Abstract
Objective
To investigate radiation doses in pediatric chest radiography in a national survey and to analyze the factors that affect radiation doses.
Materials and Methods
The study was based on the results of 149 chest radiography machines in 135 hospitals nationwide. For each machine, a chest radiograph was obtained by using a phantom representing a 5-year-old child (ATOM® dosimetry phantom, model 705-D, CIRS, Norfolk, VA, USA) with each hospital's own protocol. Five glass dosimeters (M-GD352M, Asahi Techno Glass Corporation, Shizuoka, Japan) were horizontally installed at the center of the phantom to measure the dose. Other factors including machine's radiography system, presence of dedicated pediatric radiography machine, presence of an attending pediatric radiologist, and the use of automatic exposure control (AEC) were also evaluated.
Results
The average protocol for pediatric chest radiography examination in Korea was 94.9 peak kilovoltage and 4.30 milliampere second. The mean entrance surface dose (ESD) during a single examination was 140.4 microgray (µGy). The third quartile, median, minimum and maximum value of ESD were 160.8 µGy, 93.4 µGy, 18.8 µGy, and 2334.6 µGy, respectively. There was no significant dose difference between digital and non-digital radiography systems. The use of AEC significantly reduced radiation doses of pediatric chest radiographs (p < 0.001).
Conclusion
Our nationwide survey shows that the third quartile, median, and mean ESD for pediatric chest radiograph is 160.8 µGy, 93.4 µGy, and 140.4 µGy, respectively. No significant dose difference is noticed between digital and non-digital radiography systems, and the use of AEC helps significantly reduce radiation doses.
Unnecessary exposure to ionizing radiation is of great concern in both adult and pediatric patients. Pediatric patients deserve special attention owing to their unique characteristics. Children are more sensitive to radiation than adults, and have longer life expectancy, raising the risk of later detrimental effects. Moreover, indiscriminate use of adult imaging protocols on children definitely results in their overexposure to radiation (1-3). Many attempts have been made to reduce radiation doses in pediatric patients. Ionizing radiation can be minimized by reducing the kilovoltage, implementing automatic anatomic tube current modulation, or applying shields (4-8). Less is known, however, about protocols to reduce radiation doses during conventional radiographs. Although several international organizations suggested diagnostic reference levels (DRL) for pediatric chest radiograph (9-11), those studies were performed years ago and did not consider radiation exposure from newer modalities such as computed and digital radiography systems. Similarly in Korea, a few studies on reducing radiation doses of pediatric CT were published, but there have been no studies on radiation doses of pediatric radiograph, so far (6, 7, 12).
The conventional radiograph remains one of the most commonly used diagnostic imaging tools, due to its economic feasibility and accessibility. Moreover, introduction of computed and digital radiography systems may result in unexpected underexposure or overexposure of pediatric patients to radiation. Thus, there is an urgent need for an overall survey of radiation dose in pediatric radiography to describe the current situation (13, 14). Also, investigation on the potential dose reduction effect of newer technologies - computed radiography system, digital radiography system, and automatic exposure control (AEC) - is needed. We performed a nationwide survey to investigate the general radiation dose in pediatric chest radiographs in Korea and to analyze the factors affecting radiation dose.
Throughout the country, we randomly selected hospitals to encompass machines from as many different manufacturers as possible and to reflect the regional distribution of radiography machines and practice. Among them, hospitals which do not regularly take pediatric chest radiographs were excluded. Consequently, we included 149 radiography machines in 135 hospitals.
The study was performed from June 2009 to September 2009. On each machine, a chest radiograph of a phantom representing a 5-year-old child (ATOM® dosimetry phantom, model 705-D, CIRS, Norfolk, VA, USA) was taken at a fixed source-to-image distance of 180 cm, using the hospitals' own protocols. Five glass dosimeters (M-GD352M, Asahi Techno Glass Corporation, Shizuoka, Japan) were horizontally installed at the center of central ray of the phantom, representing the seventh thoracic spine. An entrance surface dose (ESD) was calculated by subtracting the pre-dose from the dose obtained from the phantom. We also measured the output parameters of each machine, including peak kilovoltage (kVp), milliampere, milliampere second (mAs), and half value layer, using an Unfors Xi R/F & MAM detector (Unfors Istruments, Billdal, Sweden).
Each machine's types of radiography systems-digital radiography, computed radiography, or film-screen-, the presence of a dedicated pediatric radiography machine, the presence of an attending pediatric radiologist, the use of an AEC, and the machine's years of operation were also recorded.
