Abstract
Objective
To evaluate image quality of female pelvic computed tomography (CT) scans reconstructed with the adaptive statistical iterative reconstruction (ASIR) technique combined with low tube-voltage and to explore the feasibility of its clinical application.
Materials and Methods
Ninety-four patients were divided into two groups. The study group used 100 kVp, and images were reconstructed with 30%, 50%, 70%, and 90% ASIR. The control group used 120 kVp, and images were reconstructed with 30% ASIR. The noise index was 15 for the study group and 11 for the control group. The CT values and noise levels of different tissues were measured. The contrast to noise ratio (CNR) was calculated. A subjective evaluation was carried out by two experienced radiologists. The CT dose index volume (CTDIvol) was recorded.
Results
A 44.7% reduction in CTDIvol was observed in the study group (8.18 ± 3.58 mGy) compared with that in the control group (14.78 ± 6.15 mGy). No significant differences were observed in the tissue noise levels and CNR values between the 70% ASIR group and the control group (p = 0.068-1.000). The subjective scores indicated that visibility of small structures, diagnostic confidence, and the overall image quality score in the 70% ASIR group was the best, and were similar to those in the control group (1.87 vs. 1.79, 1.26 vs. 1.28, and 4.53 vs. 4.57; p = 0.122-0.585). No significant difference in diagnostic accuracy was detected between the study group and the control group (42/47 vs. 43/47, p = 1.000).
The growing application of computed tomography (CT) in clinical practice has raised concerns about the increasing incidence of cancer from radiation exposure. Therefore, how to reduce the radiation dose without compromising diagnostic imaging quality has received a great deal of attention (1234). Low dose CT scans have been mainly used for chest organs, which exhibit high physical contrast. Radiation dose is directly proportional to the square of the tube voltage (5); therefore, decreasing tube voltage more effectively reduces the radiation dose but inevitably increases the noise level. Thus, the innate limitation of the traditional filtered back projection (FBP) reconstruction algorithm hinders further reducing the radiation dose. Low tube-voltage scanning (67) has been used increasingly in clinical practice with the merging of iterative reconstruction techniques and automatic tube current modulation technology. The iterative image reconstruction algorithm significantly reduces noise (8910111213) and improves image quality. Abdominal and pelvic CT scans used to diagnose and treat diseases often require contrast enhancement using large range and multi-phase scans, which increase the radiation dose. Moreover, the female pelvic area contains reproductive organs that are particularly sensitive to radiation. Therefore, reducing the scan dose is particularly desirable. Most low-dose studies have focused on the abdominal area (7111415), and only a few studies have investigated the lower abdominal and pelvic areas. In this study, we used a lower tube-voltage pelvic scan combined with automatic tube current modulation and iterative reconstruction to explore the feasibility of a low-dose scan protocol to achieve acceptable diagnostic image quality on female pelvic scans.
A total of 101 patients who underwent contrast-enhanced gynecological abdominal/pelvic CT scan in outpatient and inpatient centers from February 2014 to August 2014 were recruited. These patients were Chinese-Han ethnicity from different domestic areas. Twenty-eight patients underwent hysterectomy and oophorectomy due to benign or malignant tumors; 19 patients were diagnosed with endometrial lesions; 28 patients were diagnosed with cervical lesions; 22 patients were diagnosed with adnexal tumors, and the other four patients were diagnosed with uterine malformations (two cases) and abdominal pain (two cases). The patients were 20-78 years old and were divided randomly into study and control groups according to evaluation date. Patients seen on the first day were designated the study group and patients seen the following day were designated as the control group. The group designation was alternated each day thereafter. All enrolled patients were adults, conscious, able to understand instructions, had no serious liver or kidney damage, and were not allergic to the contrast agent. Exclusion criteria included patients with a history of allergies, kidney dysfunction, and those < 18 years old. Five patients were excluded due to renal dysfunction or allergy to the contrast agent and two patients were excluded due to contrast extravasation during the injection process. Ninety-four cases were finally included in this study (n = 47/group). Height, weight, and body mass index (BMI) were recorded for both groups. All patients signed informed consent before enrollment in this study. This study was approved by the hospital ethics committee.
