Abstract
Objective
To assess the image quality and effective radiation dose of prospectively electrocardiogram (ECG)-gated high-pitch spiral acquisition mode (flash mode) of dual-source CT (DSCT) coronary angiography (CTCA) in patients with high heart rates (HRs) as compared with retrospectively ECG-gated spiral acquisition mode.
Materials and Methods
Two hundred and sixty-eight consecutive patients (132 female, mean age: 55 ± 11 years) with mean HR > 65 beats per minute (bpm) were prospectively included in this study. The patients were divided into two groups. Collection was performed in group A CTCA using flash mode setting at 20-30% of the R-R interval, and retrospectively ECG-gated spiral acquisition mode in group B. The image noise, contrast-to-noise ratio (CNR), image quality scores, effective radiation dose and influencing factors on image quality between the two groups were assessed.
Results
There were no significant differences in image quality scores and proportions of non-diagnostic coronary artery segments between two groups (image quality scores: 1.064 ± 0.306 [group A] vs. 1.084 ± 0.327 [group B], p = 0.063; proportion of non-diagnostic coronary artery segments: segment-based analysis 1.52% (group A) vs. 1.74% (group B), p = 0.345; patient-based analysis 7.5% (group A) vs. 6.7% (group B), p = 0.812). The estimated radiation dose was 1.0 ± 0.16 mSv in group A and 7.1 ± 1.05 mSv in group B (p = 0.001).
Conclusion
In conclusion, in patients with HRs > 65 bpm without cardiac arrhythmia, the prospectively high-pitch spiral-acquisition mode with image-acquired timing set at 20-30% of the R-R interval provides a similar image quality and low rate of non-diagnostic coronary segments to the retrospectively ECG-gated low-pitch spiral acquisition mode, with significant reduction of radiation exposure.
Computed tomography coronary angiography (CTCA) is a non-invasive tool with high diagnostic accuracy for detection of coronary arterial stenosis (1-6). In retrospectively electrocardiogram (ECG)-gated spiral data acquisition mode (spiral mode), radiation exposure is high and radiation doses can be as high as 12 mSv (7). With prospective ECG-triggering axial acquisition mode (sequential mode, also referred to as "step and shot acquisition"), the average effective radiation dose can be as low as 2.1 mSv. For the first generation of dual-source CT (DSCT), the effective radiation doses of CTCA can be lowered to 1.5-2.5 mSv using prospective ECG-triggering axial acquisition mode (8).
The second generation of dual-source 128-slice CT systems (Definition Flash, Forchheim, Germany) provides a wide coverage of 38.4 mm, allowing CTCA examinations to be performed at a high-pitch, up to 3.4 (9). A gantry rotation time of 0.28 s enables a temporal resolution of 75 ms. Thus all axial planes that comprise the heart can be acquired in approximately 280 ms, and the CTCA acquisition time is reduced to a quarter of a second, depicting of the entire heart within a single heart beat. Previous studies indicate that prospectively ECG-gated high-pitch spiral acquisition mode (flash mode) CTCA provides a high diagnostic accuracy for the assessment of coronary stenosis with an effective radiation dose below 1 mSv (10, 11). This technique is limited by the need for regular sinus rhythm and low heart rates (HRs) (below 60-65 beats per minute, bpm) to ensure an adequate examination using the high-pitch spiral acquisition mode (11, 12). Clinically, although oral metoprolol is administered for reducing HRs, some patients' HRs can not be controlled below 60 bpm. At higher HRs, motion artifacts is a limiting factor for proper assessment of the coronary arteries using CTCA with flash mode (11). Retrospectively ECG-gated spiral data acquisition is commonly used for CTCA in patients with high HRs. Studies have shown that optimal timing of image data acquisition with minimized coronary arterial motion artifacts may shift to systolic intervals in patients with higher HRs, while diastasis shortens and eventually disappears with increasing HRs (13-15). Recent work suggests that a systolic window for data acquisition for high-pitch DSCT in patients with high HRs significantly improves the quality of coronary artery imaging (16). Less is known about the image quality and rate of non-diagnostic coronary artery segments between flash mode and spiral mode in patients with high HRs.
