Abstract
Ultrasound-guided percutaneous radiofrequency (RF) ablation has become one of the most promising local cancer therapies for both resectable and nonresectable hepatic tumors. Although RF ablation is a safe and effective technique for the treatment of liver tumors, the outcome of treatment can be closely related to the location and shape of the tumors. There may be difficulties with RF ablation of tumors that are adjacent to large vessels or extrahepatic heat-vulnerable organs and tumors in the caudate lobe, possibly resulting in major complications or treatment failure. Thus, a number of strategies have been developed to overcome these challenges, which include artificial ascites, needle track ablation, fusion imaging guidance, parallel targeting, bypass targeting, etc. Operators need to use the right strategy in the right situation to avoid the possibility of complications and incomplete thermal tissue destruction; with the right strategy, RF ablation can be performed successfully, even for hepatic tumors in high-risk locations. This article offers technical strategies that can be used to effectively perform RF ablation as well as to minimize possible complications related to the procedure with representative cases and schematic illustrations.
Since the first clinical study in 1995, the clinical implications of ultrasound (US)-guided percutaneous radiofrequency (RF) ablation for the treatment of hepatocellular carcinoma (HCC) and liver metastasis have expanded (123). Currently, RF ablation is recognized as a curative modality for early-stage HCC, the outcomes of which are comparable with those of surgical resection, as evidenced by many studies (12345). RF ablation for liver tumors is widely performed because of its ease of use, relatively low cost, short hospitalization time, and minimal invasiveness (6). Furthermore, major complications of hepatic RF ablation have been reported as occurring rarely, ranging between 2% and 3%, and complications were less observed in cases of HCC (1.8%) as opposed to metastasis (5.8%) (789). Although the treatment algorithms differ slightly among nations, RF ablation is basically recommended for patients with three or fewer HCCs that are 3 cm in diameter or smaller who are contraindicated for surgery (10).
The local recurrence rate after RF ablation for HCCs ranged from 1.7% to 41% (11). Local recurrence appears to arise from incompletely treated viable tumors, satellite tumor nests, and microscopic vascular invasion (1213). Although local tumor progression can occur even after successful RF ablation, local recurrence rates differ significantly between patients with and without an adequate safety margins (1415). Therefore, when performing RF ablation, it should be the ultimate goal of the procedure to ensure a sufficient ablative margin in all directions that surround the index tumor in order to reduce the local recurrence rate. Further, it is also important for the operator to complete the procedure with no complications even in challenging settings.
In this article, we discuss and illustrate technical strategies to minimize complications and treatment failures of RF ablation on the basis of a review of the literature and our experience.
Although RF ablation is widely performed to treat liver tumors, most if not all radiologists who are asked to perform percutaneous US-guided RF ablation have a single major concern: how to successfully perform RF ablation with no complications. There are a number of strategies that might be helpful for preventing complications during the procedure (Table 1).
When a tumor is located near heat-vulnerable organs, such as the gastrointestinal tract or the diaphragm, it is not straightforward to successfully perform RF ablation because of the risk of collateral thermal damage to the adjacent organs. Further, if an index tumor is situated in the hepatic dome, the visualization of the tumor with US is obscured by the lung (16). To overcome these difficulties, artificial ascites (AA) can be effectively used. Indeed, the AA method is safe and practical when performing RF ablation for tumors adjacent to heat-vulnerable organs because it can separate the RF ablation zone from the adjacent organs and improve the visibility of an index tumor (1718).
If AA is difficult in cases with peritoneal adhesion because of prior surgery, artificial pleural effusion may be an alternative to improve the sonic window when performing RF ablation for an index tumor in the hepatic dome (1920). However, in contrast with AA, artificial pleural effusion cannot protect the diaphragm from collateral thermal injury (21).
In order to obtain safe access to the peritoneal space, the Seldinger technique is used. First, a relevant route for AA should be selected according to the tumor location. After the optimal route is chosen, the peritoneal cavity is punctured with an 18-gauge sheathed needle under US guidance. After a stylet is removed, a round-tipped guidewire is advanced through the lumen of the hollow needle while the patient breathes shallowly. Finally, a 4- to 6-French angiosheath is inserted along the guidewire into the peritoneal space for the infusion of 500-1000 mL of 5% dextrose solution (17).
