Abstract
Objective
To compare the effect of imaging time delay on the MR detection of intracerebral metastases using single dose gadobutrol.
Materials and Methods
Twenty-one patients with intracerebral metastases underwent contrast-enhanced MR with three-dimensional T1-weighted sequence at 1 minute, 5 minutes and 10 minutes after a single dose injection of gadobutrol. One hundred index metastatic lesions (1 to 30 mm; median, 7 mm) were chosen for the analysis. For the qualitative analysis, lesion conspicuity were assessed on a 1 (worst) to 5 (best) scale of the index lesions by an expert reader. For the quantitative analysis, signal intensity (SI) of enhancing lesions and normal parenchyma was measured to determine the contrast rate (CR, %) ([postcontrast SI lesion - postcontrast SI white matter] × 100 / postcontrast SI white matter) and the enhancement rate (ER, %) ([postcontrast SI lesion - baseline SI gray matter] × 100 / baseline SI gray matter). Statistical comparisons were made between three different time delays.
Results
Lesion conspicuity did not differ significantly among the three time delays (p = 0.097). Although the SI, CR and ER of lesions did not reveal any significant difference between 1 minute and 5 minutes delayed images, both the 1 minute and 5 minutes delayed images showed significantly higher CRs of lesions compared with the 10 minutes delayed images (p = 0.004 and p = 0.001, respectively).
Magnetic resonance imaging (MRI) is the modality of choice for the diagnosis of central nervous system diseases. In particular, enhanced MRI with gadolinium-based contrast agent (GBCA) is essential for the detection and follow-up of intracerebral metastases. GBCAs increase signal intensity (SI) on T1-weighted images by shortening the T1 relaxation time. T1 shortening depends on the intrinsic relaxivity of the agent and its concentration in the region of interest.
Gadobutrol, the first 1.0 mol/L (1 M) gadolinium chelate agent, has recently been introduced in clinical practice. Gadobutrol has inherently higher T1 relaxivity (the efficiency of a contrast agent to reduce the T1 relaxation time, L/mM/s) than gadopentetate dimeglumine (Gd-DTPA). Therefore, the standard dose (0.1 mmol/kg body weight) of gadobutrol can cause superior contrast enhancement compared to the standard dose of Gd-DTPA (1, 2).
Optimizing imaging time delay between contrast injection and imaging sequence can be an important issue to improve the diagnostic sensitivity of contrast enhanced MRI for brain metastases. A few earlier studies reported that the lesion contrast of central nervous system lesions increases with delayed imaging time (3-5). Accordingly, contrast-enhanced sequences of brain lesions are usually obtained at an average of 5 minutes after contrast injection up to 10 minutes after contrast injection (6). However, more recent studies using dynamic contrast-enhanced T1-weighted perfusion imaging have suggested that the shape of the time-SI curve of a brain tumor is not that of steady enhancement but rather that of enhancement with a plateau or enhancement with a later washout (7, 8). Based on this, we can hypothesize that delayed imaging time beyond a certain time point does not provide better enhancement of brain metastases.
With respect to the 1 M gadolinium contrast agent gadobutrol there has been no research demonstrating the effect of delayed enhanced MRI in the clinical setting of patients with primary brain tumor or metastasis. Hence, the purpose of this study was to compare the effect of MRI at 3T on lesion contrast and lesion conspicuity at different imaging times using gadobutrol in patients with intracerebral metastases.
The institutional review board approved this retrospective study, and the requirement of informed consent was waived.
Between March 2011 and June 2011, 87 consecutive patients over 18 years of age with a clinical suspicion of intracerebral metastasis or those who were screened for metastases underwent contrast enhanced MRI. Among them, only those who had contrast enhancing lesions and had undergone follow-up imaging study or surgical biopsy of the lesion in order to confirm the diagnosis of metastases were included. Among 30 patients with intracerebral metastases who met the inclusion criteria, 9 patients who had received radiotherapy or GKS for brain metastases before undergoing MRI were excluded from the study. Finally, 21 patients with intracerebral metastases (12 men and 9 women; mean age, 60.5 years; age range, 28-85 years) confirmed by imaging follow-up (n = 16) or pathological examination (n = 5) were included in the analysis. The primary cancer sites in these patients were as follows: lungs (n = 14), breast (n = 3), colon (n = 1), liver (n = 1), stomach (n = 1), and uterus (n = 1).