Scans were performed using a 64-row multidetector CT scanner (Discovery CT 750 HD, General Electric Corp., Milwaukee, WI, USA). All patients underwent conventional abdominal and pelvic scans in the supine position with a scan range from the top of the diaphragm to the inferior margin of the pubic symphysis. All scans were performed with a single breath-hold to minimize motion and misregistration artifacts. Automatic tube current modulation technology was applied, and the milliamperage range was adjusted to 50-600 mAs. The parameters used in the study group were: tube-voltage, 100 kVp; noise index (NI) = 15; image reconstruction with 30%, 50%, 70%, and 90% adaptive statistical iterative reconstruction (ASIR) (four image sets for each patient). The parameters used in the control group were: tube-voltage 120 kVp; NI = 11; image reconstruction with 30% ASIR (one image set for each patient). Other scanning parameters were applied as follows: gantry rotation time, 0.8 seconds; pitch, 0.984; collimation, 0.625 × 64 mm; reconstruction slice thickness, 5 mm. Ioversol (Jiangsu Hengrui Medicine, Co., Jiangsu, China) (320 mgI/mL, 100 mL) was injected with a high-pressure syringe via a peripheral vein at a flow rate of 3 mL/s. The bolus-tracking technique was used to monitor the scans. The arterial phase scan was started 10 seconds after the contrast enhancement threshold (150 Hounsfield units [HU]) was reached within the region of interest (ROI; lumen of the abdominal aorta at the level of the renal hilus). The venal phase scan was started 40 seconds after completing the arterial phase scan.
All CT image data were viewed and evaluated using a GE ADW4.6 workstation with unified window width and window level (window width, 400 HU; window level, 40 HU). All images from the two groups (Fig. 1) were evaluated randomly. The related imaging information in the display tools of workstation user interface remained anonymous. The CT value and the standard deviation (SD, interpreted as noise level) of the bladder were measured during the unenhanced bladder scan. The CT values and the SD of the iliac muscle, obturator muscle, abdominal fat tissue, and the uterus were measured during the venal phase. These measurements were performed at the homogeneous and maximum area levels of the bladder, iliac muscle, and the uterine and obturator muscles (Fig. 2). The contrast to noise ratio (CNR) was calculated as: CNR = (tissue CT value - CT value of the abdominal subcutaneous fat tissue at the same level) / fat noise. The CT value ROI was 50-200 mm2 (bladder, 190-200; iliac muscle, obturator muscle, and fat, 50-70; and uterus, 60-80 mm2). Three independent measurements were performed and averaged for each parameter. The location and size of the measurements were identical for all patients.
The CT images were subjectively scored by two experienced radiologists who were blinded to the reconstruction algorithms using recommended CT image quality standards described in previous studies (1617) and recommended by the European guidelines (18). Image quality was assessed on a 5-point scale: 1 point, unqualified, did not meet the diagnostic requirements; 2 points, poor, did not meet the diagnostic requirements; 3 points, acceptable, met the diagnostic requirements; 4 points, good, met the diagnostic requirements; 5 points, excellent, met the diagnostic requirements. Images scored ≥ 3 points were considered clinically acceptable. The specific evaluation criteria were: 1) Subjective image noise was assessed on a 5-point scale: 1, minimal; 2, less than average; 3, average; 4, above average; and 5, unacceptable. 2) Artifacts were graded on a 4-point scale: 1, no artifacts; 2, minor artifacts not interfering with diagnostic decision making; 3, major artifacts affecting visualization of major structures, diagnosis still possible; and 4, artifacts affecting diagnostic information. The types of artifacts were: helical artifacts; streak artifacts caused by metals; beam-hardening artifacts caused by the arms being at the side of body or large body size; truncation artifacts due to large body size or off-centering; and ASIR artifacts (blocky pixelated appearance or over-smooth appearance). 3) Visibility of small structures (i.e., small lymph nodes near the uterus, small blood vessels, etc.) was ranked on a 5-point scale: 1, excellent; 2, above average; 3, acceptable; 4, suboptimal; and 5, unacceptable. 4) Overall diagnostic confidence was assessed and ranked using a 4-point scale: 1, completely confident; 2, probably confident; 3, confident only for a limited clinical situation; and 4, nondiagnostic examination.
The radiologists also made diagnoses while they were evaluating the images. The two radiologists summarized their results and gave a final diagnosis after the subjective scoring. If there were disagreements between the two readers, a third radiologist adjudicated the disagreement. Diagnostic accuracy (number of patients with accurate diagnosis/total number of patients) was calculated. The diagnostic standard was referred to the patient's pathology and medical history.