One of four different infusion routes may be selected according to the location of hepatic tumors (Fig. 1). If a tumor is located in the right lobe and segment 4, a perihepatic route through the right 7-8 intercostal space or a sub-hepatic route below the hepatic angle along the anterior axillary line is recommended (Fig. 2). If a tumor is found within segments 2, 3, and 4 abutting the diaphragm, a sub-xiphoid (sub-phrenic) route is appropriate for AA. If a tumor is located in segments 2 and 3 abutting the stomach, a gastrohepatic (lesser sac) route may be chosen (Fig. 3). Based on our experience, the infused fluid via a gastrohepatic route tends to flow into another peritoneal space, such as the sub-hepatic space, without fluid retention in the lesser sac. Therefore, in cases when a gastrohepatic route is used, an angiosheath needs to be inserted around the tumor, and fluid should be continuously infused during RF ablation.
Even when AA infusion is performed successfully, hydro-dissection may not be sufficient to prevent potential thermal injury. In such cases, leverage (lifting) can be helpful for widening the distance between the target tumor and the surrounding organs. If the handle of the needle is tilted in the caudal direction after an electrode is placed in the tumor, the liver moves toward the cranial direction (Fig. 4). This leverage effect, in turn, may allow for a wider gap between the ablation zone and the heat-vulnerable organs and can lead to a successful procedure (Fig. 5). Although AA plays a role as an effective thermal barrier, it is also important to adjust the ablation time by stopping the procedure when microbubbles generated around the needle tip abut on the extrahepatic organs in order to minimize collateral damage (22).
Bleeding usually results from mechanical damage to the vessels during electrode placement, especially in patients with coagulopathy (923). A serum platelet count of 50000/µL and an international normalized ratio of 1.5 are often used as threshold values for performing RF ablation (24). Therefore, at our institution, common coagulation tests, including prothrombin time, activated partial thromboplastin time and platelet count, are routinely performed before the procedure. To minimize the risk of bleeding, the procedure needs to be postponed until corrective measures including platelet and fresh frozen plasma transfusions are taken (9). In addition, there are a number of tips for reducing vascular complications, which include avoiding vascular transgression, minimizing the number of electrodes that are repositioned, traversing sufficient normal hepatic parenchyma before reaching the tumor, and ablating the needle tract after the procedure (724).
Given that the liver is much more movable in the craniocaudal direction during deep breathing than shallow breathing, the risk of a tear of the hepatic parenchyma could be increased during needle placement if patients breathe deeply. Thus, we recommend performing RF ablation when patients breathe shallowly. In any case, deep breathing should be avoided during electrode placement, especially if the trajectory of the electrode is not perpendicular to the liver parenchyma.
Occasionally, vascular insults to the intercostal vessels may occur (9). The intercostal vessels only run along the inferior margin in the posterior arc of the rib, whereas the vessels run along both the superior and inferior margins in the anterior arc of the rib. Therefore, when using an intercostal approach, an electrode should cautiously penetrate through the middle portion of the intercostal space to avoid penetrating the vessels.
Bile duct injury can result from direct mechanical trauma during needle placement or thermal damage, which may lead to bile leakage, bile peritonitis, hemobilia, cholangitis, biliary stricture, biloma, or biliary fistula (9). RF ablation for tumors that are close to the hepatic hilum may be at risk of injuring adjacent bile ducts (25). This is because the risk of injury to the central bile ducts is directly proportional to efforts to overcome the heat-sink effect of the larger vessel around the central mass (7). Thus, the operator should strike a balance between the potential risk of bile duct injury and the extent of the RF ablation zone. A minimum distance of 5 mm or more between the electrode tip and the bile ducts is usually required for preventing severe and irreversible bile duct injury (26). Ogawa et al. (27) described that cooling the bile ducts with an endoscopic nasobiliary drainage tube was an effective way to prevent biliary complications during RF ablation.
Tumor seeding is a rare but feared complication of RF ablation (923). According to previous reports, the overall risk of needle tract seeding ranged between 0.2% and 2.8% (782829). The risk factors for neoplastic seeding include use of a large-gauge electrode, multiple punctures, poor tumor differentiation, subcapsular tumors, and a high alpha-fetoprotein level (9293031). Potential strategies to reduce the risk of tumor seeding include minimizing the number of punctures and repositioning the electrode and traversing the normal hepatic parenchyma as much as possible before accessing the tumor (9). Cauterizing the needle tract upon completion of the procedure may be an option for preventing needle tract seeding (29). In addition, to prevent transportal spread by sudden rupture (popping) of an exophytic tumor during the procedure, gradually increasing the RF energy is needed (23).
