Abstract
Objective
To determine the optimum low dose (LD) digital tomosynthesis (DT) setting, and to compared the image quality of the LD DT with that of the standard default (SD) DT.
Materials and Methods
Nine DT settings, by changing tube voltage, copper filter, and dose ratio, were performed for determining the LD setting. Among combinations of DT setting, a condition providing the lowest radiation dose was determined. Eighty artificial nodules less than 1 cm in diameter (subcentimeter nodules: 40, micronodules less than 4 mm: 40) were attached to a Styrofoam and a diaphragm of the phantom. Among these, 38 nodules were located at the periphery of the lung (thin area) and 42 nodules were located at the paravertebral or sub-diaphragmatic area (thick area). Four observers counted the number of nodules detected in the thick and thin areas. The detection sensitivity in SD and LD settings were calculated separately. Data were analyzed statistically.
Results
The lowest LD setting was a combination of 100 kVp, 0.3 mm additional copper filter, and a 1 : 5 dose ratio. The effective dose for the LD and SD settings were 62 µSv and 140 µSv, separately. A 56.7% dose reduction was achieved in the LD setting compared with the SD setting. Detection sensitivities were not different between the SD and the LD settings except between observers 1 and 2 for the detection of micronodules in the thick area.
Digital tomosynthesis (DT) refers to the technique of collecting a number of projection radiographs at very low doses (LDs) and at varying angles, using these radiographs for reconstructing sections of the imaged object (1). These reconstructed section images contain fewer overlays of anatomical structures than the original radiographs. As a result, DT has the potential to increase the detectability of subtle nodules (2). The current standard for lung nodule detection and characterization is therapy with the use of computed tomography (CT), but CT is expensive compared to conventional radiography and can impart a significant radiation dose to the patient (3, 4). While there are numerous strategies for reducing radiation dose from CT (5), CT remains an increasing source of population radiation exposure (3). Recent reports have shown that the use of tomosynthesis, instead of conventional chest radiography, leads to considerable improvement in diagnostic information at only a modest increase in radiation dose (2). In this study, the effective dose imparted in tomosynthesis averaged 120 µSv, which is approximately 3 times that of a standard postero-anterior chest radiograph or a lateral radiograph chest exam. This was considerably lower than the 5 mSv commonly associated with CT of the chest. However, the radiation dose given to the patient should be optimized in order to follow the "As Low as Reasonably Achievable" principle (6). According to this principle, the necessary level of image quality for correct diagnosis in medical imaging should be obtained at the lowest possible radiation dose to the patient.
Thus, the purpose of our study was to determine the optimum LD DT setting by adjusting the user changeable parameters of DT, and to compare the image quality of the LD DT setting with that of the standard default (SD) DT setting.
Digital tomosynthesis was performed using a commercially available product (Volume RAD, GE healthcare, Chalfont St Giles, UK) with a commercial CsI/a-Si flat-panel detector system. Within a time period of about 11 s, the X-ray tube moved continuously at a vertical angle from -17.5° to 17.5°, around the standard, orthogonal-posteroanterior position. The detector position was fixed. In final, 60 projection images were acquired at the tube angle between -15° and +15°, and were used for reconstruction of approximately 54 coronal images with a thin thickness of 5 mm without overlap.
With the DT equipment used in this department, the user can adjust the ratio of the cumulative exposure dose of the tomosynthesis sweep to the exposure of the scout radiograph as a reference. The SD setting for chest DT was 120 kVp, automatic exposure control (AEC) speed of 400, no additional filter, and 1 : 10 dose ratio at 180 cm of source-to-image distance.
We used an anthropomorphic phantom (RANDO phantom, model RAN110, churchin associates, Smithtown, NY, USA), and radiophotolumininescent glass dosimeters (GD-352M, Asahi Techno Glass Corporation, Shizuoka, Japan) for the calculation of entrance surface dose (ESD).
Three radiophotoluminescent glass dosimeters were placed on the T6 level (both lungs, surface of vertebra). The attachment location of glass dosimeters was maintained for all measurements. The position of the prepared RANDO phantom was close to the front of detector with a posterior-anterior position.