The statistical analysis was performed using SPSS 19.0 software (SPSS Inc., Chicago, IL, USA). Data are expressed as mean ± standard deviation. One-way analysis of variance and the Bonferroni correction were used to analyze different tissues noise levels and CNR. The independent sample t test was used to compare basic information (height, weight, and BMI) of patients. Diagnostic accuracy of the two groups was compared with the chi-square test. The Kruskal-Wallis test was used to analyze differences in the subjective scores. The linear-weighted Kappa test was used to measure consistency of the subjective scores between the observers. Interobserver agreement based on the k values was classified as: ≥ 0.8, almost perfect agreement; 0.61-0.8, good consistency; 0.41-0.6, moderate consistency; 0.21-0.4, fair agreement; and < 0.2, inconsistent.
No significant differences were observed in height, age, weight, or BMI between the two patient groups (p = 0.271-0.911) (Table 1), indicating the comparability of the data. A 44.7% decrease in the CT dose index volume (CTDIvol) value was observed in the study group (8.18 ± 3.58 mGy), compared to that in the control group (14.78 ± 6.15 mGy).
The CT values and the noise levels of the bladder, uterine, iliac, and obturator muscles, as well as abdominal fat were measured, and the CNRs were calculated (Table 2). Fifteen patients in the study group underwent a hysterectomy; therefore, 32 uterine measurements were made in the study group. Thirteen patients in the control group underwent a hysterectomy, and two were excluded due to interference by a intrauterine device; therefore, 32 uterine measurements were made in the control group. Noise levels in different tissues decreased and the CNR increased in proportion with the increase in ASIR in the study group (Figs. 3, 4). The differences were statistically significant. The tissue noise level in the 70% ASIR study group was similar to that in the control group (p = 1.000); however, the tissue noise levels in the other ASIR groups were significantly different from that in the control group. The CNR values of the 50% and 70% ASIR groups in the study group were similar to that in the control group (iliac muscle, p = 0.136-0.928; bladder, p = 0.210-0.961; obturator muscle, p = 0.068-0.102; uterus, p = 0.114-1.000). The CNR value in the control group was between that in the 50% ASIR and 70% ASIR groups. Therefore, the noise level of the 70% ASIR group was equivalent to that of the control group, but the CNR was better in the 70% ASIR group.
The subjective image quality score results (Table 3) indicated that all images reached the clinical diagnostic requirement and the image quality scores were ≥ 3 points. The 70% ASIR study group had the highest score, which was similar to that in the control group (4.53 vs. 4.57; p = 0.572). The 50% ASIR and 90% ASIR groups had similar scores (p = 0.302). The image quality score was significantly lower in the 30% ASIR group than those in the other groups (p < 0.001). Image noise scores decreased in proportion to the increase in the percentage ASIR. No statistical difference was observed between the 70% ASIR and control groups, whereas the other groups were significantly different. The diagnostic confidence scores were similar between the study and control groups (1.26-1.34 points, p = 0.193-0.840). No significant differences in the visibility scores for the small structures were observed between the 50% ASIR, 70% ASIR, and control groups (p = 0.047-0.651). No significant difference was observed between the 30% ASIR and 90% ASIR groups. The 70% ASIR group had nine patients (19.1%) and the 90% ASIR group had 30 patients (63.8%) who showed an over-smooth or blotchy pixilated appearance in the image artifact score evaluation. The image artifacts score was significantly higher in the 90% ASIR group than those in the other groups (p < 0.001), but no other differences were detected in the other groups. No significant difference in diagnostic accuracy was detected between the study group (42/47) and the control group (43/47) (p = 1.000). Diagnostic accuracy (42/47) was equal among the 30% ASIR, 50% ASIR, 70% ASIR, and 90% ASIR groups in the study group.
The scores between the two observers were consistent (kappa = 0.515-0.775).