There are many causes for incomplete RF thermal ablation, including both technical factors related to the procedure and biological aspects of the index tumor (9). Given that ablation zones with ablative margins less than 5 mm from the tumor border are significantly associated with local tumor progression (15), it is crucial to obtain a sufficient ablative margin in order to minimize local tumor progression. A number of investigators have suggested that the location and shape of the tumors are closely related to treatment outcomes (6222332333435). For example, RF ablation for tumors in close proximity to large vessels might often lead to insufficient ablation owing to a heat-sink effect (22). It has been considered technically challenging to perform the procedure for central tumors adjacent to the hepatic hilum because of the risk of traversing large vessels and central bile ducts (9). Thus, in these difficult settings, various technical strategies should be used to overcome the possibility of treatment failure, by which RF ablation can be performed successfully, even for index tumors in high-risk locations (Table 1).
Although among the various imaging modalities including US, CT, MR imaging and fluoroscopy, US is most widely used for guiding RF ablation because of its numerous strengths, the procedure may not be feasible when the index tumor is too inconspicuous to be localized with conventional gray-scale US. In this situation, fusion imaging guidance and contrast-enhanced US can be used to enhance the conspicuity of the index tumor (21).
Fusion imaging guidance makes use of real-time US coupled with CT or MR images. This technique is especially useful for targeting small index tumors that are not clearly seen under conventional US. Indeed, a fusion imaging technique allows the operator to perform the procedure with higher confidence because the anatomic information from CT or MR imaging is simultaneously displayed on real-time US images (Fig. 6) (213637).
Contrast-enhanced US is also effective for guiding RF ablation in cases that are not highly visible with conventional US. Using this technique, the operator can detect the index tumor by imaging findings such as hyper-enhancement in the arterial phase or defects in the background of enhanced liver in the post-vascular phase (213839).
Local tumor progression after RF ablation is closely related to the presence of vessels that are at least 3 mm in size and contiguous to the tumor because of the heat-sink effect from blood flow (3440). In addition, during RF ablation for perivascular tumors, there may be increased risk of vascular complications (41) because vessels could be damaged directly by the electrode and indirectly by the heat induced inside the tumor. Thus, a number of strategies to increase the ablation zone by minimizing the heat-sink effect have been developed for when the tumor is near large vessels. These include RF ablation combined with transcatheter arterial chemoembolization, RF ablation using multiple overlapping, using large electrodes and a more powerful generator, dual switching monopolar RF ablation using separable clustered electrodes, and "parallel" targeting (612414243444546).
"Parallel" targeting is a method in which a needle electrode is inserted near a large vessel's lumen parallel to the vessel wall in order to enlarge the contact surface between the ablation zone and the vessel, which may lead to a wider ablation zone (Fig. 7) (6). Parallel targeting along the large vessel may reduce the heat-sink effect and, in turn, produce the confluent shape of the ablation zone. Indeed, in order to overcome the heat-sink effect, an electrode needs to be positioned as close as possible to the large vessels that are in contact with the tumor (33). However, it might be impractical in cases when the tumor encases a large vessel or is adjacent to multiple large vessels.
If the shape of an index tumor is ovoid, the straight cooled-tip electrode should be placed along the long axis of the mass rather than its short axis because the electrode frequently makes an ablation zone elliptical (32). Thereby, the number of punctures during the procedure can be decreased while securing a sufficient ablation zone.
With subcapsular tumors that grow exophytically from the liver, it may be difficult to obtain complete ablation. A useful strategy for successful ablation of an exophytic tumor is to ablate the intrahepatic portion first, thus obstructing the blood supply to the exophytic portion, which should be ablated second (32).