The purpose of our study was to assess the image quality, rate of non-diagnostic coronary artery segments, and radiation dose of flash mode with image-acquired timing set at 20-30% of R-R interval in patients with high HRs, to spiral mode. Potential influencing factors of flash mode were evaluated simultaneously.
From August 2010 to March 2011, two hundred and sixty-eight consecutive patients who were referred to our institution for clinically indicated CTCA (136 male, 132 female; age 55 ± 11 years [mean ± standard deviation]; body mass index [BMI] 23.47 ± 2.56 kg/m2 [range, 19.2-29.8 kg/m2]; mean HR > 65 bpm) were prospectively included in this study. The study protocol had Institutional Review Board approval, and all patients gave informed consent to participate in the study. The enrolled patients were divided into two groups: group A (134 patients: 57 male, 77 female) underwent CTCA using flash mode while group B (134 patients: 79 male, 55 female) used spiral mode. Patients with previous coronary artery interventions, i.e. stenting and/or coronary artery bypass grafts, as well as BMI ≥ 30 kg/m2 were not included in this study. Patients with renal failure or any known allergy to contrast agent were not considered for CTCA. Patients with irregular HRs including atrial fibrillation and premature ventricular contraction were also excluded from this study. No study patients took any beta-receptor antagonist medication for reducing their HRs (15). Heart rate variability (HRV) was defined as the difference between minimum and maximum HR in ten heart beats before image acquisition divided by ten, as previously reported(16, 17).
All CTCA examinations were performed with a second-generation DSCT system (Somatom Definition Flash, Siemens Healthcare, Forchheim, Germany). All patients received a single dose of 0.8 mg nitroglycerin aerosol (Shandong Jingwei Pharma, Taian, China) 3 minutes prior to scanning. Next 60 mL of iohexol (Omnipaque 350 mgI/mL, GE Healthcare, Fairfield, CT, USA) was injected at a flow rate of 6 mL/s, followed by 60 mL saline chasing. Contrast-agent application was controlled by the bolus-tracking technique in the ascending aorta (signal attenuation threshold 100 HU). Data acquisition was initiated with a mean delay of 8 seconds after threshold in the ascending aorta was reached. CT parameter settings were as follows: detector collimation 2 × 64 × 0.6 mm, slice acquisition 2 × 128 × 0.6 mm by means of a z-flying focal spot, gantry rotation time 280 ms, pitch 3.4, tube current 370 mAs per rotation, and tube voltage 100 KV (18). The scan range was from 2 cm below the tracheal carina to the diaphragm. The image acquisition phase started at 20-30% of the R-R interval for the patients in group A. The best image acquisition phase was automatically calculated by the CT software guided by the ECG in group B. Images were reconstructed with a slice thickness of 0.6 mm, a reconstruction increment of 0.4 mm, and a soft-tissue convolution kernel (B26f) was applied. In the case of vessel wall calcifications, additional images were reconstructed using a sharp-tissue convolution kernel (B46) to compensate for blooming artifacts.
Coronary segments were defined by American Heart Association tandard (19). The right coronary artery (RCA) was defined to include segments 1-4, segment 5 is in the left main artery (LM), segments 6-10 are in the left anterior descending artery (LAD), and left circumflex artery (LCX) includes segments 11-15. The intermediary artery was designated as segment 16. Two experienced readers (3 years experience in cardiovascular image readings) assessed all coronary artery segments on cross-sectional source images and multiplanar reformations with subjective image quality evaluation of three-point scales: score 1 (excellent image quality, no motion artifacts), score 2 (moderate, with minor blurring of the vessel wall) and score 3 (non-diagnostic, severe blurring or doubling of the vessel wall).
One independent radiologist, while blinded to the results of the qualitative image analysis, measured image noise and Contrast-to-Noise Ratio (CNR) was calculated. Image noise was defined as the standard deviation of the attenuation value in a region of interest (ROI) placed in the ascending aorta (20). Vessel contrast of the proximal RCA and the LM was defined as the difference in mean attenuation (in HU) between the contrast-enhanced lumen of the vessel and the perivascular tissue. Attenuations were measured by manually placing the ROI in the proximal segment of the RCA and in the LM. The CNR was defined as the ratio of vessel contrast and image noise.
