23. Fischer UM, Harting MT, Jimenez F, Monzon-Posadas WO, Xue H, Savitz SI, et al. Pulmonary passage is a major obstacle for intravenous stem cell delivery: the pulmonary first-pass effect. Stem Cells Dev. 2009; 18:683–692.
24. Parr AM, Tator CH, Keating A. Bone marrow-derived mesenchymal stromal cells for the repair of central nervous system injury. Bone Marrow Transplant. 2007; 40:609–619.
25. Cheng JL, Yang YJ, Li HL, Wang J, Wang MH, Zhang Y. In vivo tracing of superparamagnetic iron oxide-labeled bone marrow mesenchymal stem cells transplanted for traumatic brain injury by susceptibility weighted imaging in a rat model. Chin J Traumatol. 2010; 13:173–177.
26. Jülke H, Veit C, Ribitsch I, Brehm W, Ludewig E, Delling U. Comparative labelling of equine and ovine multipotent stromal cells with superparamagnetic iron oxide particles for magnetic resonance imaging in vitro. Cell Transplant. 2013; 12. 10. [Epub].
27. Pawelczyk E, Arbab AS, Chaudhry A, Balakumaran A, Robey PG, Frank JA. In vitro model of bromodeoxyuridine or iron oxide nanoparticle uptake by activated macrophages from labeled stem cells: implications for cellular therapy. Stem Cells. 2008; 26:1366–1375.
28. Cohen ME, Muja N, Fainstein N, Bulte JW, Ben-Hur T. Conserved fate and function of ferumoxides-labeled neural precursor cells in vitro and in vivo. J Neurosci Res. 2010; 88:936–944.
Supplementary Materials
The online-only Data Supplement is available with this article at http://dx.doi.org/10.3348/kjr.2015.16.3.575.