All examinations were performed with a 3T scanner (SignaHDxt; GE Medical System, Milwaukee, WI, USA) with an eight-channel head coil. The MRI protocol included the following sequences: 1) the axial T1-weighted inversion recovery sequences (repetition time [TR]/echo time [TE]/inversion time [TI], 2468/12/920 ms; field of view [FOV], 220 mm; section thickness [ST]/intersection gap [IG], 5/2 mm; matrix, 512 × 224), 2) the axial T2-weighted FSE sequence (TR/effective TE, 4000/106 ms; FOV, 220 mm; ST/IG, 5/2 mm; matrix, 384 × 384), 3) the axial fluid attenuated inversion recovery (TR/TE/TI, 11000/105/2600 ms; FOV, 220 mm; ST/IG, 5/2 mm; matrix, 384 × 224), 4) the axial T2*-weighted gradient-echo sequence (TR/TE, 550/17 ms; FOV, 220 mm; ST/IG, 5/2 mm; matrix, 384 × 224; flip angle, 17°), and 5) the T1-weighted three-dimensional (3D) fast spoiled gradient-echo (FSPGR) sequence (TR/TE, 6.2/2.6 ms; FOV, 220 mm; ST/IG, 1/0 mm; matrix, 512 × 512).
Scanning with contrast-enhanced 3D-FSPGR was started at 1 minute, 5 minutes, and 10 minutes after the injection of a standard dose of gadobutrol (Gadovist; Schering, Berlin, Germany; 0.1 mmol/kg of body weight) through the antecubital vein using a power injector. All injections were followed by a saline flush of up to 30 mL.
Index lesions to be investigated in this study were first chosen by a consensus review between two neuroradiologists (5-year and 12-year experience) who were blinded to the time delay of contrast-enhanced MR sequence and clinical information the patients. All images acquired at three different time points of contrast-enhanced MR sequences in each patient were displayed simultaneously on three LCD displays but in random order. An enhancing metastatic tumor was defined as a well-demarcated area of unequivocally increased SI as compared with normal-appearing white matter. We tried to detect pseudo-enhancement, enhancing vessels, and flow artifacts by evaluating unenhanced and contrast-enhanced images at the same time. Leptomeningeal enhancement was counted as one lesion. Each lesion was classified according to its maximum diameter in the axial imaging plane as less than 5 mm, 5 to 10 mm, and larger than 10 mm. When more than 5 lesions in each size category were found in one patient, only 5 lesions in each size category were chosen for the assessment of individual lesion conspicuity. Of a total number of 156 enhancing metastatic nodules identified in the 21 patients (65 nodules measuring less than 5 mm, 39 nodules measuring 5 to 10 mm, and 52 nodules measuring greater than 10 mm), 100 nodules were selected as index lesions for further investigation in this study (32 nodules measuring less than 5 mm, 35 nodules measuring 5 to 10 mm, and 33 nodules measuring greater than 10 mm). The mean number of index lesions in each patient was 4.3 ± 3.3 (range; 1 to 10, median; 4).
At two weeks after the initial reading session, one of the two readers (12-year experience), both of whom were blinded to the time delay of contrast-enhanced MR sequence and patient clinical information, performed a side-by-side comparison of three different sets of images in each patient with respect to the overall and individual lesion conspicuity for the 100 index lesions. Overall lesion conspicuity was evaluated for each sequence per patient. The reader rated lesion conspicuity on a 5-point scale (1: very poor visualization of the enhancing lesion, 2: poor visualization of the enhancing lesion, 3: acceptable visualization of the enhancing lesion, 4: satisfactory visualization of the enhancing lesion, and 5: excellent visualization of the enhancing lesion).