Radiofrequency ablation of liver tumors located in the caudate lobe is technically feasible, despite the deep location and proximity to the major vessels and central bile ducts (33). However, it is still a heavy burden for operators to perform the procedure for index tumors in the caudate lobe for the following reasons: Firstly, given the anatomically deep-seated position of the caudate lobe, it is challenging to maneuver an electrode with a long trajectory. Secondly, there are major vessels surrounding the caudate lobe, such as the inferior vena cava and the central right portal vein, that make it difficult to plan the needle tract in order not to injure central vessels. In this regard, "bypass" targeting may be useful for successful treatment without puncturing vessels.
Large vessels and central bile ducts that are located in front of a liver tumor become obstacles for targeting the index tumor using a needle electrode. In such cases, RF ablation can be considered technically impossible to perform from an operator's viewpoint. However, "bypass" targeting may be a solution. Bypass targeting is a method that helps in placing a needle electrode without puncturing any large vessels or intrahepatic bile ducts that are adjacent to an index tumor. This technique takes advantage of the structural flexibility of the liver and can be accomplished by bending the trajectory of the straight electrode into a gentle curved shape. Indeed, this method could make it possible to insert a needle into target lesions nearly anywhere in the liver without traversing large vessels (Fig. 8). However, it may still be limited in cases with severe cirrhotic changes or a narrow gap between the index tumor and obstacles.
Ultrasound-guided percutaneous RF ablation has been widely used for HCCs and metastases in the liver, and it can achieve overall and disease-free survival rates that are comparable with those of surgery for patients with early-stage HCC. In order to reduce local recurrence rates after RF ablation, it is of utmost importance to ensure a sufficient ablative margin. However, when index tumors are in high-risk locations within the liver, the procedure may result in undesirable complications followed by insufficient treatment. To overcome these challenges, operators should be familiar with multiple technical strategies, such as AA, needle track ablation, fusion imaging guidance, parallel targeting, and bypass targeting. These methods may be quite helpful for overcoming technical difficulties related to the location and shape of the tumors as well as for safely completing the procedure. Thereby, RF ablation can be successfully performed with curative intent.
Figures and Tables
Fig. 1
Schematic illustration shows four different routes for artificial ascites (AA) infusion.
Perihepatic, sub-hepatic, sub-xiphoid (sub-phrenic), and gastrohepatic (lesser sac) routes can be selectively used for AA infusion based on location of hepatic tumors (T).
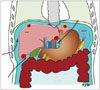
Fig. 2
RF ablation using AA via sub-hepatic route in 44-year-old man.
A. Longitudinal US image shows hypoechoic HCC (arrows) in segment 6 near ascending colon (arrowhead). B. Longitudinal US image demonstrates inserted angiosheath (arrowhead) and AA (asterisk) in sub-hepatic space. C. Longitudinal US image during RF ablation depicts transient hyperechoic ablation zone (asterisk), needle electrode (arrow), and angiosheath (arrowhead). D. Immediate follow-up contrast-enhanced CT image reveals low-attenuated RF ablation zone (asterisk) that sufficiently covers index tumor as well as intact adjacent colon (arrowhead). AA = artificial ascites, HCC = hepatocellular carcinoma, RF = radiofrequency, US = ultrasound
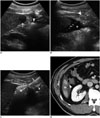
Fig. 3
RF ablation using AA method via gastrohepatic (lesser sac) route in 63-year-old woman.
A. Sagittal US image depicts hypoechoic HCC (arrows) in segment 3 near stomach (S). B. Photograph shows inserted angiosheath (arrowhead) through gastrohepatic route and needle electrode into target tumor. C. Sagittal US image during procedure demonstrates transient hyperechoic cloud (asterisk) and AA (arrow) in lesser sac between liver and stomach (S). D. Immediate follow-up contrast-enhanced CT image reveals low-attenuated RF ablation zone (asterisk) that sufficiently covers index tumor as well as intact adjacent gastric wall (arrow). AA = artificial ascites, HCC = hepatocellular carcinoma, RF = radiofrequency, US = ultrasound
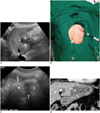
Fig. 4
Schematic illustrations show mechanism of leverage (lifting) method.
A. Schematic illustration depicts insufficient hydrodissection between target tumor (T) and adjacent colon (C) after artificial ascites infusion. B. Schematic illustration demonstrates caudal tilting of distal end (handle) of needle electrode by which gap between index tumor (T) and abutting colon (C) is widened.
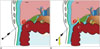
Fig. 5
RF ablation using leverage (lifting) method in 73-year-old man.
