Abstract
Objective
To assess the technical feasibility and local efficacy of percutaneous radiofrequency ablation (RFA) combined with transcatheter arterial chemoembolization (TACE) for an intermediate-sized (3-5 cm in diameter) hepatocellular carcinoma (HCC) under the dual guidance of biplane fluoroscopy and ultrasonography (US).
Materials and Methods
Patients with intermediate-sized HCCs were treated with percutaneous RFA combined with TACE. RFA was performed under the dual guidance of biplane fluoroscopy and US within 14 days after TACE. We evaluated the rate of major complications on immediate post-RFA CT images. Primary technique effectiveness rate was determined on one month follow-up CT images. The cumulative rate of local tumor progression was estimated with the use of Kaplan-Meier method.
Results
Twenty-one consecutive patients with 21 HCCs (mean size: 3.6 cm; range: 3-4.5 cm) were included. After TACE (mean: 6.7 d; range: 1-14 d), 20 (95.2%) of 21 HCCs were visible on fluoroscopy and were ablated under dual guidance of biplane fluoroscopy and US. The other HCC that was poorly visible by fluoroscopy was ablated under US guidance alone. Major complications were observed in only one patient (pneumothorax). Primary technique effectiveness was achieved for all 21 HCCs in a single RFA session. Cumulative rates of local tumor progression were estimated as 9.5% and 19.0% at one and three years, respectively.
Radiofrequency ablation (RFA) is used widely for the local treatment of hepatocellular carcinoma (HCC) (1). Although the therapeutic efficacy of RFA of small HCC (i.e., < 2 cm) is comparable to surgical outcome (2), RFA has shown poor local tumor control when the tumor size exceeds 3 cm (3). This is because in these cases, an ablation zone created by RFA is not adequate enough to cover the large primary tumor and any microscopic satellite nodules that may be present at approximately the primary tumor (4). Therefore, it is generally agreed that patients with HCCs < 3 cm in the greatest diameter are the best candidates for RFA according to the guidelines for the management of HCC (5, 6).
Recently, combined transcatheter arterial chemoembolization (TACE) and RFA has attracted attention as a more promising technique for improving the local tumor control of HCCs, even lesions with a maximum diameter > 3 cm (7-10). Combined TACE and RFA have several advantages over RFA treatment alone. Theoretically, hypoxic injury by embolization along with the chemotherapeutic effect is synergic to thermal ablation by lowering the convection by blood flow and decreasing the impedance in the tumor (11, 12). In addition, a disruption of the intratumoral septa after chemoembolization may facilitate heat distribution within the tumor and decreased perfusion-mediated tissue cooling by embolization results in a larger ablation zone during the RFA procedure (13). Moreover, satellite nodules, which are found more commonly around large HCCs can be controlled by TACE (14).
In terms of the guidance for electrode placement during the RFA procedure, the retained iodized oil by prior TACE is a good landmark for accurate targeting and overlapping. This merit is magnified when the size of the tumor is large, thereby requiring multiple overlapping treatments. Therefore, biplane fluoroscopy (both anteroposterior and lateral projections), which can facilitate easier targeting and overlapping ablations using accumulated iodized oil by prior TACE as a landmark, can be considered an alternative guiding modality in this setting (15). On the other hand, ultrasonography (US) or CT/CT fluoroscopy have been used exclusively for the guidance of RFA in combined TACE and RFA (7, 8, 10, 16, 17), but few have described the dual guidance of biplane fluoroscopy and US for intermediate-sized (3-5 cm) HCC (15). Therefore, the purpose of this study was to evaluate the technical feasibility and local efficacy of RFA combined with TACE for intermediate-sized HCCs under the dual guidance of biplane fluoroscopy and US.