To evaluate the diagnostic value of the phantom images, two radiologists analyzed and scored the sharpness of the lung, heart, bone, and the level of noise by consensus. For each factor, a five-point rating scale was used, with one being poor and five being excellent. The overall image quality was evaluated by averaging the point. Scores higher than three were considered acceptable image quality.
The overall difference of ESD according to radiography system (digital radiography and non-digital radiography), the use of AEC, the presence of dedicated pediatric radiography machine and the presence of an attending pediatric radiologist was analyzed by using Student's t test.
Since the international guideline recommendation dose for pediatric chest radiograph was 100 microgray (µGy) (10), we divided the machines into two groups by setting the threshold value as 100 µGy. We used chi-square tests to compare the ratio difference of the aforementioned factors between these two groups whose ESD are lower than 100 µGy (low dose group) and higher than 100 µGy (high dose group). The standard deviation (SD) of ESD was also calculated for each factor compared. To identify the correlation between the machine's years of operation and ESD, Pearson correlation coefficient was calculated. All statistical analyses were performed by using Statistical Package for the Social Sciences (SPSS) version 18.0 (SPSS, Inc, an IBM Company, Chicago, IL, USA). We rejected null hypotheses of no difference if p values were less than 0.05.
The regional distribution of each hospital is summarized in Table 1. Of the 149 radiography machines, 63 (42%) were computed radiography, 83 (56%) were digital radiography, and only three (2%) were film-screen system. Twenty-six of 149 machines (17%) were dedicated to pediatric radiography and were all attended by pediatric radiologists specializing in such radiographs with one exception.
The mean tube kVp and mAs were 94.9 kVp and 4.30 mAs, respectively. Under these exposure conditions, ESD ranged from 18.8 to 2334.6 µGy. The third quartile, median, and the mean values of ESD were 160.8 µGy, 93.4 µGy, and 140.4 µGy, respectively. Seventy-eight machines (52.3%) showed doses less than 100 µGy, whereas 26 (17%) exhibited doses higher than 200 µGy. Figure 1 demonstrated the dose distribution among 149 machines.
We excluded two extreme ESD values of 2334.6 µGy and 832 µGy when obtaining other statistical data. The overall difference of ESD according to radiography system, presence of AEC, presence of dedicated pediatric radiography machine and attending pediatric radiologist is summarized in Table 2. Of the 147 machines, 46 (31.3%) were equipped with AEC, and they showed significantly lower mean ESD than in machines not equipped with AEC (83.6 µGy [SD: 63.4] vs. 144.0 µGy [SD: 92.8]; p < 0.001) (Fig. 2). The mean ESD was 118.5 µGy for digital radiography system, and 135.1 µGy for non-digital radiography system. Though not statistically significant, the mean ESD and SD were lower in the digital radiography system. In the same way, dedicated pediatric radiography machines attended by pediatric radiologists tend to show lower ESD and SD (Fig. 2). Table 3 showed comparison of multiple factors between low and high dose groups. Significantly higher numbers of machines were equipped with AEC in low dose group than high dose group (44.4% vs. 16.2%; p < 0.0001).
The scattergram for the machine's years of operation and ESD is shown in Figure 3. There was no significant correlation between the machine's years of operation and ESD by Pearson correlation coefficient.
In case of phantom images, all images demonstrated well-preserved sharpness at the interface between the lung, heart, and bone. The level of noise in each image was low enough to discern normal structures from each other. All phantom images had scores higher than three in each factors assessed and the average score was also higher than three.
Although radiation doses for adults have been widely investigated, less is known about the optimal doses for pediatric patients. Several international organizations have published recommended doses for pediatric radiograph in the past (Table 4) (9, 10). In addition to being somewhat old, these studies were performed before computed and digital radiography became broadly used. We believe this study is the first to survey radiation doses in pediatric chest radiograph following the adoption of computed and digital radiography based on the nationwide data from a large number of institutes.
This nationwide study showed that the mean exposure condition for pediatric chest radiographs was 94.9 kVp and 4.30 mAs, with a mean ESD of 140.4 µGy. Generally, compared to film-screen systems, computed and digital radiography machines yield quality images at lower radiation doses (13, 14). Although not statistically significant, mean radiation dose of digital radiography was lower than that of non-digital radiography machines. This can be explained by wide range of SD as have demonstrated on box-plots (Fig. 2), which may consequently led to statistical insignificance.