A. Longitudinal US image depicts index tumor with mixed echogenecity (arrows) in segment 6. There is still narrow gap (arrowhead) between tumor and colon (C) even after AA (asterisk) infusion. B. Longitudinal US image during procedure shows that gap (arrowhead) between RF ablation zone (asterisk) and colon (C) is not wide enough to prevent possible thermal damage to colon. Arrow indicates electrode. C. Photograph demonstrates caudal tilting of distal end of electrode by hand (arrow) (leverage method). D. Longitudinal US image during RF ablation shows wider gap (arrowhead) between RF ablation zone (asterisk) and colon (C) that is generated by leverage method. Note less steep slope of electrode (arrow) compared with that shown in B. E. Immediate follow-up contrast-enhanced CT image shows low-attenuated RF ablation zone (asterisk) that sufficiently covers tumor and intact adjacent colon (arrowhead) that is surrounded by infused AA. AA = artificial ascites, RF = radiofrequency, US = ultrasound
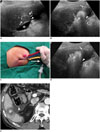
Fig. 6
RF ablation under fusion imaging guidance in 67-year-old man with 2.1 cm HCC.
A. Gadoxetic acid-enhanced axial arterial-phase T1-weighted image shows enhancing mass (arrow) in segment 7 of liver. B. Index tumor is not clearly seen on conventional gray-scale US image. HCC = hepatocellular carcinoma, RF = radiofrequency, US = ultrasound C. RF ablation was performed using fusion imaging technique (volume navigation, GE Healthcare). Fused image (left) and its corresponding MR image (right) during procedure show index tumor (T) and transient hyperechoic microbubbles (asterisk). D. Immediate follow-up contrast-enhanced CT image depicts low-attenuated RF ablation zone (asterisk), which sufficiently covers index tumor (arrowhead). HCC = hepatocellular carcinoma, RF = radiofrequency
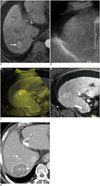
Fig. 7
RF ablation using parallel targeting along large vessel in 50-year-old man.
A. Gadoxetic acid-enhanced axial hepatobiliary-phase T1-weighted image shows metastasis (arrow) from rectal cancer in segment 7 abutting right hepatic vein (arrowhead). B. Intercostal US image depicts hypoechoic metastasis (arrowhead) abutting right hepatic vein (arrow), which can potentially cause heat-sink effect during RF ablation. C. Intercostal US image during procedure demonstrates needle (arrow) in parallel to right hepatic vein (arrowhead) in order to enlarge contact surface between ablation zone and vessel. D. Immediate follow-up contrast-enhanced CT image shows elongated low-attenuated RF ablation zone (asterisk) along right hepatic vein (arrowhead), which sufficiently covers index tumor, as well as intact right hepatic vein. RF = radiofrequency, US = ultrasound
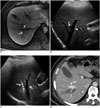
Fig. 8
RF ablation using bypass targeting technique in 66-year-old man.
A. Pre-RF ablation contrast-enhanced CT image shows HCC (curved arrow) in segment 1 near main portal vein and inferior vena cava (IVC) and right portal vein (arrowhead) on expected approach path (dotted arrow) of electrode. B. Intercostal US image demonstrates hypoechoic HCC (curved arrow) between main portal vein and IVC in segment 1. Note that right portal vein (arrowhead) blocks approach path for needle insertion into index tumor. C. Contrast-enhanced CT image obtained from 5 mm below level of A depicts safe approach path (dotted arrow) without traversing right portal vein. D. Serial schematic illustrations show process of placing electrode while bypassing large vessel in front of target tumor using structural flexibility of liver. HCC = hepatocellular carcinoma, RF = radiofrequency, US = ultrasound E. Intercostal US image during procedure shows that electrode (arrowhead) is safely placed within index tumor (arrow) using bypass targeting without puncturing right portal vein. F. Immediate follow-up contrast-enhanced CT image reveals low-attenuated RF ablation zone (asterisk) and intact right portal vein (arrowhead) with no signs of penetration by electrode. RF = radiofrequency, US = ultrasound
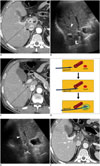
Table 1
Technical Tips for Safe and Complete RF Ablation
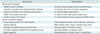
Acknowledgments
We thank Shin Il Kim, PhD and Joon Yeon Kim, PhD of Chonnam National University (Hwasun) Hospital for their support in performing RF ablation.