This retrospective study was approved by the institutional review board and the need for informed consent was waved. From February 2009 to September 2010, patients who met the following inclusion criteria were assessed and treated with combination of TACE and RFA: 1) 3 or less HCCs, 2) the size of the largest tumor ranging in size from 3 cm to 5 cm; 3) Child-Pugh class A or B; 4) no evidence of vascular invasion or extrahepatic metastases on the CT or MR images; 5) absence of severe coagulopathy (i.e., prothrombin activity < 40% or platelet count < 40000/mL); and 6) tumor located at least 1 cm away from the hilar bile duct. All patients suspected of having HCC underwent a routine physical examination, blood laboratory tests, and dynamic CT and/or MRI. The diagnosis of HCC was based on the American Association for the Study of Liver Diseases guidelines (18) as follows: typical vascular pattern (hypervascular in the arterial phase, and wash-out in the portal/delayed phase) of the liver nodule in at least one of the dynamic CT or MRI. No patient underwent a percutaneous biopsy. The location and size of each patient's HCC was evaluated using the CT or MR images. Tumor size was defined as the maximum diameter measured on the CT or MR images, and the segmental location of the tumor was recorded in the data sheet according to the Couinaud nomenclature.
During the study period, 21 consecutive patients with HCCs were included (Tables 1, 2). Among them, 2 patients had 2 HCC nodules each. Both patients had one tumor larger than 3 cm at the longest diameter and the other smaller than 3 cm, making a total of 23 HCCs. Percutaneous RFA was also performed for these 2 HCCs < 3 cm, but these 2 lesions were not of our interest in this study, and thus excluded from the analysis. Of the 21 patients, 16 patients had no treatment history of HCC, whereas the other 5 patients had a previous treatment history using the following methods: hepatic resection (n = 3) or percutaneous RFA (n = 2). The size of 21 HCCs was 3.6 ± 0.4 cm in the longest diameter with a range of 3-4.5 cm (median: 3.5 cm). Seventeen (81.0%) HCCs were located in the subphrenic region, either directly contacting the diaphragm or within 1 cm from the diaphragm.
For the patients who met the inclusion criteria, planning US was performed by one certificated abdominal radiologist with at least 8 years' experience in liver US (more than 15000 cases) and US-guided interventional procedures (more than 2000 cases) including biopsy, drainage and RFA, at the beginning of this study. Through the planning US, the radiologist searched for the HCC thoroughly and evaluated the expected electrode path, adjacent organ vulnerable to thermal injury, tumor size, lesion conspicuity, and heat-sink effect to determine if percutaneous RFA was feasible. All planning US were performed with a multi-frequency 4C1 convex array probe (Acuson Sequoia 512, Siemens Medical Solutions, Mountain View, CA, USA). At the time of planning US, the radiologist was aware of the patients' clinical information and liver CT and/or MR images. After a careful evaluation of the prior liver CT and/or MR images, the radiologist searched for the HCC thoroughly and evaluated the expected electrode path, adjacent organ vulnerable to thermal injury, tumor size, lesion conspicuity, and heat-sink effect to determine if percutaneous RFA was feasible (19).
All TACE procedures were performed on an inpatient basis by one of 2 interventional radiologists with at least 9 years of experience in interventional radiology at the starting point of this study. The hepatic artery was catheterized after celiac and superior mesenteric arteriography using a 5 Fr catheter (Cook, Bloomington, IN, USA). Depending on the size, location and arterial supply of the tumor, the 3 Fr microcatheter (Microferret; Cook, Bloomington, IN, USA) was advanced toward the tumor-feeding arteries for selective embolization. Therefore, segmental embolization of the tumor supplying artery was performed, sparing the majority of hepatic parenchymal arterial supply, using an emulsion of iodized oil (Lipiodol; Andre Gurbet, Aulnay-sous-Bois, France) and doxorubicin hydrochloride (Adriamycin RDF; Ildong Pharmaceutical, Seoul, Korea). An infusion of this emulsion was performed until arterial flow stasis had been achieved and/or iodized oil was visualized in the portal branches. The dose of embolization agent was determined according to the tumor size, tumor number, feeding vessels and liver function status. This procedure was followed by the embolization of gelatin sponges (1-mm in diameter; Gelfoam; Upjohn, Kalamazoo, MI, USA). After embolization, angiography was performed again to determine the extent of the vascular occlusion and the presence of any residual tumor staining.