The same reader performed the quantitative measurements. SI measurements were recorded in ROIs positioned on 1) the enhancing metastatic lesion and 2) normal appearing white matter (typical areas of lesion-free brain parenchyma without strong enhancing structures or artifacts). In each patient, the largest enhancing lesion measuring over 5 mm in diameter was chosen for the ROI measurement. The minimum ROI was 20 mm2. Enhancing lesions measuring 5 mm or less in diameter were not included in the analysis because smaller ROI size results in less precise measurement of SI. Each reader chose the most suitable image for ROI placement in the 1 minute-delayed scan, and performed the ROI measurement to determine the SI of gray matter, the enhancing lesion and white matter, independently.
ROI placement was performed using PIVIEW software (INFINITT, Seoul, Korea), and the ROI in a 1 minute delayed image was copied and transferred to the 5 minutes and 10 minutes delayed images. Inclusion of vessels or other strongly contrasting structures or artifacts in the ROI was strictly avoided. The ROI was positioned over the entire area of the enhancing lesion in case of homogeneous lesions, or over the maximal enhancing area in case of inhomogeneous lesions. For extensive lesions with an irregular circumference, the ROI was positioned to include the largest possible extent of the lesion.
The ROI data were used to calculate the contrast rate (CR) and the enhancement rate (ER) of the lesion as detailed below (9):
To calculate the ER, we used the SI of the background gray matter obtained at 1 minute after contrast injection as baseline SI because precontrast 3D-FSPGR MR images were not available, and the background gray matter was considered to be least affected by contrast agents.
In addition, we did not calculate the signal-to-noise ratio or the contrast-to-noise ratio because it was practically impossible to precisely measure the background noise in MR images that were acquired with parallel imaging techniques (10).
Statistical analyses were performed using commercially available software (PASW Statistics version 17.0; SPSS, Chicago, IL, USA). Because the data did not follow a normal distribution, we used non-parametric statistical tests. The level of significance was defined at p < 0.05. The Friedman test was used to evaluate the differences in the lesion number, lesion conspicuity and SI of enhancing lesions among the three different time delays (1 minute, 5 minutes and 10 minutes delayed imaging groups after contrast injection). Comparison of individual lesion conspicuity between different time delays was also performed for subgroups of patients according to lesion size (< 5 mm, 5 to 10 mm, and > 10 mm). Differences were considered significant at p < 0.05. For post hoc pairwise multiple comparison, we used the Wilcoxon signed-rank test (1 minute versus 5 minutes delayed imaging groups, 5 minutes versus 10 minutes delayed imaging groups, and 1 minute versus 10 minutes delayed imaging groups). In this case, the Bonferroni-corrected significance level of p < 0.017 was used.
In 21 patients, the number of enhancing lesions on MR images obtained at 1 minute, 5 minutes, and 10 minutes after the injection of 1.0-mol/L gadobutrol which was 156, 156 and 155, respectively, without statistical significance (p = 0.368).
The per-patient grade of overall lesion conspicuity on enhanced MR images obtained at 1 minute (mean ± standard deviation [SD], 4.65 ± 0.573), 5 minutes (4.87 ± 0.458), and 10 minutes (4.70 ± 0.635) after the contrast injection also did not differ significantly (p = 0.097). The grade of individual lesion conspicuity of the 100 nodules chosen for individual lesion conspicuity analysis, however, was different among the 1 minute, 5 minutes, and 10 minutes delayed images (mean ± SD of 4.79 ± 0.498, 4.79 ± 0.518, and 4.71 ± 0.591, respectively; p = 0.027). Post hoc comparison showed that the 10 minutes delayed images had a lower grade of lesion conspicuity than that of the 5 minutes delayed images (p = 0.005); however, the grade of lesion conspicuity between the 1 minute and 5 minutes delayed images was not significantly different (p = 1.000) (Fig. 1). When 100 nodules were divided into three subgroups according to the size criteria, there was no significant difference in terms of lesion conspicuity between the three different time delays for any subgroup (< 5 mm nodules [n = 32], p = 0.180; 5 to 10 mm nodules [n = 35], p = 0.074; and > 10 mm nodules [n = 33], p = 1.000).
