Abstract
Objective
Materials and Methods
Results
Figures and Tables
Fig. 1
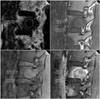
Fig. 2
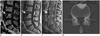
Fig. 3
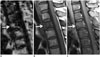
Fig. 4
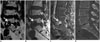
Fig. 5
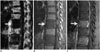
Table 1
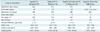
Table 2
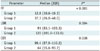
Note.- *Statistical significance in difference of median values between group 1 and group 2 for each parameter. Group 1 = malignant marrow-replacing lesion, Group 2 = benign red marrow deposition. CER = contrast-enhancement ratio, FF = fat-signal fraction, IQR = interquartile range, LDR = lesion-disc ratio
Table 3

Note.- Numbers in parentheses in AUC column are 95% confidence intervals. Numbers in parenthesis in sensitivity and specificity columns are counts used for calculation and 95% confidence intervals. *Lesions were considered malignant when FF was 16.8% or less, LDR was 114.8% or less, and CER was 93.7% or greater, †P value of AUC. AUC = area under receiver operating characteristic curve, CER = contrast-enhancement ratio, FF = fat-signal fraction, LDR = lesion-disc ratio
Table 4
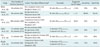
Study | Total Number of Patients (Lesions)* | Lesions That Were Differentiated† | Parameter | Suggested Cut-Off Value‡ | Sensitivity | Specificity |
---|---|---|---|---|---|---|
Disler et al. (9) | 30 patients (31) |
Non-neoplastic lesion (14) Neoplastic lesion (17) |
SI ratio (SIopposed-phase/SIin-phase) | > 0.81 | 95% | 95% |
Zampa et al. (7) | 86 patients (86) |
Benign lesion (41) Malignant lesion (45) |
SI ratio (SIopposed-phase/SIT1WI) | > 1.2 | 88.8% | 80.4% |
Eito et al. (21) | 108 patients (190) |
Normal vertebrae (90) Compression fracture (100) Non-neoplastic (73) Neoplastic (27) |
SI ratio (SIopposed-phase/SIin-phase) | None§ | N/A | N/A |
Zajick et al. (4) |
75 patients (569) 92 patients (215) |
Normal vertebrae (569) Benign lesion (164) Malignant lesion (51) |
% decrease of SIopposed-phase compared with SIin-phase | ≤ 20% | N/A | N/A |
Erly et al. (6) | 21 patients (49) |
Benign compression fracture (29) Malignant lesion (20) |
SI ratio (SIopposed-phase/SIin-phase) | > 0.8 | 95% | 89% |
Ragab et al. (5) | 40 patients (40) |
Compression fracture (40) Osteoporotic (20) Neoplastic (20) |
% decrease of SIopposed-phase compared with SIin-phase | ≤ 35% | 95% | 100% |
Note.- *Numbers in parentheses in this column are counts of lesions, †Normal, benign, and malignant lesions that were described and assessed. Numbers in parentheses of this column are counts of lesions, ‡Lesions were defined as malignant when suggested cut-off value was satisfied for each parameter, §Eito et al. calculated mean value of each lesion group, but did not estimate cut-off value for differentiating between groups. N/A = not available, SI = signal intensity, SIin-phase = signal intensity of in-phase image, SIopposed-phase = signal intensity of opposed-phase image, SIT1WI = signal intensity of T1-weighted image