Within 14 days after TACE, percutaneous RFA was performed by a radiologist with more than 4 years' experience (> 200 cases) in RFA of HCC at the starting point of this study. The patients were placed in the supine position and treated under local anesthesia or general anesthesia depending on the tumor size and location. When the index tumor was abutting the portal vein, we preferred general anesthesia rather than local anesthesia. RFA was performed in an interventional suite equipped with a flat-panel biplane fluoroscopy/angiography instrument (Allura Xper FD 20/10, Philips Healthcare, Best, The Netherlands). US guidance was used concurrently to determine the safe skin entry site and to enable accurate targeting of the index tumor, as well as monitoring of the ablation process. Seventeen-gauge, internally cooled electrodes with a 3 cm-long exposed tip (Cool-tip; Valleylab, Boulder, CO, USA or Well-point RF Electrode; STARmed, Goyang, Korea) were used. RF energy was delivered using an impedance-based control algorithm of the generator according to the manufacturer's instructions for each device. Artificial ascites was infused, whenever it was required, to improve the sonic window and decrease the degree of collateral thermal injury to the adjacent diaphragm or colon (20, 21). The site of skin puncture was determined based on the findings of both biplane fluoroscopy and US. Therefore, traversal of the critical structures, such as colon, gallbladder, and large vessels, was avoided in all cases. Although the tumor was located in the subphrenic region, a transhepatic approach not traversing the thoracic cavity was possible in almost all cases using the oblique approach.
Real-time biplane fluoroscopy was used to place the radiofrequency electrode through the tumors, if the index tumor was visible on both anteroposterior and lateral projections due to iodized oil retained from prior TACE. The targeting time, which is the time measured from the skin puncture of the electrode to the placement of the electrode through the index tumor, was typically within one minute. To achieve adequate ablative margin (at least 0.5 cm), multiple overlapping ablations were applied as needed, depending on the tumor size, shape, and location. The number of radiofrequency electrodes was dependent on the visibility of the target tumor on fluoroscopy and the location of the tumor. Generally, multiple electrodes (up to 3 electrodes) were used if the lesion conspicuity on fluoroscopy was not radiopaque enough for multiple overlapping treatments. In addition, if the tumor was located close to any critical structures vulnerable to thermal injury, multiple electrodes were used for precise placement of the electrode. When multiple electrodes were used, sequential ablation of each electrode was performed since generator capable of delivering RF energy with switching mode was not available at the time of the study period. Multiple overlapping treatments were possible in most tumors under the real-time guidance of biplane fluoroscopy, because the visibility of iodized oil retained remained almost unchanged after ablation cycles. The ablation time was variable and dependent on the US findings. If a large echogenic zone was obtained in 3-6 min, the tip of the electrode was repositioned under real-time guidance of biplane fluoroscopy. In other words, a short ablation time with a multiple overlapping strategy rather than a standard 12 min single ablation (i.e., 6 min × 2 overlapping ablations rather than 12 minutes × 1 ablation) was adopted because we believe that the former could create a larger ablation zone than the latter. If the index tumor was not clearly demonstrated on the fluoroscopic imaging, US guidance alone was used for the RFA procedure. At the end of the procedure, tract ablation was performed to prevent bleeding or tumor seeding.
Computed tomography was performed immediately after RFA to evaluate the technical success and procedure-related complications. When the CT images depicted the presence of a nonenhancing area surrounding the entire tumor, it was defined as a technical success (10, 22). A second RFA session was undertaken only when nodular enhancement was observed near the accumulated iodized oil at the CT images (10, 22). The patients were then followed up with contrast-enhanced CT, one month later, and then every three months after treatment. The overall follow-up time was defined as the interval between the first RFA and either local tumor progression or the last follow-up visit by May 23, 2012.
The presence of major complications was evaluated based on the previous guideline of standardized terminology (23), which is defined as any event leading to substantial morbidity and disability, increased level of care, lengthened hospital stay, or a required blood transfusion or interventional drainage procedure. All other complications were deemed to be minor.
In addition, therapeutic efficacy of RFA was assessed based on standardization paper of image-guided tumor ablation (23). Technical success was assessed based on immediate post-RFA CT images. Primary technique effectiveness was evaluated by dynamic CT performed one month after the combined TACE and RFA. At dynamic liver CT, local tumor progression was defined as a new enhancing lesion within or adjacent to the ablation site (23). The cumulative rate of local tumor progression was estimated with the use of Kaplan-Meier method. A distant metastasis was defined as a new HCC in the liver distant from the treated nodule or in extrahepatic regions.