The effective radiation dose was calculated by applying a method from the European Guidelines on Quality Criteria for CT using the dose-length product (DLP) and a conversion coefficient of 0.017 mSv/(mGy × cm) (21). The DLP were taken from the patient protocol provided by the CT system.
Continuous variables were expressed as means ± standard deviations and categorical variables were expressed as frequencies or percentages. A p value below 0.05 was considered statistically significant. All statistical analyses were performed using commercially available software (SPSS, release 17.0, SPSS, Chicago, IL, USA).
Inter-observer agreements regarding the presence and severity of motion artifacts in coronary artery segments was evaluated using Cohen's kappa statistics (kappa > 0.81: excellent agreement; kappa = 0.61-0.80: good agreement; kappa = 0.41-0.60: moderate agreement; kappa = 0.21-0.40: fair agreement; kappa < 0.20: poor agreement).
Differences in the proportions of non-diagnostic segments between the two groups were evaluated with χ2 test. Age, BMI, HR, HRV, scan length, scan time, calcium score, image noise, CNR, mean image quality scores and effective radiation dose were compared between the two groups by the independent-samples t test.
Dual-source computed tomography coronary angiography were successfully performed in all patients without side effects. The average HR and HR variability were 79 ± 9 bpm (range: 66-109 bpm) and 10.72 ± 4.88 bpm (range: 2-41 bpm) in group A, while the values for group B were 80 ± 11 bpm (range: 66-139 bpm) and 10.05 ± 5.69 bpm (range: 0-38 bpm), respectively. The mean BMI was 23.8 ± 2.7 (range: 19.2-29.8) in group A and 23.7 ± 2.5 (range: 19.1-29.2) in group B. The mean scan length was 12.08 ± 1.12 cm (range: 9.6-14.89 cm) in group A and 12.72 ± 1.88 cm (range: 9.8-14.5 cm) in group B. The acquisition time was 247 ± 25 ms (range: 194-417 ms) and 252 ± 22 ms (range: 215-307 ms) in groups A and B, respectively. There were no significant differences in age, BMI, HR, HRV, scan length, scan time or calcium score between the two groups (all p > 0.05). An overview of the results for groups A and B can be found in Table 1.
In total, there were 1842 coronary segments in group A and 1838 segments in group B. The inter-observer agreement for image quality rating was good (group A: kappa = 0.63; group B: kappa = 0.65). Image quality was rated as being excellent (score 1) in 92.3% (1701/1842) of segments in group A and 95.3% (1751/1838) of segments in group B; moderate in 6.1% (113/1842) of segments in group A and 3.0% (56/1838) of segments in group B; and non-diagnostic in 1.5% (28/1842) of segments in group A and 1.7% (31/1838) of segments in group B. There were no significant differences in image quality scores and proportion of non-diagnostic coronary artery segments between groups A and B (image quality scores: 1.064 ± 0.306 vs. 1.084 ± 0.327, respectively, p = 0.063; non-diagnostic coronary artery segments segment-based analysis 1.52% vs. 1.74%, respectively, p = 0.345; and patient-based analysis 7.5% vs. 6.7%, respectively, p = 0.812) (Table 2).
Ten patients had non-diagnostic images due to motion artifacts in group A. In five of these patients the motion artifacts occurred at the RCA and LCX because the acquisition phase fell on the R wave due to high HRV (Fig. 2). Examples of good image quality are displayed in Figure 1, while examples of typical non-diagnostic images are displayed in Figure 2. Table 2 summarizes the results of subjective image quality evaluation in both groups.
The average image noise was 21.4 ± 4.5 HU (range: 19-27 HU) and CNR was 12.1 ± 4.2 (range: 6.4-25.3) in group A, and the corresponding numbers were 20.9 ± 4.3 HU (range: 19-28 HU) and 13.8 ± 5.1 (range: 7.1-28.2) in group B. There were no significant differences in image noise or CNR between the two groups (Table 1).