The International Commission on Radiation Protection has suggested that CT scans must follow the As Low As Reasonably Achievable principle of optimization. CT scanning protocols should keep the radiation dose at the lowest achievable level while satisfying the clinical CT image quality diagnostic requirements. Our results showed a 44.7% radiation dose reduction in the study group (8.18 mGy), compared with that in the control group (14.78 mGy). The comparisons of objective noise, CNR, and the subjective evaluation between the ASIR groups and the control group indicated that the image quality of 70% ASIR group was similar to that of the control group (p = 0.068-1.000). We applied automatic current modulation technology during our low-dose CT scans to prevent increases in the radiation dose by increasing the default NI to control tube current and to prevent an excess increase in the tube current caused by a drop in tube voltage (15). Image contrast increased during enhanced scanning using iodine-containing contrast agent when tube voltage was decreased from the conventional 120 kVp to 100 kVp. The CT attenuation value of the iodine contrast agent significantly increased (1920) and the CT values of the enhanced tissue improved. We applied high proportion ASIR (70% ASIR) to reduce the noise level and ensure adequate image quality to meet clinical requirements. The control group used 120 kVp and 30% ASIR, which are routine in our department, so the control group radiation dose was lower than that of other studies using the conventional FBP reconstruction algorithm (14.78 mGy vs. 19.5-20.79 mGy) (2122); therefore, the reduced radiation dose in our study groups was higher than 44.7%, when compared with the dose of other studies using the conventional FBP reconstruction algorithm. Sagara et al. (15) showed that applying the ASIR algorithm decreased the radiation dose by 23-66% in 53 patients who underwent enhanced abdominal CT scans. Gervaise et al. (21) reported that using adaptive iterative dose reduction three-dimensional decreased the radiation dose 49.5% on abdominal and pelvic scans, compared with that of the FBP image reconstruction algorithm. However, the reductions in radiation dose may not be comparable between studies, as different research groups use different scanning parameters and iterative reconstruction types.
Iterative reconstruction is the mainstream method for image reconstruction. Previous studies applied 20-40% ASIR for abdominal CT scans (1115232425). It is generally assumed that a high proportion of ASIR results in a blotchy pixilated or over-smoothing appearance of images (242627). However, the ASIR proportion needs to be increased to meet the clinical diagnostic image quality requirements for low-dose scans (1324). Singh et al. (24) compared abdominal CT images of 22 patients and found that when mA was decreased to 50 (120 kVp), the ASIR proportion must be increased to 70% to obtain clinically acceptable image quality. However, four of the 22 images (18.2%) showed an obvious blotchy pixilated appearance with 70% ASIR. In our study group, nine of the 47 images (19.1%) showed an obvious blotchy pixilated or over smooth appearance in the 70% ASIR group; however, it did not interfere with the diagnostic accuracy. The blotchy pixilated or over smooth appearance became more obvious (30/47 images, 63.8%) when the ASIR proportion was increased to 90%, and the subjective scores and diagnostic confidence of the images declined.
Our study had several limitations. The majority of the patients had medium BMIs and only 9.6% of the patients were obese, which may have been due to the small sample size and the Asian ethnicity of the patients. We will increase our sample size in our future study.
We only recruited female patients who underwent contrast-enhanced scans for postoperative follow-up of benign and malignant tumors or for a comprehensive preoperative assessment. The scan range included the upper abdomen; therefore, the CTDIvol represented both the abdominal and pelvic dose. In this case, the pelvic radiation dose assessment may not have been accurate. We found that the mA used for the abdominal scan was significantly lower than the mA used for the pelvic scan, suggesting that the radiation dose to the pelvis could have been higher than measured. Because the patients in the two groups were different, we only evaluated the diagnostic confidence for lesions and compared overall diagnostic accuracy, but we did not evaluate lesion details. In addition, further reducing tube voltage would produce more noise, so we chose a relatively conservative condition (100 kVp). Some studies have indicated that 80 kVp can be used for abdominal scans (202328). Thus, we will further decrease tube voltage in our future study.
In summary, our findings suggest that lowering tube voltage to 100 kVp and using higher ASIR levels than typically reported can provide acceptable diagnostic imaging quality when imaging the female pelvis using enhanced CT.
Figures and Tables
Fig. 1
Images reconstructed with different proportions of ASIR and kVp.
A-E are reconstructed images using 30% ASIR (100 kVp), 50% ASIR (100 kVp), 70% ASIR (100 kVp), 90% ASIR (100 kVp), and 30% ASIR (120 kVp), respectively. Image scores for these five sets of images were > 3 points. Noise level in D was lower than that in A. Noise level in E was similar to those in C and D. A-D were from same patient with BMI of 23.2 kg/m2 and CTDIvol of 6.35 mGy. E was from another patient with BMI of 22.8 kg/m2 and CTDIvol of 13.3 mGy. ASIR = adaptive statistical iterative reconstruction, BMI = body mass index, CTDIvol = computed tomography dose index volume
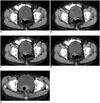
Fig. 2
Locations of ROIs in different tissues.