Radiofrequency ablation was performed with a 2-week interval after initial TACE. At the starting point of this study, a sufficient time interval between the 2 procedures was preferred due to the safety concerns of the patients. On the other hand, the time interval between the 2 procedures was subsequently reduced to less than a week. The mean time interval between the 2 procedures was 6.7 d, ranging from 1 to 14 d. Artificial ascites was introduced in 17 (81.0%) patients immediately before placing the electrodes to minimize collateral thermal injury and enhance the sonic window. A single electrode was used in 16 (76.2%) patients and 3 electrodes were used in the other 5 (23.8%) patients. After segmental TACE, 20 (95.2%) out of 21 HCCs were visible on fluoroscopy, and were ablated under biplane fluoroscopy guidance assisted by US. The remaining HCC nodule, which was poorly visible on fluoroscopy, was ablated under US guidance alone. Multiple overlapping ablations under US guidance alone were technically difficult due to the echogenic zone induced by prior ablation cycles. A long RFA procedure time (total ablation time: 31.1 ± 9.0 min; range, 17.5-47 min) was required to achieve a sufficient ablative margin using multiple overlapping ablations (the number of overlapping ablations: 5.9 ± 2.0; range: 3-12).
On the CT images, the mean diameters of the ablation zone for the long and short axes induced by RFA were 5.2 ± 0.4 cm and 3.8 ± 0.2 cm, respectively. Technical success was achieved in all 21 HCCs after a single RFA session (Fig. 1). Primary technique effectiveness based on the one-month follow-up CT was also achieved in all 21 HCCs.
There were no treatment-related deaths. There was only one major complication, which was a pneumothorax necessitating chest tube placement for 4 days. This was an unexpected complication due to an unplanned transpulmonary approach. It may have been caused by a transgression of the lower margin of the basal lung by the lesser caudal angled approach, which could have been avoided easily if a steeper approach to transgress the liver, not the lung, had been performed. There were 8 minor complications: post-ablation syndromes (n = 5) and diaphragmatic thermal injury (n = 3).
Local tumor progression was found in 4 (19.0%) of 21 HCCs during the follow-up period (mean follow up: 24.8 ± 9.9 months; range: 6.5-37.3 months). Cumulative rates of local tumor progression were estimated as 9.5% and 19.0% at 1 and 3 years, respectively (Fig. 2). Four HCCs, which were found to have local tumor progression 7, 11, 15, and 16 months after the combined treatment, had an adequate ablative margin after the initial combined treatment. The 4 HCCs with local tumor progression were treated with RFA (n = 3) and TACE (n = 1), respectively. A distant intrahepatic metastasis was found in 11 (52.4%) of 21 patients, which were managed with repeated RFA (n = 2), TACE (n = 3), combined TACE and RFA (n = 5) or radiation therapy (n = 1). None of the patients died during the follow-up period.
This study demonstrates that RFA combined with TACE under dual guidance of biplane fluoroscopy and US is technically feasible and effective in controlling intermediate-sized HCCs. Technical success was achieved after single RFA session in all 21 HCC nodules through multiple overlapping treatments (mean: 5.9 times). This treatment method seems to have an acceptable rate of local tumor progression of 19.0% (4/21) at the end of the third year. Pneumothorax was a major complication in only one patient and was resolved after chest tube insertion. Therefore, this dual guiding method can be considered a viable option for RFA combined with TACE.
In terms of combined TACE and RFA for intermediate-sized HCCs, several studies have employed US alone as a guiding modality for RFA (9, 10). In a study by Kim et al. (9), RFA was performed 3 days to 2 weeks after TACE, which was similar to our study (1 to 14 days after TACE). Although the mean size of the tumors between the 2 studies is similar (our study: 3.6 ± 0.4 cm versus the study by Kim et al. (9): 3.8 ± 0.5 cm), the number of overlapping ablations was larger in this study than in that study (5.9 ± 2.0 vs. 3.1 ± 1.7). Consequently, second RFA session was not required in our study, whereas it was 5.3% (3/57) for patients in Kim et al. (9) Moreover, although statistical significance was not reached (p = 0.1088, Fisher's exact test), the local tumor progression rate at the end of the third year was lower in our study (19.0%, 4/21) than Kim et al. (9) (40.4%, 23/57). This suggests that the dual guidance of biplane fluoroscopy and US has an advantage over US guidance alone. In general, large HCCs are often difficult to ablate under US alone guidance because multiple overlapping ablations are technically difficult due to echogenic zone generated by previous ablation cycles obscuring the target tumor and electrode. Furthermore, the US visibility of the tumor is sometimes poor when the index tumor is located in the subphrenic region (24, 25). In addition, in cases of combined treatment, prior chemoembolization can alter the sonographic conspicuity of the index tumor owing to the variable uptake of iodized oil and chemotherapeutic agent in the tumor and adjacent hepatic parenchyma (26).