The average HRV was 2.29 ± 1.06 (95% CI 2.05-2.53) in patients of score 1, 5.17 ± 1.37 (95% CI 4.77-5.58) in patients of score 2, and 8.88 ± 1.53 (95% CI 7.71-10.07) in patients of score 3 in group A. In group B, the average HRV was 2.61 ± 1.85 (95% CI 2.23-2.99), 7.90 ± 3.97 (95% CI 6.47-9.34) and 11.22 ± 5.62 (95% CI 6.89-15.55) in patients of scores 1-3, respectively (Table 2). In group B, four patients' vessels were unassessable from motion artifacts induced by poor cooperation in breath-holding. Diagnostic evaluation of the entire coronary artery tree was obtained in qualified patients using flash mode in acquisition at 20% of the R-R interval (Fig. 3).
The DLP of DSCTCA examinations was 61.17 ± 7.24 mGy·cm in group A and 414.67 ± 61.71 mGy·cm in group B. The estimated radiation dose was 1.04 ± 0.16 mSv (range: 0.7-1.55 mSv) in group A and 7.05 ± 1.05 mSv (range: 5.68-11.92 mSv) in group B (p = 0.001) (Table 1).
There are three scan modes in high pitch DSCT examinations: spiral mode, sequential mode and flash mode (22). In spiral mode, the spiral DSCTCA scan technique delivers the X-ray throughout the entire cardiac cycle. Even with ECG-based tube current modulation, the radiation dose is still high and the effective radiation doses can be as high as 12 mSv (7). In sequential mode, the radiation exposure is turned on only with the optimal phase of the cardiac cycle and then turned off (23). This short time data acquisition can reduce the radiation dose substantially, resulting in an average effective radiation dose of 2.1-4.1 mSv (8, 24-26). Moreover, the "padding" technique allows for an additional exposure time before and after the optimal phase in the cardiac cycle. Overall, the high-pitch spiral mode provides a high diagnostic accuracy for the assessment of coronary stenosis with doses below 1 mSv (11).
The most challenging issue in CTCA examination is non-diagnostic images. The rate of non-diagnostic segments has been reported to be as high as 12% with 64-section CTCA (27), with a range of 1 to 6% with first generation DSCTCA (28-30) and around 10% of patients affected. The high-pitch spiral mode of DSCT permits data acquisition from the entire heart within one heart beat by continuous and fast table movement. Leschka et al. (11) found that 99% of evaluable coronary segments in CTCA performed with the flash mode were with HR of 60 bpm, while the initiation of the imaging was at 60% of the R-R interval. Sensitivity of 94% and specificity of 96% were reported for the detection of significant coronary artery stenosis. High HRs were thought to be problematic in these acquisitions, especially during the high-pitch mode needed for motion-free imaging in patients with a low HR. Thus, regular sinus rhythm and HR ≤ 60 bpm have been considered prerequisites for an adequate examination.
At higher HRs, motion artifacts will lead to a non-diagnostic image, predominantly affecting the mid RCA. The optimal time selection aims to minimize the coronary arterial motion artifacts, especially the artifacts in the mid to distal RCA. The optimal time may shift to systolic intervals in patients with high HRs as diastolic diastasis shortens and eventually disappears with increased HRs (31). With retrospectively ECG-gated DSCT, Adler et al. (32) found that the optimal systolic phase is between 35% and 50% of the R-R interval in patients with HR > 65 bpm. Araoz et al. (15) reported that optimal image sharpness of the coronary arteries was achieved at 35-40% of the R-R interval in patients with HR > 70 bpm. Recently, Goetti et al. (16) suggested initiation of the high-pitch CT scanning at 30% of the R-R interval in high-pitch DSCTCA in patients with high HRs (≥ 70 bpm) significantly improved the image quality of coronary arteries.
Using high-pitch DSCT, Goetti et al. (16) selected forty patients with HR ≥ 70 bpm and chose the 30% of of the R-R interval for triggering of imaging. They found that the systolic window for data acquisition from high-pitch DSCTCA in patients with high HRs (HR ≥ 70 bpm) significantly improves the quality of coronary artery imaging. Based on their finding, we performed CTCA with flash mode with the optimal phase setting at 20-30% of the R-R interval in patients with high HRs (> 65 bpm), and we demonstrated good diagnostic image quality of coronary arteries using these methods.