Our survey found that the third quartile of the radiation dose used for pediatric chest radiograph in Korea is 160.8 µGy. This is lower than the dose recommended by Japanese guidelines (200 µGy) (19), but higher than that of the European Union (100 µGy) (10). Despite international efforts to reduce radiation dose in diagnostic procedures, computed and digital radiography have potential to increase radiation dose through a "dose creep" phenomenon. Higher positron efficiency and wide range of latitude of digital radiography machines may actually inhibit image and dose feedback, and can inadvertently lead to unnecessarily high radiation doses (9-11, 13, 14). In this ironic situation, the wide latitude of both computed and digital radiography systems provides relatively constant image quality throughout variable exposure conditions so that unjustified high radiation dose may pass unnoticed. Since the ESD of the current study exceeds the recommended dose of the European Union established before 2000, we presume "dose creep" phenomenon may have played a role. Also, as demonstrated in our study, images obtained with a low radiation dose also had good diagnostic value. Therefore, our study successfully showed that there is a potential for reducing the radiation doses in pediatric radiograph.
Although there was no significant dose reduction in dedicated pediatric radiography machines attended by pediatric radiologists, SD is much smaller in the dedicated machine. Thus, good quality control is a feature of the dedicated machine. Increasing the number of dedicated machines may help lower the radiation dose.
Among related factors, application of AEC significantly reduced the radiation dose. Since relatively newer machines are equipped with AEC, it is likely that manual exposure controls are set at higher radiation doses. Therefore, proper control of exposure as well as the use of AEC can reduce unnecessary radiation to pediatric patients.
Although the radiography machine's years of operation has been reported to affect radiation dose in adult patients, we found that manufacture date was unrelated to radiation dose in pediatric patients. Several factors may explain this discrepancy. First, since a relatively lower radiation dose is needed for diagnostic pediatric chest radiographs, manufacture date did not affect image quality. Second, the large SD in radiation dose caused by variable exposure conditions and the presence of AEC may have masked the effect of manufacture date. Third, good maintenance of these radiography machines through regular quality control may have prevented the machines from deterioration.
Although the average ESD was overall significantly lower in machines equipped with AEC, the actual contribution of AEC in keeping the ESD under certain level of exposure was not very clear in the beginning of our study. Therefore, we felt the need to set a baseline value to divide the radiography machines into two groups, and to compare the specification of the machines in each group. We chose 100 µGy as a threshold value. This value was the previously recommended dose for pediatric chest radiograph by EU (10), and also was the approximate median value of our study. As expected, a significantly larger proportion of the AEC-equipped machines were in low dose group than in the high dose group. This finding further points up the contribution of AEC in lowering the radiation exposure.
A recommended dose for a radiologic study is usually expressed in DRL. This reference value is set to provide the lowest possible radiation dose that can produce images of sufficient diagnostic value. It is usually the third quartile value of the overall distribution of radiation doses to be applied in real practice (15, 16). In our study, the third quartile of overall dose was 160.8 µGy. However, considering that images obtained with low radiation dose also had adequate diagnostic quality, this value is too high to be set as a DRL. It is extremely important to use a radiation dose as low as possible in radiation-sensitive pediatric patients. Therefore, we do not recommend the third quartile value of 160.8 µGy as DRL. As we have discussed, there is still a great potential for reducing the radiation doses in pediatric radiography, and further studies should be performed to provide the ideal value of DRL.
Our study had several limitations. Our analysis of image quality was limited to penetration quality and did not evaluate visibility of clinically important pulmonary lesions. Also, we could not clearly analyze the clinical quality of the images, including factors such as spatial resolution, visibility of low contrast lesions, and peripheral lung markings owing to the anatomical imperfection of the anthropomorphic phantom.
We are not able to suggest DRL in this study. Although DRL is an advisory value and is neither a limit nor ideal radiation dose, it is important since it provides general target dose without degrading the image quality (17). Therefore, abiding by the DRL is even more important in the era of computed and digital radiography due to their wide latitude and potential risk of overexposure to radiation (18). Although there is virtually no previously stated DRL based on the dose data of computed and digital radiography, we concluded the third quartile value in our study is too high. Further study should be conducted to set a DRL of a reasonably achievable value that can prevent unjustified radiation exposure.
In conclusion, our nationwide survey showed that the third quartile, median, and mean ESD for pediatric chest radiograph is 160.8 µGy, 93.4 µGy, and 140.4 µGy, respectively. These values are somewhat higher than previous reports, and the "dose creep" phenomenon may have occurred. Use of AEC significantly reduced the radiation dose. The lowest possible doses of pediatric chest radiographs can be achieved by using appropriate examination protocols and by implementing expert's quality control.