References
1. Kim YS, Lim HK, Rhim H, Lee MW, Choi D, Lee WJ, et al. Ten-year outcomes of percutaneous radiofrequency ablation as first-line therapy of early hepatocellular carcinoma: analysis of prognostic factors. J Hepatol. 2013; 58:89–97.
2. Shiina S, Tateishi R, Arano T, Uchino K, Enooku K, Nakagawa H, et al. Radiofrequency ablation for hepatocellular carcinoma: 10-year outcome and prognostic factors. Am J Gastroenterol. 2012; 107:569–577. quiz 578.
3. Giannini EG, Farinati F, Del Poggio P, Rapaccini GL, Di Nolfo MA, Benvegnù L, et al. Ten-year outcome of radiofrequency thermal ablation for hepatocellular carcinoma: an Italian experience. Am J Gastroenterol. 2012; 107:1588–1589. author reply 1590.
4. Forner A, Llovet JM, Bruix J. Hepatocellular carcinoma. Lancet. 2012; 379:1245–1255.
5. Livraghi T, Meloni F, DiStasi M, Rolle E, Solbiati L, Tinelli C, et al. Sustained complete response and complications rates after radiofrequency ablation of very early hepatocellular carcinoma in cirrhosis: is resection still the treatment of choice? Hepatology. 2008; 47:82–89.
6. Thanos L, Mylona S, Galani P, Pomoni M, Pomoni A, Koskinas I. Overcoming the heat-sink phenomenon: successful radiofrequency thermal ablation of liver tumors in contact with blood vessels. Diagn Interv Radiol. 2008; 14:51–56.
7. Rhim H, Yoon KH, Lee JM, Cho Y, Cho JS, Kim SH, et al. Major complications after radio-frequency thermal ablation of hepatic tumors: spectrum of imaging findings. Radiographics. 2003; 23:123–134. discussion 134-136.
8. Livraghi T, Solbiati L, Meloni MF, Gazelle GS, Halpern EF, Goldberg SN. Treatment of focal liver tumors with percutaneous radio-frequency ablation: complications encountered in a multicenter study. Radiology. 2003; 226:441–451.
9. Mendiratta-Lala M, Brook OR, Midkiff BD, Brennan DD, Thornton E, Faintuch S, et al. Quality initiatives: strategies for anticipating and reducing complications and treatment failures in hepatic radiofrequency ablation. Radiographics. 2010; 30:1107–1122.
10. Shibata T, Isoda H, Hirokawa Y, Arizono S, Shimada K, Togashi K. Small hepatocellular carcinoma: is radiofrequency ablation combined with transcatheter arterial chemoembolization more effective than radiofrequency ablation alone for treatment? Radiology. 2009; 252:905–913.
11. Kitamoto M, Nakanishi T, Kira S, Kawaguchi M, Nakashio R, Suemori S, et al. The assessment of proliferating cell nuclear antigen immunohistochemical staining in small hepatocellular carcinoma and its relationship to histologic characteristics and prognosis. Cancer. 1993; 72:1859–1865.
12. Kim JW, Shin SS, Kim JK, Choi SK, Heo SH, Lim HS, et al. Radiofrequency ablation combined with transcatheter arterial chemoembolization for the treatment of single hepatocellular carcinoma of 2 to 5 cm in diameter: comparison with surgical resection. Korean J Radiol. 2013; 14:626–635.
13. Goldberg SN, Bonn J, Dodd G, Dupuy D, Geschwind JH, Hicks M, et al. Society of Interventional Radiology Interventional Oncology Task Force: interventional oncology research vision statement and critical assessment of the state of research affairs. J Vasc Interv Radiol. 2005; 16:1287–1294.
14. Kim YS, Lee WJ, Rhim H, Lim HK, Choi D, Lee JY. The minimal ablative margin of radiofrequency ablation of hepatocellular carcinoma (> 2 and < 5 cm) needed to prevent local tumor progression: 3D quantitative assessment using CT image fusion. AJR Am J Roentgenol. 2010; 195:758–765.