Mimimal motion artifacts occur in a phase of cardiac cycle with the least coronary artery motion. According to cardiac electrical mechanical coupling theory (33-36), the basic activity of the heart is electric and mechanical activity. After 40-60 ms of electric activity, the mechanical activity begins. Ventricle contraction includes three phases: isovolumetric contraction, rapid ejection and the slow ejection phase. The isovolumetric contraction phase lasts 50 ms, while the rapid ejection phase lasts around 100 ms. In the slow ejection phase, ventricle contraction is relatively slow and occurs about 150 ms after the QRS wave. For example, a high HR of 100 bpm (R-R interval: 600 ms), 30% of the R-R interval would be 180 ms. Data acquisition for high-pitch DSCTCA falls exactly in the slow ejection phase. Regarding the upper limit of HRs of the high pitch mode DSCTA, the scan time of high pitch mode CTCA is about 250 ms and the P-R interval is 120-200 ms. The isovolumetric contraction and rapid ejection phase lasts 150 ms. Thus, 150 + 250 + 200 ms = 600 ms (HR: 100 bpm). This gives an upper limit of HRs of 100 bpm with less coronary arterial motion artifacts via the high pitch mode DSCTCA. Figure 4 illustrates the most important features of the cardiac cycle and the best scan time of HP mode DSCTCA within a single heart beat in patients with high HRs.
A significant negative correlation between overall quality of images of the coronary arteries and HRV during scanning has been consistently reported since the introduction of MDCT cardiac imaging in clinical practice (37, 38). Using 64-Section CT, Leschka et al. (37) found HRV had a strong negative impact on image quality for all coronary segments and each coronary artery. Our data indicate that HRV is an important factor affecting the image quality of High-Pitch DSCTCA in patients with HRs, in agreement with previously reported work (37, 38). The average HRV in patients of score 3 were significantly higher than that of scores 1 and 2 in flash mode and spiral mode DSCTCA.
In our study, we found that four patients' vessels were unassessable using flash mode CTCA due to motion artifacts induced by poor cooperation in breath-holding. In contrast, the flash mode is still able to gain diagnostic evaluation of the entire coronary artery tree although patients cannot hold their breath. This suggests that the influence of respiration seems to be a minor concern for high-pitch spiral mode DSCTCA.
The reported effective doses for prospective CTCA were 2.1-4.3 mSv on 64-slice CT (39-41) and 1.5-2.5 mSv on the first generation DSCT (8, 29). Leschka et al. (11) reported a mean dose of 0.9 mSv in 35 patients that was investigated via high-pitch spiral CT, but with a less stringent control of tube voltage and current (320 mAs). In this study, the average effective radiation dose was about 1.04 mSv for the new flash spiral acquisition mode protocol. This dose is considerably lower than DSCT of 7.05 mSv for the retrospectively spiral scan in this study, and lower than retrospectively ECG-gated CTCA on DSCT reported by others (42). Moreover, it is substantially lower than that of first generation DSCT with reported values of 1.5-2.5 mSv on average (29). These results are similar to that of doses reported in other recent trials that evaluated high-pitch DSCT (9, 11, 43).
There are several limitations to the study that we have performed. First, the image quality grading may have been inluenced by a subjectivity bias. Second, patients with irregular HRs were excluded, especially on atrial fibrillation or premature ventricular contraction. Third, in this study, we did not compare the results of the high-pitch acquisition protocol with sequential mode procols. Further studies will be required for a detailed analysis.
In conclusion, in patients with HRs > 65 bpm without cardiac arrhythmia, the prospectively high-pitch spiral acquisition mode with image acquired timing set at 20-30% of the R-R interval provides a similar image quality and low rate of non-diagnostic coronary segments to the retrospectively ECG-gated low-pitch spiral acquisition mode, with significant reduction of radiation exposure.
Figures and Tables
Fig. 1
HP dual-source computed tomography coronary angiography in 55-year-old woman (heart rate: 99 bpm) from Group A.
Electrocardiogram signal (A) demonstrates depiction of entire heart within single heart beat. Curved reformations of right coronary artery (B), left anterior descending artery (C) and left circumflex artery (D) did not show motion artifacts (score 1). Image quality was classified as excellent (i.e. score 1) in all coronary segments. RCA = right coronary artery, LCX = left circumflex artery
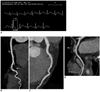
Fig. 2
HP dual-source computed tomography coronary angiography in 40-year-old man (heart rate: 79 bpm) from group A.