We performed 9 DT scans, including SD setting, altering DT parameters, such as tube voltage, dose ratio, and filter (0.3 mm copper filter) (Table 1). Each scan was performed 10 times for minimizing a deviation of equipment and an error of measurement.
The dosimeters were transferred to a fully automatic readout system (FGD-1000, Asahi Techno Glass, Shizuoka, Japan), that was used to measure the absorbed radiation doses from the dosimeters. ESD was measured one time, and a final ESD was calculated by a tenth part of initial ESD. We obtained an each ESD for nine configurations. Among these, the condition that had lowest ESD value was chosen as the LD setting.
Effective doses and organ doses in SD and LD settings were calculated using Monte Carlo software (PCXMC, version 2.0; STUK, Helsinki, Finland).
To evaluate image quality, we used an anthropomorphic chest phantom (Alderson Lung/Chest Phantom RS-320; Radiology Support Devices, Long Beach, CA, USA, male skeleton, 175 cm, 73.5 kg).
We first removed the lung and diaphragm of the phantom. We then made 3 sets of artificial nodules embedded in Styrofoam. Each set consisted of 5 soaked adlay, red-bean, millet and un-soaked millet. Three sets of artificial nodules were inserted inside of the phantom (Fig. 1). We then attached an additional 20 artificial nodules (5 soaked adlay, red-bean, millet and un-soaked millet) on the pleural surface of the removed diaphragm to represent diaphragmatic and costo-phrenic nodules (subdiaphragmatic nodules). The total number of artificial nodules inserted in the phantom was 80. These were composed of 40 subcentimeter nodules (4-8 mm) and 40 micronodules (less than 4 mm). Among these, vertebral or paravertebral (right and left 2 cm from margin of vertebral body) nodules, and sub-diaphragmatic nodules were counted separately, and were classified as nodules in the 'thick area'. Other nodules were classified as nodules in the 'thin area'.
The Phantoms were then exposed using the LD and SD DT settings.
Four observers counted the number of subcentimeter nodules and micronodules detected in the thick and thin area.
One observer was a board-certified radiologist with 15 years of experience in chest radiology (observer 1). Two observers were board-certified radiologist with one-year of experience (observer 2 and 3). And final observer was a fourth-year radiology resident (observer 4).
In order to reduce bias, the observers independently evaluated the images in the same room without any time limitation. To avoid recall bias, DT images at the SD and LD settings were separately evaluated with a one week interval. LD DT was firstly evaluated.
We analyzed the numbers of detected nodules and the sensitivity according to area and size (subcentimeter nodules in the thick area and in the thin area, micronodules in the thick area and in the thin area) for the LD and SD settings. Data were analyzed using the McNemar test. We also counted false positive nodules in LD and SD setting for four observers. They were analyzed by chi-square test. A p value < 0.05 was considered significant.
Entrance surface dose measurements according to kVp, filter and dose ratio are shown in Table 1. The lowest DT dose was acquired at 100 kVp, a 1 : 5 dose ratio, and with the use of an additional Cu filter. And that setting was decided as LD setting.
Under the 1 : 10 dose ratio, the ESD decreased by 38%, 54%, and 42% at 120 kVp, 100 kVp, and 80 kVp, respectively, when we used an additional 0.3 mm Cu filter. With fixed use of an additional Cu filter, the ESD decreased by 37%, 46%, and 50% at 120 kVp, 100 kVp, and 80 kVp, respectively, when we changed the dose ratio from 1 : 10 to 1 : 5. A lower ESD was seen at 100 kVp than at 120 kVp or 80 kVp under the same AEC speed of 400.
In this study, the effective dose using the SD setting was 140 µSV, and the effective dose using the LD setting was 62 µSv. 56.7% dose reduction was noted using the LD setting compared with the SD setting. The estimated organ doses calculated by Monte Carlo simulation are compared in Figure 2. The organ dose to the lung was deceased by 57% noted using the LD setting compared with the SD setting (0.42 mGy in SD vs. 0.18 mGy in LD).