15. Nakazawa T, Kokubu S, Shibuya A, Ono K, Watanabe M, Hidaka H, et al. Radiofrequency ablation of hepatocellular carcinoma: correlation between local tumor progression after ablation and ablative margin. AJR Am J Roentgenol. 2007; 188:480–488.
16. Song I, Rhim H, Lim HK, Kim YS, Choi D. Percutaneous radiofrequency ablation of hepatocellular carcinoma abutting the diaphragm and gastrointestinal tracts with the use of artificial ascites: safety and technical efficacy in 143 patients. Eur Radiol. 2009; 19:2630–2640.
17. Rhim H, Lim HK, Kim YS, Choi D. Percutaneous radiofrequency ablation with artificial ascites for hepatocellular carcinoma in the hepatic dome: initial experience. AJR Am J Roentgenol. 2008; 190:91–98.
18. Kang TW, Lee MW, Hye MJ, Song KD, Lim S, Rhim H, et al. Percutaneous radiofrequency ablation of hepatic tumours: factors affecting technical failure of artificial ascites formation using an angiosheath. Clin Radiol. 2014; 69:1249–1258.
19. Koda M, Ueki M, Maeda Y, Mimura K, Okamoto K, Matsunaga Y, et al. Percutaneous sonographically guided radiofrequency ablation with artificial pleural effusion for hepatocellular carcinoma located under the diaphragm. AJR Am J Roentgenol. 2004; 183:583–588.
20. Minami Y, Kudo M, Kawasaki T, Chung H, Ogawa C, Inoue T, et al. Percutaneous ultrasound-guided radiofrequency ablation with artificial pleural effusion for hepatocellular carcinoma in the hepatic dome. J Gastroenterol. 2003; 38:1066–1070.
21. Kim YJ, Lee MW, Park HS. Small hepatocellular carcinomas: ultrasonography guided percutaneous radiofrequency ablation. Abdom Imaging. 2013; 38:98–111.
22. Teratani T, Yoshida H, Shiina S, Obi S, Sato S, Tateishi R, et al. Radiofrequency ablation for hepatocellular carcinoma in so-called high-risk locations. Hepatology. 2006; 43:1101–1108.
23. Kwon HJ, Kim PN, Byun JH, Kim KW, Won HJ, Shin YM, et al. Various complications of percutaneous radiofrequency ablation for hepatic tumors: radiologic findings and technical tips. Acta Radiol. 2014; 55:1082–1092.
24. Akahane M, Koga H, Kato N, Yamada H, Uozumi K, Tateishi R, et al. Complications of percutaneous radiofrequency ablation for hepato-cellular carcinoma: imaging spectrum and management. Radiographics. 2005; 25:Suppl 1. S57–S68.
25. McGhana JP, Dodd GD 3rd. Radiofrequency ablation of the liver: current status. AJR Am J Roentgenol. 2001; 176:3–16.
26. Liu N, Gao J, Liu Y, Li T, Feng K, Ma K, et al. Determining a minimal safe distance to prevent thermal injury to intrahepatic bile ducts in radiofrequency ablation of the liver: a study in dogs. Int J Hyperthermia. 2012; 28:210–217.
27. Ogawa T, Kawamoto H, Kobayashi Y, Nakamura S, Miyatake H, Harada R, et al. Prevention of biliary complication in radiofrequency ablation for hepatocellular carcinoma-Cooling effect by endoscopic nasobiliary drainage tube. Eur J Radiol. 2010; 73:385–390.
28. Kong WT, Zhang WW, Qiu YD, Zhou T, Qiu JL, Zhang W, et al. Major complications after radiofrequency ablation for liver tumors: analysis of 255 patients. World J Gastroenterol. 2009; 15:2651–2656.
29. Jaskolka JD, Asch MR, Kachura JR, Ho CS, Ossip M, Wong F, et al. Needle tract seeding after radiofrequency ablation of hepatic tumors. J Vasc Interv Radiol. 2005; 16:485–491.
30. Livraghi T, Lazzaroni S, Meloni F, Solbiati L. Risk of tumour seeding after percutaneous radiofrequency ablation for hepatocellular carcinoma. Br J Surg. 2005; 92:856–858.
31. Llovet JM, Vilana R, Brú C, Bianchi L, Salmeron JM, Boix L, et al. Increased risk of tumor seeding after percutaneous radiofrequency ablation for single hepatocellular carcinoma. Hepatology. 2001; 33:1124–1129.