Recently, Morimoto et al. (10) reported combined TACE and RFA of intermediate-sized HCC performed on the same day. The mean size (3.6 ± 0.7 cm) of the tumors in Morimoto et al. (10) was similar to that (3.6 ± 0.4 cm) of our study. Although statistical significance was not reached (p = 0.3451, Fisher's exact test), the local tumor progression rate at the end of the 3rd year was lower in Morimoto et al. (10) (5.3%, 1/19) than in our study (19.0%, 4/21). On the other hand, the number of overlapping ablations (1.6 ± 0.6) was significantly smaller in Morimoto et al. than in our study (5.9 ± 2.0). Nevertheless, the size of the ablation zone (long axis: 5.8 ± 1.3 cm and short axis: 5.0 ± 1.1 cm) in Morimoto et al. (10) was slightly larger than that in our study (long axis: 5.2 ± 0.4 cm and short axis: 3.8 ± 0.2 cm). This can be explained by the fact that the time interval between TACE and RFA was different (the study by Morimoto et al: same day versus this study: mean 6.7 d, range: 1-14 d). Although there is no consensus regarding the ideal time interval between TACE and RFA, it is believed that the shorter time interval between the 2 procedures provides better therapeutic efficacy with a larger ablation zone by the reduction of perfusion-mediated tissue cooling using embolization, which is supported by Morimoto et al. (10). In our study, the retained iodized oil was not radiopaque enough to place the electrode under biplane fluoroscopy guidance due to the washout of iodized oil from the target tumor when RFA was performed 2 weeks after TACE. In addition, the size of the ablation zone by RFA after TACE was smaller than expected with a 2-week interval after TACE. The size of the ablation zone would decrease with time after TACE. Therefore, the time interval between the 2 procedures was reduced to less than a week during the study period. Technical success was achieved after 1.1 ± 0.2 RFA sessions by Morimoto et al. (10) and after a single RFA session in our study, which suggests that US alone guidance for RFA is technically difficult for intermediate-sized HCCs compared to dual guidance of biplane fluoroscopy and US (Fig. 1).
The merit of biplane fluoroscopy-guided RFA for HCC after TACE is well documented in a recent study (15). The point that deserves emphasis is that targeting and multiple overlapping ablations during RFA procedure for HCC with retained iodized oil can be performed easily under real-time guidance of biplane fluoroscopy. This is because simultaneous visualization of the anteroposterior and lateral projection images improves the spatial relationship of the tumor with the retained iodized oil, which contributes to a faster and more efficacious procedure. Unlike US, the lesion conspicuity was not hindered by microbubbles generated by previous ablation cycles on fluoroscopy; thereby multiple overlapping ablations can be performed with high confidence. In this study, multiple overlapping ablations were performed up to 12 times. Although US was also used as an adjuvant guiding modality, US was used to avoid traversing the critical intrahepatic or extrahepatic structures during targeting the tumor and monitoring the ablation procedure.
Computed tomography fluoroscopy has also been used as a guiding modality for RFA combined with TACE (7, 8, 16). Similar to guidance under biplane fluoroscopy, microbubbles within and around the tumor does not prevent repositioning of the electrode in the tumor with CT fluoroscopy, and the accumulation of iodized oil in a tumor is helpful for inserting the electrode precisely in the tumor. On the other hand, the major drawback of this method is the substantial radiation exposure to both the patients and physicians (16). In addition, targeting a hepatic dome HCC would be technically cumbersome because transhepatic approach (oblique approach) is sometimes difficult due to the limited range of CT gantry tilting. Although the transthoracic approach for a dome lesion is generally considered safe, a pneumothorax can occur in 38-70% of cases, requiring frequent chest tube placement (27-29). Compared to CT fluoroscopy, the major strength of biplane fluoroscopy is the relatively low radiation exposure to the patients and physicians. Furthermore, the easier targeting of subphrenic HCCs, which is often difficult to visualize on US, through an oblique approach without thoracic transgression is a potential advantage of biplane fluoroscopy guidance. One drawback of biplane fluoroscopy guidance compared to CT guidance is that not all HCCs with retained iodized oil are clearly visible on fluoroscopy. In the present study, 4.8% (1/21) of HCCs treated previously with TACE was poorly visible on fluoroscopy; hence US alone-guided RFA was performed.