Mean numbers of detected nodules, detection sensitivities, and statistical significances for the 2 settings are summarized in Table 2.
The detection sensitivities for subcentimeter nodules in the thin area were similar: 95% using the SD setting and 97% using the LD setting. The sensitivities were also similar for subcentimeter nodule in thick area: 86% in the SD setting and 83% in the LD setting. Differences in the detection of subcentimeter nodules between the 2 settings were not statistically significant between observers.
The detection sensitivities of micronodules in the thin area were similar: 92% using the SD setting and 94% using the LD settings, and showed no statistical significance. However, for micronodules in the thick area, sensitivities were different: 63% using the SD setting and 44% using the LD setting, and showed statistical significances with observer 1 (p = 0.04) and observer 2 (p = 0.03).
Detected false positive nodules in SD and LD settings for four observers were noted in Table 3. There were no statistically significant differences in SD and LD settings for all observers.
As described above, DT is a newly available imaging modality that offers the potentially substantial improvements over conventional chest radiography for the detection of subtle lung disease (2, 7, 8). The major advantages of DT over conventional chest radiography are the removal of overlying structures, the enhancement of local tissue separation, and the availability of depth information for the structure of interest. Despite these advantages, the problem of high radiation dose remains. In published data, the estimated effective dose of DT ranged from 120 µSv to 200 µSv (2, 8-10), whereas the effective dose for a standard chest postero-anterior radiograph of a patient of the size of an average adult male was 17 µSv, and the dose for the left lateral view was 39 µSv (9). Other reports gave 40-50 µSv as the dose found on conventional two-view (PA and lateral view) chest radiographs. Thus the effective DT dose was 10 times higher than that of a standard chest postero-anterior radiograph, and 3-4 times higher than that of standard 2-view chest radiograph. Because DT is a relatively new technique, the number of published dose reduction studies are limited (11).
In our study, we sought to optimize the LD setting for DT by varying the DT parameters. Based on previous studies, we first added a 0.3 mm copper filter (12, 13). Hamer et al reported that subjectively equivalent chest radiographic image quality was found with an estimated 30% dose reduction after the addition of 0.3 mm copper filter with flat-panel CsI/a-Si technology (12). By adding a copper filter with DT, the ESD was decreased by 38% in comparison to the SD setting. This was comparable with previous study. When dose ratio was changed to 1 : 5 from 1 : 10 with the use of a Cu filter, the ESD was reduced by 37-50%. With a 1 : 10 dose ratio (in a case of 2 mAs in scout view), the cumulative mAs of all 60 views should be 20 mAs, or 0.33 mAs per view. Therefore, this decrease in the ESD was reasonable. However, the ESD in 1 : 5 was not one half of the ESD in 1 : 10. This was why that exposure time of equipment was adjusted to several steps (ex. 25 ms, 40 ms, 64 ms). As kVp is decreased, ESD is usually decreased. In our study, there was little correlation between kVp and ESD. The reasons of that were as follows: First, we used AEC. Though we decreased kVp, exposure was increased to maintain same density due to AEC. Second, nonhomogeneity of effective patient dose, image quality, and efficiency according to kVp might have been due to complex phenomenon in relation with k-edge effect of detector material (CsI), and absorption coefficient of the human molecular structure. Accordingly, the selected LD DT parameters were 100 kVp, a dose ratio 1 : 5, and with the use of an additional copper filter. The estimated effective dose was 62 µSv using the LD setting, which showed a 56.7% decrease compared with the SD setting. This result was about 2-3 times that of standard postero-anterior chest radiograph and was comparable with the dose of standard 2-view chest radiograph.