Electrocardiogram signal (A) demonstrates that high-pitch spiral mode in acquisition at 20% of R-R interval falls into scan range of R wave because HR variability was too high (26 bpm). Motion artifacts occurred at right coronary artery (B, C) and left circumflex artery (D) (score 3). LCX = left circumflex artery
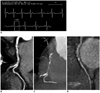
Fig. 3
47-year-old woman (heart rate: 70 bpm) underwent CTCA.
Image is not evaluative and vessels are not differentiable due to effect of respiration using spiral mode (B, C, D). High-pitch spiral mode in acquisition at 20% of R-R interval CTCA was used, and patient had diagnostic evaluation of entire coronary artery tree (F, G, H). Electrocardiogram signal (A, E) demonstrated acquisition window of spiral mode and high-pitch spiral mode. CTCA = computed tomography coronary angiography, LAD = left anterior descending artety, LCX = left circumflex artery
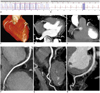
Fig. 4
Most important features of cardiac cycle and optimal scan time of flash mode computed tomography coronary angiography within single heart beat.
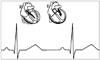
References
1. Husmann L, Herzog BA, Burger IA, Buechel RR, Pazhenkottil AP, von Schulthess P, et al. Usefulness of additional coronary calcium scoring in low-dose CT coronary angiography with prospective ECG-triggering impact on total effective radiation dose and diagnostic accuracy. Acad Radiol. 2010. 17:201–206.
2. Ropers D, Pohle FK, Kuettner A, Pflederer T, Anders K, Daniel WG, et al. Diagnostic accuracy of noninvasive coronary angiography in patients after bypass surgery using 64-slice spiral computed tomography with 330-ms gantry rotation. Circulation. 2006. 114:2334–2341. quiz 2334.
3. Carrascosa P, Capuñay C, Deviggiano A, Goldsmit A, Tajer C, Bettinotti M, et al. Accuracy of low-dose prospectively gated axial coronary CT angiography for the assessment of coronary artery stenosis in patients with stable heart rate. J Cardiovasc Comput Tomogr. 2010. 4:197–205.
4. Xu Y, Tang L, Zhu X, Xu H, Tang J, Yang Z, et al. Comparison of dual-source CT coronary angiography and conventional coronary angiography for detecting coronary artery disease. Int J Cardiovasc Imaging. 2010. 26:Suppl 1. 75–81.
5. Zhang LJ, Wu SY, Wang J, Lu Y, Zhang ZL, Jiang SS, et al. Diagnostic accuracy of dual-source CT coronary angiography: the effect of average heart rate, heart rate variability, and calcium score in a clinical perspective. Acta Radiol. 2010. 51:727–740.
6. Moon JH, Park EA, Lee W, Yin YH, Chung JW, Park JH, et al. The diagnostic accuracy, image quality and radiation dose of 64-slice dual-source CT in daily practice: a single institution's experience. Korean J Radiol. 2011. 12:308–318.
7. Hausleiter J, Meyer T, Hermann F, Hadamitzky M, Krebs M, Gerber TC, et al. Estimated radiation dose associated with cardiac CT angiography. JAMA. 2009. 301:500–507.
8. Scheffel H, Alkadhi H, Leschka S, Plass A, Desbiolles L, Guber I, et al. Low-dose CT coronary angiography in the step-and-shoot mode: diagnostic performance. Heart. 2008. 94:1132–1137.
9. Achenbach S, Goroll T, Seltmann M, Pflederer T, Anders K, Ropers D, et al. Detection of coronary artery stenoses by low-dose, prospectively ECG-triggered, high-pitch spiral coronary CT angiography. JACC Cardiovasc Imaging. 2011. 4:328–337.
10. Alkadhi H, Stolzmann P, Desbiolles L, Baumueller S, Goetti R, Plass A, et al. Low-dose, 128-slice, dual-source CT coronary angiography: accuracy and radiation dose of the high-pitch and the step-and-shoot mode. Heart. 2010. 96:933–938.