A. Image shows locations of ROI in bladder. B. Image shows locations of ROIs in iliac and fat. C. Image shows locations of ROI in uterine. D. Image shows locations of ROI in obturator muscles. ROI = region of interest
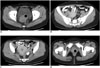
Fig. 3
Noise level at different ASIR proportions and over smooth appearance on 70% and 90% ASIR images.
A-D are 30-90% ASIR images from same patient. Noise level decreased in proportion to increases in ASIR. C and D show over smooth appearance. ASIR = adaptive statistical iterative reconstruction
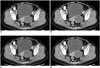
Fig. 4
Noise level at different ASIR proportions; over smooth appearance on 90% ASIR image.
A-D are 30-90% ASIR images from same patient. Noise level decreased in proportion to increases in ASIR. D shows over smooth appearance. ASIR = adaptive statistical iterative reconstruction
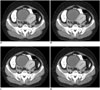
Table 1
Basic Information and Scanning Dose from Study Groups and Control Group

Table 2
Noise Level and CNR of Bladder, Uterus, Iliac Muscle, and Internal Obturator Muscle and Fat Noise of Same Level of Different Tissue from Study Groups and Control Group
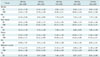
Table 3
Image Quality Scores from Study Groups and Control Group

References
1. Chen JH, Jin EH, He W, Zhao LQ. Combining automatic tube current modulation with adaptive statistical iterative reconstruction for low-dose chest CT screening. PLoS One. 2014; 9:e92414.
2. Shin HJ, Chung YE, Lee YH, Choi JY, Park MS, Kim MJ, et al. Radiation dose reduction via sinogram affirmed iterative reconstruction and automatic tube voltage modulation (CARE kV) in abdominal CT. Korean J Radiol. 2013; 14:886–893.
3. Pickhardt PJ, Lubner MG, Kim DH, Tang J, Ruma JA, del Rio AM, et al. Abdominal CT with model-based iterative reconstruction (MBIR): initial results of a prospective trial comparing ultralow-dose with standard-dose imaging. AJR Am J Roentgenol. 2012; 199:1266–1274.
4. McCollough CH, Primak AN, Braun N, Kofler J, Yu L, Christner J. Strategies for reducing radiation dose in CT. Radiol Clin North Am. 2009; 47:27–40.
5. Kalra MK, Maher MM, Toth TL, Hamberg LM, Blake MA, Shepard JA, et al. Strategies for CT radiation dose optimization. Radiology. 2004; 230:619–628.
6. Marin D, Nelson RC, Schindera ST, Richard S, Youngblood RS, Yoshizumi TT, et al. Low-tube-voltage, high-tube-current multidetector abdominal CT: improved image quality and decreased radiation dose with adaptive statistical iterative reconstruction algorithm--initial clinical experience. Radiology. 2010; 254:145–153.
7. Yu MH, Lee JM, Yoon JH, Baek JH, Han JK, Choi BI, et al. Low tube voltage intermediate tube current liver MDCT: sinogram-affirmed iterative reconstruction algorithm for detection of hypervascular hepatocellular carcinoma. AJR Am J Roentgenol. 2013; 201:23–32.
8. Hara AK, Paden RG, Silva AC, Kujak JL, Lawder HJ, Pavlicek W. Iterative reconstruction technique for reducing body radiation dose at CT: feasibility study. AJR Am J Roentgenol. 2009; 193:764–771.
9. Martinsen AC, Sæther HK, Hol PK, Olsen DR, Skaane P. Iterative reconstruction reduces abdominal CT dose. Eur J Radiol. 2012; 81:1483–1487.
10. Prakash P, Kalra MK, Digumarthy SR, Hsieh J, Pien H, Singh S, et al. Radiation dose reduction with chest computed tomography using adaptive statistical iterative reconstruction technique: initial experience. J Comput Assist Tomogr. 2010; 34:40–45.
11. Prakash P, Kalra MK, Kambadakone AK, Pien H, Hsieh J, Blake MA, et al. Reducing abdominal CT radiation dose with adaptive statistical iterative reconstruction technique. Invest Radiol. 2010; 45:202–210.
12. Silva AC, Lawder HJ, Hara A, Kujak J, Pavlicek W. Innovations in CT dose reduction strategy: application of the adaptive statistical iterative reconstruction algorithm. AJR Am J Roentgenol. 2010; 194:191–199.