In order to calculate the CR and the ER of lesions, two patients were excluded due to unavailability of ROI measurements (n = 1, leptomeningeal metastasis; n = 1, small enhancing lesion less than 5 mm). Thus, 19 patients were included in the ROI data analysis. The SI, CR and ER of the lesions did not show any significant difference between 1 minute and 5 minutes delayed images (p = 0.379, p = 0.024 and p = 0.355, respectively). However, compared with the 10 minutes delayed images, the 1 minute delayed images showed a significantly higher CR of the lesions (p = 0.004), and the 5 minutes delayed images showed a significantly higher SI, CR and ER of the lesions (p = 0.009, p = 0.001 and p = 0.005) (Table 1). Overall SI, CR and ER of lesions tended to decrease with increasing time delay.
In this study, we found that 1 minute and 5 minutes delayed images following gadobutrol injection were comparable in terms of contrast enhancement, but 10 minutes delayed images showed a significantly lower enhancement than the other delayed imaging groups although qualitative assessment was not significantly different between the three groups.
A recent intra-individual comparison study (11) showed superior power of gadobutrol in the detection of brain metastases. In addition, because of its unique stable cyclic structure, gadobutrol has been proven to be a relatively safe MR imaging contrast agent in patients with impaired renal function at doses of ≤ 0.3 mmol/L/kg of body weight (12).
While most research about contrast-enhanced MRI has been focused on the dosage and concentration of gadolinium agents, there have been a comparatively smaller number of studies focusing on 'imaging time' after contrast injection. Also, a few previous studies on brain tumors reported that the sensitivity of gadolinium-enhanced MRI could be improved by increasing the delay between contrast injection and scanning (3, 4). A study by Knopp et al. (13) showed that in patients with either gliomas or metastases, lesion enhancement with doses of 0.5 mol/L Gd-DTPA and gadobenate dimeglumine tended to increase with delayed imaging time as per increase in imaging delay. Using gadoteridol (Gd-HP-DO3A), 10 minutes delayed imaging after contrast injection caused improved detection of brain metastases that were smaller than 10 mm (14).
In contrast to these previous studies, we found that 10 minutes delayed images did not show improved lesion detection in patients with brain metastases. Furthermore, in a nodule-by-nodule comparison between the three sequences, there was no significant difference in individual conspicuity of brain metastases. Unlike our study, previous two studies were conducted with the use of Gd-DTPA and gadobenate dimeglumine. Thus, our results may be due to the fact that the contrast enhancement kinetics of gadobutrol which has a circular structure is slightly different from those of the two previously studies using GBCAs which have a linear structure. However, the exact kinetic mechanism to explain our results is unclear.
Our results may also be attributed to the fact that angiogenesis is a key element in tumor growth and metastasis. Angiogenic vessels in tumorous conditions have large gaps between endothelial cells, the endothelium, and basement membranes, as well as between the basement membrane and pericytes, thus making the vessels hyperpermeable to many macromolecules. MR contrast agents that leak slowly through normal vasculature are able to pass through tumor vessels more quickly to produce differential enhancement. This results in a fast "wash-in" of contrast coupled with a rapid "wash-out" (15, 16). Using the time-intensity curve, contrast enhancement patterns can be categorized into steady enhancement with time, enhancement with a plateau and enhancement with a washout (17). Recent dynamic contrast-enhanced perfusion studies indirectly suggested that the time-SI curve of viable tumors shows enhancement with a plateau or enhancement with a washout, but time-SI curve post-treatment necrosis shows steady enhancement with time (7, 8). In this respect, our study results are in agreement with these DCE T1-weighted perfusion imaging studies.
In the qualitative comparison of our study, there was no difference in individual conspicuity of brain metastases. The conventional wisdom from a limited number of previous studies was that a smaller enhancing nodule or metastasis could be easily diagnosed with delayed imaging as well as with the use of increased dose of the contrast agent (13, 14). A further study including a larger number of patients receiving gadobutrol may be necessary to confirm our results.