Recently, Lee et al. (30) reported promising results of switching monopolar RFA with multiple electrodes for 3.1-5.0 cm sized HCC without combining chemoembolization. In that study, technique effectiveness was achieved in 29 (96.7%) of 30 patients and local tumor progression occurred in 3 (10.3%) of 29 patients with technique effectiveness during the follow-up period (mean, 12.5 months). Although long-term results are not available, this switching monopolar RFA with multiple electrodes may have a potential to obviate the need for combining TACE and RFA for intermediate-sized HCC. Further study with long-term results is warranted to verify the therapeutic efficacy of switching monopolar RFA with multiple electrodes.
There were several limitations in this study. First, all RFA procedures were performed by a single radiologist in a single institution. Therefore, the results of this study might have been influenced by the experience of the radiologist and patient population. In addition, unlike US, the accessibility of biplane fluoroscopy is not high in most institutions because it is more expensive than monoplane fluoroscopy. On the other hand, if biplane fluoroscopy is available, using both biplane fluoroscopy and US would be helpful for the guidance of RFA for HCCs with retained iodized oil. Second, the number of patients included was relatively small. Therefore, a large cohort of patients and prolonged follow-up period will be needed to assess its long-term therapeutic efficacy.
In conclusion, percutaneous RFA combined with TACE under dual guidance of biplane fluoroscopy and US is technically feasible and effective for the treatment of intermediate-sized HCCs. This dual guiding technique facilitates accurate targeting and overlapping ablations using the iodized oil retained in the tumor as a landmark. Although the HCC lesions are located in the subphrenic region, the transthoracic approach can be avoided using this technique.
Figures and Tables
Fig. 1
69-year-old female with hepatocellular carcinoma (HCC) of liver.
A. Axial arterial phase T1 weighted image (repetition time/echo time, 4.4/2.1 ms) shows 3 cm-sized enhancing HCC (arrowheads) in segment 5 of liver. B. On planning sonography for radiofrequency ablation (RFA) obtained 7 d after MR imaging, index tumor is seen as heterogeneous, low-echoic lesion (arrowheads). Index tumor measured 3.8 cm in longest diameter which is much larger than MR measurement (3.0 cm). C. After chemoembolization, near compact iodized oil has accumulated in tumor (arrowheads). D. RFA was performed 2 weeks after chemoembolization. To minimize thermal injury to adjacent abdominal wall, 500 mL of artificial ascites was introduced immediately before RFA procedure. Anteroposterior (superior images) and its corresponding lateral (inferior images) fluoroscopic images show electrodes obliquely placed in index tumor with retained iodized oil. Total of 9 overlapping ablations were performed using retained iodized oil, as Anatomic landmark. Retained iodized oil remained almost unchanged through multiple overlapping treatments. Total ablation time was 32 min. E. On sonography after initial electrode placement, echogenicity of index tumor (arrowheads) is slightly increased compared to that of on planning ultrasonography. Electrode was inserted until exposed tip (arrows) passed through tumor, enough to obtain sufficient ablative margin. F. After ablating upper part of index tumor (black asterisk), electrode tip and margin of tumor was invisible due to echogenic zone produced by prior ablations. In addition, margin of lower part of tumor not yet ablated (white asterisk), is also obscured by echogenic zone and its shadow. G. Portal venous phase axial CT image obtained immediately after RFA shows HCC with iodized oil retention (arrow) and nonenhancing area surrounding tumor (arrowheads), indicating technical success.