Figures and Tables
Fig. 1
Dose distribution.
A. Distribution of radiation dose and number of machines allocated in each range of dose. B. Radiation dose distribution of machines. Yellow line indicates median value of overall distribution and red line indicates third quartile.
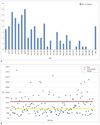
Fig. 2
Boxplots comparing radiography system, AEC, dedicated pediatric radiography machines and pediatric radiologists for ESD (µGy). ESD = entrance surface dose, AEC = automatic exposure control
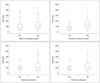
Fig. 3
Scattergram of machine's years of operation and ESD (*r = -0.24, p = 0.783). Pearson correlation coefficient. ESD = entrance surface dose
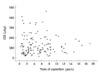
References
1. Doody MM, Lonstein JE, Stovall M, Hacker DG, Luckyanov N, Land CE. Breast cancer mortality after diagnostic radiography: findings from the U.S. Scoliosis Cohort Study. Spine (Phila Pa 1976). 2000. 25:2052–2063.
2. Tanimura M, Matsui I, Abe J, Ikeda H, Kobayashi N, Ohira M, et al. Increased risk of hepatoblastoma among immature children with a lower birth weight. Cancer Res. 1998. 58:3032–3035.
3. Shu XO, Potter JD, Linet MS, Severson RK, Han D, Kersey JH, et al. Diagnostic X-rays and ultrasound exposure and risk of childhood acute lymphoblastic leukemia by immunophenotype. Cancer Epidemiol Biomarkers Prev. 2002. 11:177–185.
4. Lee YJ, Chung YE, Lim JS, Kim JH, Kim YJ, Lee HJ, et al. Cumulative radiation exposure during follow-up after curative surgery for gastric cancer. Korean J Radiol. 2012. 13:144–151.
5. Goo HW. CT radiation dose optimization and estimation: an update for radiologists. Korean J Radiol. 2012. 13:1–11.
6. Goo HW. Pediatric CT: understanding of radiation dose and optimization of imaging techniques. J Korean Radiol Soc. 2005. 52:1–5.
7. Yang DH, Goo HW. Pediatric 16-slice CT protocols: radiation dose and image quality. J Korean Radiol Soc. 2008. 59:333–347.
8. Brenner DJ. Estimating cancer risks from pediatric CT: going from the qualitative to the quantitative. Pediatr Radiol. 2002. 32:228–231. discussion 242-244.
9. Hart D, Wall BF, Shrimpton PC, Bungay DR, Dance CR. Reference doses and patient size in pediatric radiology. 2003. Chilton: National Radiological Protection Board Report 2000 R318, NRPB.
10. EC Study Group. European guidelines on quality criteria for diagnostic radiographic images in pediatrics. 1996. Luxemburg: 1996 EUR 16261.
11. United Nations Scientific Committee on the Effects of Atomic Radiation (UNSCEAR). Sources and Effects of Ionizing Radiation. 2000. New York: 2000 United Nations.
12. Jung YY, Goo HW. The optimal parameter for radiation dose in pediatric low dose abdominal CT: cross-sectional dimensions versus body weight. J Korean Radiol Soc. 2008. 58:169–175.
13. Don S. Radiosensitivity of children: potential for overexposure in CR and DR and magnitude of doses in ordinary radiographic examinations. Pediatr Radiol. 2004. 34:Suppl 3. S167–S172. discussion S234-S241.
14. Huda W. Assessment of the problem: pediatric doses in screen-film and digital radiography. Pediatr Radiol. 2004. 34:Suppl 3. S173–S182. discussion S234-S241.
15. Recommendations of the International Commission on Radiological Protection. ICRP Publication 60. Annals of the ICRP 21, No. 1-3. 1991. Oxford: Pergamon Press.
16. Radiological protection and safety in medicine. ICRP Publication 73. Annals of the ICRP 26, No. 2. 1996. Oxford: Pergamon Press.
17. Schaefer-Prokop C, Neitzel U, Venema HW, Uffmann M, Prokop M. Digital chest radiography: an update on modern technology, dose containment and control of image quality. Eur Radiol. 2008. 18:1818–1830.
18. Uffmann M, Schaefer-Prokop C. Digital radiography: the balance between image quality and required radiation dose. Eur J Radiol. 2009. 72:202–208.
19. Japanese Association of Radiological Technologists. "Guideline 2006". Available at: http://www.jart.jp/guideline/index.html.