In the quantitative analysis of our study, we found that both 1 minute delayed images and 5 minutes delayed images showed a higher CR for enhancing nodules than 10 minutes delayed images. Although there was a problem of selection bias since we only included nodules that were 5 mm or larger in diameter in the quantitative measurement, our result was corroborated by a recent animal study using Fischer rats with superficially implanted gliomas (18). The study showed that lesion enhancement was maximal at 1 minute after gadobutrol injection, after which it showed a decreasing pattern (18).
Our study is the first to demonstrate the effect of imaging time delay after gadobutrol injection for the detection of brain metastases. In addition, our results may have some implications in terms of patient care and in the busy clinical setting of MR imaging centers. Long brain MRI examination may be uncomfortable, especially for elderly patients. Longer brain MRI acquisition time can also lead to poor imaging quality due to patient motion. In this respect, contrast-enhanced imaging with shorter imaging time delay may be beneficial for both patients and physicians.
There are some limitations to our study. First, the diagnosis of brain metastasis was based not on the pathologic report but on radiologic findings and clinical data. Therefore, we could not exclude the possibility of false positives, particularly, in the case of detection of one enhancing lesion. However, we tried to review follow-up MRI to exclude false-positive lesions. Second, we used only one enhancing lesion over 5 mm in diameter per patient for the ROI measurement. Therefore, our results obtained from the enhancement data may not represent the true characteristics of all metastatic nodules since other metastatic lesions were excluded. Third, the number of cases included in this study was not large enough to generalize our findings. Therefore, an additional prospective study with a larger number of subjects is warranted to verify these preliminary findings.
In conclusion, time delay does not affect the clinically relevant measures of the number of lesions detected or lesion conspicuity, and both 1-minute and 5-minute-delayed contrast-enhanced 3D-FSPGR sequences may be effective for detecting brain metastases. Thus, our findings suggest that a less time-consuming protocol be adopted using a short time delay contrast-enhanced image with good quality for the detection of brain metastases in elderly patients and in the busy clinical setting of many institutions.
Figures and Tables
Fig. 1
28-year-old woman displaying brain metastases from breast cancer.
Axial contrast-enhanced 3D-FSPGR images at 1 minute (A), 5 minutes (B), and 10 minutes (C) after injection of 1.0-mol/L gadobutrol show enhancing brain metastasis, which shows higher contrast enhancement in 1 minute and 5 minutes delayed images than in 10 minutes delayed images (arrows). This lesion in 10 minutes delayed image was missed in initial reading session. Larger enhancing mass shows good contrast enhancement at any time-point. 3D = three-dimensional, FSPGR = fast spoiled gradient echo
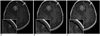
Table 1
Effects of Time Delay after Contrast Injection on MRI Enhancement Characteristics in 19 Patients with Intracerebral Metastases

Note.-*Values are expressed as mean ± standard deviation, †p = 0.009 for 5 minutes versus 10 minutes delayed imaging groups; p = 0.030 for 1 minute versus 10 minutes delayed imaging groups, ‡p = 0.001 for 5 minutes versus 10 minutes delayed imaging groups; p = 0.004 for 1 minute versus 10 minutes delayed imaging groups, §p = 0.005 for 5 minutes versus 10 minutes delayed imaging groups; p = 0.027 for 1 minute versus 10 minutes delayed imaging groups. SI = signal intensity
References
1. Attenberger UI, Runge VM, Jackson CB, Baumann S, Birkemeier K, Michaely HJ, et al. Comparative evaluation of lesion enhancement using 1 M gadobutrol vs. 2 conventional gadolinium chelates, all at a dose of 0.1 mmol/kg, in a rat brain tumor model at 3 T. Invest Radiol. 2009; 44:251–256.
2. Kim ES, Chang JH, Choi HS, Kim J, Lee SK. Diagnostic yield of double-dose gadobutrol in the detection of brain metastasis: intraindividual comparison with double-dose gadopentetate dimeglumine. AJNR Am J Neuroradiol. 2010; 31:1055–1058.