32. Rhim H, Goldberg SN, Dodd GD 3rd, Solbiati L, Lim HK, Tonolini M, et al. Essential techniques for successful radiofrequency thermal ablation of malignant hepatic tumors. Radiographics. 2001; 21 Spec No:S17–S35. discussion S36-S39.
33. Seror O, Haddar D, N'Kontchou G, Ajavon Y, Trinchet JC, Beaugrand M, et al. Radiofrequency ablation for the treatment of liver tumors in the caudate lobe. J Vasc Interv Radiol. 2005; 16:981–990.
34. Lu DS, Raman SS, Limanond P, Aziz D, Economou J, Busuttil R, et al. Influence of large peritumoral vessels on outcome of radiofrequency ablation of liver tumors. J Vasc Interv Radiol. 2003; 14:1267–1274.
35. Cha DI, Lee MW, Rhim H, Choi D, Kim YS, Lim HK. Therapeutic efficacy and safety of percutaneous ethanol injection with or without combined radiofrequency ablation for hepatocellular carcinomas in high risk locations. Korean J Radiol. 2013; 14:240–247.
36. Lee MW, Rhim H, Cha DI, Kim YJ, Choi D, Kim YS, et al. Percutaneous radiofrequency ablation of hepatocellular carcinoma: fusion imaging guidance for management of lesions with poor conspicuity at conventional sonography. AJR Am J Roentgenol. 2012; 198:1438–1444.
37. Kunishi Y, Numata K, Morimoto M, Okada M, Kaneko T, Maeda S, et al. Efficacy of fusion imaging combining sonography and hepatobiliary phase MRI with Gd-EOB-DTPA to detect small hepatocellular carcinoma. AJR Am J Roentgenol. 2012; 198:106–114.
38. Rajesh S, Mukund A, Arora A, Jain D, Sarin SK. Contrast-enhanced US-guided radiofrequency ablation of hepatocellular carcinoma. J Vasc Interv Radiol. 2013; 24:1235–1240.
39. Kim AY, Lee MW, Rhim H, Cha DI, Choi D, Kim YS, et al. Pretreatment evaluation with contrast-enhanced ultrasonography for percutaneous radiofrequency ablation of hepatocellular carcinomas with poor conspicuity on conventional ultrasonography. Korean J Radiol. 2013; 14:754–763.
40. Lu DS, Yu NC, Raman SS, Limanond P, Lassman C, Murray K, et al. Radiofrequency ablation of hepatocellular carcinoma: treatment success as defined by histologic examination of the explanted liver. Radiology. 2005; 234:954–960.
41. Kang TW, Lim HK, Lee MW, Kim YS, Choi D, Rhim H. Perivascular versus nonperivascular small HCC treated with percutaneous RF ablation: retrospective comparison of long-term therapeutic outcomes. Radiology. 2014; 270:888–899.
42. Kim JH, Won HJ, Shin YM, Kim SH, Yoon HK, Sung KB, et al. Medium-sized (3.1-5.0 cm) hepatocellular carcinoma: transarterial chemoembolization plus radiofrequency ablation versus radiofrequency ablation alone. Ann Surg Oncol. 2011; 18:1624–1629.
43. Yoon JH, Lee JM, Hwang EJ, Hwang IP, Baek J, Han JK, et al. Monopolar radiofrequency ablation using a dual-switching system and a separable clustered electrode: evaluation of the in vivo efficiency. Korean J Radiol. 2014; 15:235–244.
44. Yoon JH, Lee JM, Han JK, Choi BI. Dual switching monopolar radiofrequency ablation using a separable clustered electrode: comparison with consecutive and switching monopolar modes in ex vivo bovine livers. Korean J Radiol. 2013; 14:403–411.
45. Lee ES, Lee JM, Kim KW, Lee IJ, Han JK, Choi BI. Evaluation of the in vivo efficiency and safety of hepatic radiofrequency ablation using a 15-G Octopus® in pig liver. Korean J Radiol. 2013; 14:194–201.
46. Woo S, Lee JM, Yoon JH, Joo I, Kim SH, Lee JY, et al. Small- and medium-sized hepatocellular carcinomas: monopolar radiofrequency ablation with a multiple-electrode switching system-mid-term results. Radiology. 2013; 268:589–600.