13. Singh S, Kalra MK, Gilman MD, Hsieh J, Pien HH, Digumarthy SR, et al. Adaptive statistical iterative reconstruction technique for radiation dose reduction in chest CT: a pilot study. Radiology. 2011; 259:565–573.
14. Lin XZ, Machida H, Tanaka I, Fukui R, Ueno E, Chen KM, et al. CT of the pancreas: comparison of image quality and pancreatic duct depiction among model-based iterative, adaptive statistical iterative, and filtered back projection reconstruction techniques. Abdom Imaging. 2014; 39:497–505.
15. Sagara Y, Hara AK, Pavlicek W, Silva AC, Paden RG, Wu Q. Abdominal CT: comparison of low-dose CT with adaptive statistical iterative reconstruction and routine-dose CT with filtered back projection in 53 patients. AJR Am J Roentgenol. 2010; 195:713–719.
16. Kalra MK, Prasad S, Saini S, Blake MA, Varghese J, Halpern EF, et al. Clinical comparison of standard-dose and 50% reduced-dose abdominal CT: effect on image quality. AJR Am J Roentgenol. 2002; 179:1101–1106.
17. Singh S, Kalra MK, Moore MA, Shailam R, Liu B, Toth TL, et al. Dose reduction and compliance with pediatric CT protocols adapted to patient size, clinical indication, and number of prior studies. Radiology. 2009; 252:200–208.
18. EUR 16262. European guidelines on quality criteria for computed tomography. http://www.drs.dk/guidelines/ct/quality/download/eur16262.w51.
19. Kondo H, Kanematsu M, Goshima S, Tomita Y, Kim MJ, Moriyama N, et al. Body size indexes for optimizing iodine dose for aortic and hepatic enhancement at multidetector CT: comparison of total body weight, lean body weight, and blood volume. Radiology. 2010; 254:163–169.
20. Schindera ST, Diedrichsen L, Müller HC, Rusch O, Marin D, Schmidt B, et al. Iterative reconstruction algorithm for abdominal multidetector CT at different tube voltages: assessment of diagnostic accuracy, image quality, and radiation dose in a phantom study. Radiology. 2011; 260:454–462.
21. Gervaise A, Osemont B, Louis M, Lecocq S, Teixeira P, Blum A. Standard dose versus low-dose abdominal and pelvic CT: comparison between filtered back projection versus adaptive iterative dose reduction 3D. Diagn Interv Imaging. 2014; 95:47–53.
22. Kaza RK, Platt JF, Al-Hawary MM, Wasnik A, Liu PS, Pandya A. CT enterography at 80 kVp with adaptive statistical iterative reconstruction versus at 120 kVp with standard reconstruction: image quality, diagnostic adequacy, and dose reduction. AJR Am J Roentgenol. 2012; 198:1084–1092.
23. Vardhanabhuti V, Riordan RD, Mitchell GR, Hyde C, Roobottom CA. Image comparative assessment using iterative reconstructions: clinical comparison of low-dose abdominal/pelvic computed tomography between adaptive statistical, model-based iterative reconstructions and traditional filtered back projection in 65 patients. Invest Radiol. 2014; 49:209–216.
24. Singh S, Kalra MK, Hsieh J, Licato PE, Do S, Pien HH, et al. Abdominal CT: comparison of adaptive statistical iterative and filtered back projection reconstruction techniques. Radiology. 2010; 257:373–383.
25. Flicek KT, Hara AK, Silva AC, Wu Q, Peter MB, Johnson CD. Reducing the radiation dose for CT colonography using adaptive statistical iterative reconstruction: a pilot study. AJR Am J Roentgenol. 2010; 195:126–131.
26. Thibault JB, Sauer KD, Bouman CA, Hsieh J. A three-dimensional statistical approach to improved image quality for multislice helical CT. Med Phys. 2007; 34:4526–4544.
27. Xu J, Mahesh M, Tsui BM. Is iterative reconstruction ready for MDCT? J Am Coll Radiol. 2009; 6:274–276.
28. Tang K, Wang L, Li R, Lin J, Zheng X, Cao G. Effect of low tube voltage on image quality, radiation dose, and low-contrast detectability at abdominal multidetector CT: phantom study. J Biomed Biotechnol. 2012; 2012:130169.