Evaluated image quality was not significantly different between the LD and SD settings, with the exception of images of micronodules in the thick area. Using the SD setting, the detection sensitivities for micronodules and subcentimeter nodules were 55-95% and 88-95%, respectively. This was compatible with previously reported data (2, 7). Dobbins et al. (7) reported 53% and 71% detection sensitivity for 3-5 mm nodules and 5-10 mm nodules, respectively. Vikgren et al. (2) reported 86% sensitivity for nodules less than 4 mm and near 100% visibility for nodules above 5 mm. These results both show a relatively low detection rate for nodules of less than 4 mm. However, in a clinical setting, detection of nodules larger than 4 mm is more important than detection of smaller nodules, which would not be considered actionable by criteria of the Fleischner Society (14). Using the LD setting, detection sensitivities were similar to those of SD setting, with the exception of micronodules in the thick area (63% vs. 44% for SD and LD, respectively). The reason for this might be a decrease in signal and an increase in noise due to the attenuation of vertebral bodies using the LD (Fig. 3). Though nodules of less than 4 mm would not be clinically significant, detecting these smaller nodules for some patients, such as metastasis work-up patients and smokers would be important. Moreover, the National Lung Cancer Trial reported 20% fewer lung cancer deaths seen among smokers screened with low-dose spiral CT than with chest X-ray (15). Dobbins and McAdams proposed potential implementation strategies for tomosynthesis (16). According to the latter paper, DT would be a better option for high-risk patients, such as current or former smokers at risk for lung cancer and metastasis work-up patients, by maximizing the chances for improved patient outcomes and minimizing cost, radiation dose and workflow issues. Thus, we propose that the SD setting is more suitable for high risk patients and for further evaluation of suspicious lesions seen in a previous standard chest radiograph, detection capability is more important than radiation issues in these patients. On the other hand, LD setting would be more suitable for low risk patients such as non-smokers, or young and pediatric patients, as avoiding unnecessary radiation is more important in these patients.
There were several limitations to our study. First, we used an anthropomorphic chest phantom instead of human. Detection of nodules in the phantom might have been easier than those in human because there was no overlying structures or decoy such as pulmonary vessels in this phantom.
Second, we evaluated image quality with artificial pulmonary nodules using globular grains. Because these grains were homogenous, regular and dense than real pulmonary nodules, detection of artificial nodules also might have been easier than detection of real nodules. However, the primary objective of this study was to compare image quality between SD and LD settings, and the overall detection sensitivities for pulmonary nodules in our study did not differ from those of previous studies (2, 7). Third, image quality was evaluated by nodule detection only. If interstitial pattern, ground glass opacity, or catheters had been added, or if evaluation by European Guidelines on Quality Criteria for Diagnostic Radiographic Images had been performed, we might have obtained more information regarding image quality (17). However, because we used a phantom replaced with Styrofoam replacing the lung, these options were not available. Kim et al. and Yoo et al. tried LD DT in an actual clinical environment using our protocol and confirmed the usefulness of LD DT for several clinical applications (18-20).
In the present study, the dose of LD DT was reduced to the similar dose with a standard 2-view chest radiograph. Image quality at the LD setting was comparable to that of the SD setting for nodules greater than 4 mm.
In conclusion, we suggest that LD DT can be useful for nodule detection bigger than 4 mm without an increase in radiation dose as compared with a standard 2-view chest radiograph and without a significant decrease in image quality from that of SD DT. We recommend that LD or SD DT should be applied appropriately according to patient characteristics.
Figures and Tables
Fig. 1
Anthropomorphic chest phantom for evaluation of image quality. Three sets of Styrofoam embedded with artificial nodules were inserted into empty chest phantom.
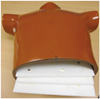
Fig. 2
Organ doses for SD DT and LD DT settings. Doses for lung and bone marrow were decreased substantially more in LD setting than in SD setting. SD = standard default, DT = digital tomosynthesis, LD = low dose
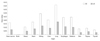
Fig. 3
Images at standard dose (SD) and at low dose (LD) digital tomosynthesis.
A. Magnified SD setting image. B. Magnified LD setting image. SD and LD images show clearly subcentimeter nodule (arrowhead) in paravertebral area. However, micronodule (arrow) in paravertebral area is less clearly showed in LD image (B) than on SD image (A).
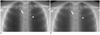
References
1. Dobbins JT 3rd, Godfrey DJ. Digital x-ray tomosynthesis: current state of the art and clinical potential. Phys Med Biol. 2003. 48:R65–R106.