3. Healy ME, Hesselink JR, Press GA, Middleton MS. Increased detection of intracranial metastases with intravenous Gd-DTPA. Radiology. 1987; 165:619–624.
4. Byrne TN. Imaging of gliomas. Semin Oncol. 1994; 21:162–171.
5. Uysal E, Erturk SM, Yildirim H, Seleker F, Basak M. Sensitivity of immediate and delayed gadolinium-enhanced MRI after injection of 0.5 M and 1.0 M gadolinium chelates for detecting multiple sclerosis lesions. AJR Am J Roentgenol. 2007; 188:697–702.
6. Maravilla KR, Maldjian JA, Schmalfuss IM, Kuhn MJ, Bowen BC, Wippold FJ 2nd, et al. Contrast enhancement of central nervous system lesions: multicenter intraindividual crossover comparative study of two MR contrast agents. Radiology. 2006; 240:389–400.
7. Jacobs AH, Kracht LW, Gossmann A, Rüger MA, Thomas AV, Thiel A, et al. Imaging in neurooncology. NeuroRx. 2005; 2:333–347.
8. Suh CH, Kim HS, Choi YJ, Kim N, Kim SJ. Prediction of Pseudoprogression in Patients with Glioblastomas Using the Initial and Final Area Under the Curves Ratio Derived from Dynamic Contrast-Enhanced T1-Weighted Perfusion MR Imaging. AJNR Am J Neuroradiol. 2013; 34:2278–2286.
9. Furutani K, Harada M, Mawlan M, Nishitani H. Difference in enhancement between spin echo and 3-dimensional fast spoiled gradient recalled acquisition in steady state magnetic resonance imaging of brain metastasis at 3-T magnetic resonance imaging. J Comput Assist Tomogr. 2008; 32:313–319.
10. Moon WJ. Measurement of signal-to-noise ratio in MR imaging with sensitivity encoding. Radiology. 2007; 243:908–909.
11. Anzalone N, Gerevini S, Scotti R, Vezzulli P, Picozzi P. Detection of cerebral metastases on magnetic resonance imaging: intraindividual comparison of gadobutrol with gadopentetate dimeglumine. Acta Radiol. 2009; 50:933–940.
12. Benner T, Reimer P, Erb G, Schuierer G, Heiland S, Fischer C, et al. Cerebral MR perfusion imaging: first clinical application of a 1 M gadolinium chelate (Gadovist 1.0) in a double-blinded randomized dose-finding study. J Magn Reson Imaging. 2000; 12:371–380.
13. Knopp MV, Runge VM, Essig M, Hartman M, Jansen O, Kirchin MA, et al. Primary and secondary brain tumors at MR imaging: bicentric intraindividual crossover comparison of gadobenate dimeglumine and gadopentetate dimeglumine. Radiology. 2004; 230:55–64.
14. Yuh WT, Tali ET, Nguyen HD, Simonson TM, Mayr NA, Fisher DJ. The effect of contrast dose, imaging time, and lesion size in the MR detection of intracerebral metastasis. AJNR Am J Neuroradiol. 1995; 16:373–380.
15. Barrett T, Brechbiel M, Bernardo M, Choyke PL. MRI of tumor angiogenesis. J Magn Reson Imaging. 2007; 26:235–249.
16. Ocak I, Baluk P, Barrett T, McDonald DM, Choyke P. The biologic basis of in vivo angiogenesis imaging. Front Biosci. 2007; 12:3601–3616.
17. Kuhl CK, Mielcareck P, Klaschik S, Leutner C, Wardelmann E, Gieseke J, et al. Dynamic breast MR imaging: are signal intensity time course data useful for differential diagnosis of enhancing lesions? Radiology. 1999; 211:101–110.
18. Kramer H, Runge VM, Naul LG, Loynachan AT, Reiser MF, Wintersperger BJ. Brain MRI with single-dose (0.1 mmol/kg) Gadobutrol at 1.5 T and 3 T: comparison with 0.15 mmol/kg Gadoterate meglumine. AJR Am J Roentgenol. 2010; 194:1337–1342.