11. Leschka S, Stolzmann P, Desbiolles L, Baumueller S, Goetti R, Schertler T, et al. Diagnostic accuracy of high-pitch dual-source CT for the assessment of coronary stenoses: first experience. Eur Radiol. 2009. 19:2896–2903.
12. Wang YN, Li L, Kong LY, Wang ZW, Zhou K, Cao J, et al. [Coronary computed tomographic angiography using low-dose prospectively electrocardiographic triggered high-pitch spiral acquisition by dual-source computed tomography: image quality and radiation dose]. Zhongguo Yi Xue Ke Xue Yuan Xue Bao. 2010. 32:597–600.
13. Seifarth H, Wienbeck S, Püsken M, Juergens KU, Maintz D, Vahlhaus C, et al. Optimal systolic and diastolic reconstruction windows for coronary CT angiography using dual-source CT. AJR Am J Roentgenol. 2007. 189:1317–1323.
14. Weustink AC, Mollet NR, Pugliese F, Meijboom WB, Nieman K, Heijenbrok-Kal MH, et al. Optimal electrocardiographic pulsing windows and heart rate: effect on image quality and radiation exposure at dual-source coronary CT angiography. Radiology. 2008. 248:792–798.
15. Araoz PA, Kirsch J, Primak AN, Braun NN, Saba O, Williamson EE, et al. Optimal image reconstruction phase at low and high heart rates in dual-source CT coronary angiography. Int J Cardiovasc Imaging. 2009. 25:837–845.
16. Goetti R, Feuchtner G, Stolzmann P, Desbiolles L, Fischer MA, Karlo C, et al. High-pitch dual-source CT coronary angiography: systolic data acquisition at high heart rates. Eur Radiol. 2010. 20:2565–2571.
17. Halliburton SS, Sola S, Kuzmiak SA, Obuchowski NA, Desai M, Flamm SD, et al. Effect of dual-source cardiac computed tomography on patient radiation dose in a clinical setting: comparison to single-source imaging. J Cardiovasc Comput Tomogr. 2008. 2:392–400.
18. Lell M, Marwan M, Schepis T, Pflederer T, Anders K, Flohr T, et al. Prospectively ECG-triggered high-pitch spiral acquisition for coronary CT angiography using dual source CT: technique and initial experience. Eur Radiol. 2009. 19:2576–2583.
19. Austen WG, Edwards JE, Frye RL, Gensini GG, Gott VL, Griffith LS, et al. A reporting system on patients evaluated for coronary artery disease. Report of the Ad Hoc Committee for Grading of Coronary Artery Disease, Council on Cardiovascular Surgery, American Heart Association. Circulation. 1975. 51:5–40.
20. Qi W, Li J, Du X. Method for automatic tube current selection for obtaining a consistent image quality and dose optimization in a cardiac multidetector CT. Korean J Radiol. 2009. 10:568–574.
21. Menzel HG, Schibilla H, Teunen D, editors. Publication no. EUR 16262 EN. European guidelines on quality criteria for computed tomography. 2000. Luxembourg: European Commission.
22. Wolf F, Leschka S, Loewe C, Homolka P, Plank C, Schernthaner R, et al. Coronary artery stent imaging with 128-slice dual-source CT using high-pitch spiral acquisition in a cardiac phantom: comparison with the sequential and low-pitch spiral mode. Eur Radiol. 2010. 20:2084–2091.
23. Goo HW. State-of-the-art CT imaging techniques for congenital heart disease. Korean J Radiol. 2010. 11:4–18.
24. Duarte R, Fernandez G, Castellon D, Costa JC. Prospective Coronary CT Angiography 128-MDCT Versus Retrospective 64-MDCT: Improved Image Quality and Reduced Radiation Dose. Heart Lung Circ. 2011. 20:119–125.
25. Hirai N, Horiguchi J, Fujioka C, Kiguchi M, Yamamoto H, Matsuura N, et al. Prospective versus retrospective ECG-gated 64-detector coronary CT angiography: assessment of image quality, stenosis, and radiation dose. Radiology. 2008. 248:424–430.
26. Earls JP, Berman EL, Urban BA, Curry CA, Lane JL, Jennings RS, et al. Prospectively gated transverse coronary CT angiography versus retrospectively gated helical technique: improved image quality and reduced radiation dose. Radiology. 2008. 246:742–753.