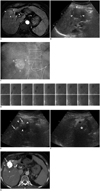
Fig. 2
Local tumor progression rate of 21 hepatocellular carcinomas after combined chemoembolization and radiofrequency ablation.
Cumulative rates of local tumor progression were estimated as 9.5% and 19.0% at 1 and 3 years, respectively '+' marks indicate censored data.
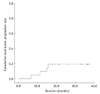
References
1. Rossi S, Ravetta V, Rosa L, Ghittoni G, Viera FT, Garbagnati F, et al. Repeated radiofrequency ablation for management of patients with cirrhosis with small hepatocellular carcinomas: a long-term cohort study. Hepatology. 2011. 53:136–147.
2. Livraghi T, Meloni F, Di Stasi M, Rolle E, Solbiati L, Tinelli C, et al. Sustained complete response and complications rates after radiofrequency ablation of very early hepatocellular carcinoma in cirrhosis: Is resection still the treatment of choice? Hepatology. 2008. 47:82–89.
3. Llovet JM, Bruix J. Novel advancements in the management of hepatocellular carcinoma in 2008. J Hepatol. 2008. 48:Suppl 1. S20–S37.
4. Sasaki A, Kai S, Iwashita Y, Hirano S, Ohta M, Kitano S. Microsatellite distribution and indication for locoregional therapy in small hepatocellular carcinoma. Cancer. 2005. 103:299–306.
5. Bruix J, Sherman M. American Association for the Study of Liver Diseases. Management of hepatocellular carcinoma: an update. Hepatology. 2011. 53:1020–1022.
6. Kudo M, Okanoue T. Japan Society of Hepatology. Management of hepatocellular carcinoma in Japan: consensus-based clinical practice manual proposed by the Japan Society of Hepatology. Oncology. 2007. 72:Suppl 1. 2–15.
7. Takaki H, Yamakado K, Nakatsuka A, Fuke H, Murata K, Shiraki K, et al. Radiofrequency ablation combined with chemoembolization for the treatment of hepatocellular carcinomas 5 cm or smaller: risk factors for local tumor progression. J Vasc Interv Radiol. 2007. 18:856–861.
8. Yamakado K, Nakatsuka A, Takaki H, Yokoi H, Usui M, Sakurai H, et al. Early-stage hepatocellular carcinoma: radiofrequency ablation combined with chemoembolization versus hepatectomy. Radiology. 2008. 247:260–266.
9. Kim JH, Won HJ, Shin YM, Kim SH, Yoon HK, Sung KB, et al. Medium-sized (3.1-5.0 cm) hepatocellular carcinoma: transarterial chemoembolization plus radiofrequency ablation versus radiofrequency ablation alone. Ann Surg Oncol. 2011. 18:1624–1629.
10. Morimoto M, Numata K, Kondou M, Nozaki A, Morita S, Tanaka K. Midterm outcomes in patients with intermediate-sized hepatocellular carcinoma: a randomized controlled trial for determining the efficacy of radiofrequency ablation combined with transcatheter arterial chemoembolization. Cancer. 2010. 116:5452–5460.
11. Goldberg SN, Hahn PF, Tanabe KK, Mueller PR, Schima W, Athanasoulis CA, et al. Percutaneous radiofrequency tissue ablation: does perfusion-mediated tissue cooling limit coagulation necrosis? J Vasc Interv Radiol. 1998. 9(1 Pt 1):101–111.
12. Patterson EJ, Scudamore CH, Owen DA, Nagy AG, Buczkowski AK. Radiofrequency ablation of porcine liver in vivo: effects of blood flow and treatment time on lesion size. Ann Surg. 1998. 227:559–565.
13. Gasparini D, Sponza M, Marzio A, Zanardi R, Bazzocchi M, Cemal Y. Combined treatment, TACE and RF ablation, in HCC: preliminary results. Radiol Med. 2002. 104:412–420.
14. Livraghi T, Goldberg SN, Lazzaroni S, Meloni F, Ierace T, Solbiati L, et al. Hepatocellular carcinoma: radio-frequency ablation of medium and large lesions. Radiology. 2000. 214:761–768.
15. Lee MW, Kim YJ, Park SW, Yu NC, Choe WH, Kwon SY, et al. Biplane fluoroscopy-guided radiofrequency ablation combined with chemoembolisation for hepatocellular carcinoma: initial experience. Br J Radiol. 2011. 84:691–697.