2. Vikgren J, Zachrisson S, Svalkvist A, Johnsson AA, Boijsen M, Flinck A, et al. Comparison of chest tomosynthesis and chest radiography for detection of pulmonary nodules: human observer study of clinical cases. Radiology. 2008. 249:1034–1041.
3. Brenner DJ, Hall EJ. Computed tomography--an increasing source of radiation exposure. N Engl J Med. 2007. 357:2277–2284.
4. Hall EJ, Brenner DJ. Cancer risks from diagnostic radiology. Br J Radiol. 2008. 81:362–378.
5. McCollough CH, Primak AN, Braun N, Kofler J, Yu L, Christner J. Strategies for reducing radiation dose in CT. Radiol Clin North Am. 2009. 47:27–40.
6. The 2007 Recommendations of the International Commission on Radiological Protection. ICRP publication 103. Ann ICRP. 2007. 37:1–332.
7. James TD, McAdams HP, Song JW, Li CM, Godfrey DJ, DeLong DM, et al. Digital tomosynthesis of the chest for lung nodule detection: interim sensitivity results from an ongoing NIH-sponsored trial. Med Phys. 2008. 35:2554–2557.
8. Quaia E, Baratella E, Cioffi V, Bregant P, Cernic S, Cuttin R, et al. The value of digital tomosynthesis in the diagnosis of suspected pulmonary lesions on chest radiography: analysis of diagnostic accuracy and confidence. Acad Radiol. 2010. 17:1267–1274.
9. Sabol JM. A Monte Carlo estimation of effective dose in chest tomosynthesis. Med Phys. 2009. 36:5480–5487.
10. Båth M, Svalkvist A, von Wrangel A, Rismyhr-Olsson H, Cederblad A. Effective dose to patients from chest examinations with tomosynthesis. Radiat Prot Dosimetry. 2010. 139:153–158.
11. Svalkvist A, Båth M. Simulation of dose reduction in tomosynthesis. Med Phys. 2010. 37:258–269.
12. Hamer OW, Sirlin CB, Strotzer M, Borisch I, Zorger N, Feuerbach S, et al. Chest radiography with a flat-panel detector: image quality with dose reduction after copper filtration. Radiology. 2005. 237:691–700.
13. Dobbins JT 3rd, Samei E, Chotas HG, Warp RJ, Baydush AH, Floyd CE Jr, et al. Chest radiography: optimization of X-ray spectrum for cesium iodide-amorphous silicon flat-panel detector. Radiology. 2003. 226:221–230.
14. MacMahon H, Austin JH, Gamsu G, Herold CJ, Jett JR, Naidich DP, et al. Guidelines for management of small pulmonary nodules detected on CT scans: a statement from the Fleischner Society. Radiology. 2005. 237:395–400.
15. National Lung Screening Trial Research Team. Aberle DR, Adams AM, Berg CD, Black WC, Clapp JD, et al. Reduced lung-cancer mortality with low-dose computed tomographic screening. N Engl J Med. 2011. 365:395–409.
16. Dobbins JT 3rd, McAdams HP. Chest tomosynthesis: technical principles and clinical update. Eur J Radiol. 2009. 72:244–251.
17. Carmichael JHE, Maccia C, Moores BM, Oestmann JW, Schibilla H, Teunen D, et al. EUR 16260 - European Guidelines on Quality Criteria for Diagnostic Radiographic Images. 1996. Brussels: European Communities.
18. Kim EY, Chung MJ, Lee HY, Koh WJ, Jung HN, Lee KS. Pulmonary mycobacterial disease: diagnostic performance of low-dose digital tomosynthesis as compared with chest radiography. Radiology. 2010. 257:269–277.
19. Kim EY, Chung MJ, Choe YH, Lee KS. Digital tomosynthesis for aortic arch calcification evaluation: performance comparison with chest radiography with CT as the reference standard. Acta Radiol. 2012. 53:17–22.
20. Yoo JY, Chung MJ, Choi B, Jung HN, Koo JH, Bae YA, et al. Digital tomosynthesis for PNS evaluation: comparisons of patient exposure and image quality with plain radiography. Korean J Radiol. 2012. 13:136–143.