27. Raff GL, Gallagher MJ, O'Neill WW, Goldstein JA. Diagnostic accuracy of noninvasive coronary angiography using 64-slice spiral computed tomography. J Am Coll Cardiol. 2005. 46:552–557.
28. Rixe J, Rolf A, Conradi G, Elsaesser A, Moellmann H, Nef HM, et al. Image quality on dual-source computed-tomographic coronary angiography. Eur Radiol. 2008. 18:1857–1862.
29. Stolzmann P, Leschka S, Scheffel H, Krauss T, Desbiolles L, Plass A, et al. Dual-source CT in step-and-shoot mode: noninvasive coronary angiography with low radiation dose. Radiology. 2008. 249:71–80.
30. Scheffel H, Alkadhi H, Plass A, Vachenauer R, Desbiolles L, Gaemperli O, et al. Accuracy of dual-source CT coronary angiography: First experience in a high pre-test probability population without heart rate control. Eur Radiol. 2006. 16:2739–2747.
31. Achenbach S, Ropers D, Holle J, Muschiol G, Daniel WG, Moshage W. In-plane coronary arterial motion velocity: measurement with electron-beam CT. Radiology. 2000. 216:457–463.
32. Adler G, Meille L, Rohnean A, Sigal-Cinqualbre A, Capderou A, Paul JF. Robustness of end-systolic reconstructions in coronary dual-source CT angiography for high heart rate patients. Eur Radiol. 2010. 20:1118–1123.
33. Guyer S. Cardiovascular physiology as a basis for clinical and financial outcomes. J Cardiovasc Manag. 1998. 9:20–24.
34. Tsakiris S, Zarros A. Medical physiology and experimentation: reconsidering the undergraduate examination structure. Adv Physiol Educ. 2006. 30:94–95.
35. Alonso Guerrero A. [Cardiac physiology]. Rev Enferm. 1983. 6:49–54.
36. Saffitz JE. Dependence of electrical coupling on mechanical coupling in cardiac myocytes: insights gained from cardiomyopathies caused by defects in cell-cell connections. Ann N Y Acad Sci. 2005. 1047:336–344.
37. Leschka S, Wildermuth S, Boehm T, Desbiolles L, Husmann L, Plass A, et al. Noninvasive coronary angiography with 64-section CT: effect of average heart rate and heart rate variability on image quality. Radiology. 2006. 241:378–385.
38. Weustink AC, Neefjes LA, Kyrzopoulos S, van Straten M, Neoh Eu R, Meijboom WB, et al. Impact of heart rate frequency and variability on radiation exposure, image quality, and diagnostic performance in dual-source spiral CT coronary angiography. Radiology. 2009. 253:672–680.
39. Herzog BA, Husmann L, Burkhard N, Gaemperli O, Valenta I, Tatsugami F, et al. Accuracy of low-dose computed tomography coronary angiography using prospective electrocardiogram-triggering: first clinical experience. Eur Heart J. 2008. 29:3037–3042.
40. Maruyama T, Takada M, Hasuike T, Yoshikawa A, Namimatsu E, Yoshizumi T. Radiation dose reduction and coronary assessability of prospective electrocardiogram-gated computed tomography coronary angiography: comparison with retrospective electrocardiogram-gated helical scan. J Am Coll Cardiol. 2008. 52:1450–1455.
41. Husmann L, Valenta I, Gaemperli O, Adda O, Treyer V, Wyss CA, et al. Feasibility of low-dose coronary CT angiography: first experience with prospective ECG-gating. Eur Heart J. 2008. 29:191–197.
42. Alkadhi H, Stolzmann P, Scheffel H, Desbiolles L, Baumüller S, Plass A, et al. Radiation dose of cardiac dual-source CT: the effect of tailoring the protocol to patient-specific parameters. Eur J Radiol. 2008. 68:385–391.
43. Achenbach S, Marwan M, Ropers D, Schepis T, Pflederer T, Anders K, et al. Coronary computed tomography angiography with a consistent dose below 1 mSv using prospectively electrocardiogram-triggered high-pitch spiral acquisition. Eur Heart J. 2010. 31:340–346.