16. Yamakado K, Nakatsuka A, Takaki H, Sakurai H, Isaji S, Yamamoto N, et al. Subphrenic versus nonsubphrenic hepatocellular carcinoma: combined therapy with chemoembolization and radiofrequency ablation. AJR Am J Roentgenol. 2010. 194:530–535.
17. Kang SG, Yoon CJ, Jeong SH, Kim JW, Lee SH, Lee KH, et al. Single-session combined therapy with chemoembolization and radiofrequency ablation in hepatocellular carcinoma less than or equal to 5 cm: a preliminary study. J Vasc Interv Radiol. 2009. 20:1570–1577.
18. Bruix J, Sherman M. Practice Guidelines Committee, American Association for the Study of Liver Diseases. Management of hepatocellular carcinoma. Hepatology. 2005. 42:1208–1236.
19. Rhim H, Choi D, Kim YS, Lim HK, Choe BK. Ultrasonography-guided percutaneous radiofrequency ablation of hepatocellular carcinomas: a feasibility scoring system for planning sonography. Eur J Radiol. 2010. 75:253–258.
20. Song I, Rhim H, Lim HK, Kim YS, Choi D. Percutaneous radiofrequency ablation of hepatocellular carcinoma abutting the diaphragm and gastrointestinal tracts with the use of artificial ascites: safety and technical efficacy in 143 patients. Eur Radiol. 2009. 19:2630–2640.
21. Rhim H, Lim HK. Radiofrequency ablation for hepatocellular carcinoma abutting the diaphragm: the value of artificial ascites. Abdom Imaging. 2009. 34:371–380.
22. Shibata T, Isoda H, Hirokawa Y, Arizono S, Shimada K, Togashi K. Small hepatocellular carcinoma: is radiofrequency ablation combined with transcatheter arterial chemoembolization more effective than radiofrequency ablation alone for treatment? Radiology. 2009. 252:905–913.
23. Goldberg SN, Grassi CJ, Cardella JF, Charboneau JW, Dodd GD 3rd, Dupuy DE, et al. Image-guided tumor ablation: standardization of terminology and reporting criteria. J Vasc Interv Radiol. 2009. 20:7 Suppl. S377–S390.
24. Lee MW, Kim YJ, Park HS, Yu NC, Jung SI, Ko SY, et al. Targeted sonography for small hepatocellular carcinoma discovered by CT or MRI: factors affecting sonographic detection. AJR Am J Roentgenol. 2010. 194:W396–W400.
25. Kang TW, Rhim H, Kim EY, Kim YS, Choi D, Lee WJ, et al. Percutaneous radiofrequency ablation for the hepatocellular carcinoma abutting the diaphragm: assessment of safety and therapeutic efficacy. Korean J Radiol. 2009. 10:34–42.
26. Lee MW, Kim YJ, Park SW, Yu NC, Park HS, Jung SI, et al. Sequential changes in echogenicity and conspicuity of small hepatocellular carcinoma on gray scale sonography after transcatheter arterial chemoembolization. J Ultrasound Med. 2010. 29:1305–1312.
27. Shibata T, Shibata T, Maetani Y, Kubo T, Itoh K, Togashi K, et al. Transthoracic percutaneous radiofrequency ablation for liver tumors in the hepatic dome. J Vasc Interv Radiol. 2004. 15:1323–1327.
28. Toyoda M, Kakizaki S, Horiuchi K, Katakai K, Sohara N, Sato K, et al. Computed tomography-guided transpulmonary radiofrequency ablation for hepatocellular carcinoma located in hepatic dome. World J Gastroenterol. 2006. 12:608–611.
29. Kato T, Yamagami T, Hirota T, Matsumoto T, Yoshimatsu R, Nishimura T. Transpulmonary radiofrequency ablation for hepatocellular carcinoma under real-time computed tomography-fluoroscopic guidance. Hepatogastroenterology. 2008. 55:1450–1453.
30. Lee J, Lee JM, Yoon JH, Lee JY, Kim SH, Lee JE, et al. Percutaneous radiofrequency ablation with multiple electrodes for medium-sized hepatocellular carcinomas. Korean J Radiol. 2012. 13:34–43.