Abstract
Objective
The aim of this study was to evaluate the usefulness of combined fluoroscopy- and CT-guided transthoracic needle biopsy (FC-TNB) using a cone beam CT system in comparison to fluoroscopy-guided TNB (F-TNB).
Materials and Methods
We retrospectively evaluated 74 FC-TNB cases (group A) and 97 F-TNB cases (group B) to compare their respective diagnostic accuracies according to the size and depth of the lesion, as well as complications, procedure time, and radiation dose.
Results
The sensitivity for malignancy and diagnostic accuracy for small (< 30 mm in size) and deep (≥ 50 mm in depth) lesions were higher in group A (91% and 94%, 92% and 94%) than in group B (73% and 81%, 84% and 88%), however not statistically significant (p > 0.05). Concerning lesions ≥ 30 mm in size and < 50 mm in depth, both groups displayed similar results (group A, 91% and 92%, 80% and 87%; group B, 90% and 92%, 86% and 90%). Pneumothorax occurred 26% of the time in group A and 14% for group B. The mean procedure time and patient skin dose were significantly higher in group A (13.6 ± 4.0 minutes, 157.1 ± 76.5 mGy) than in group B (9.0 ± 3.5 minutes, 21.9 ± 15.2 mGy) (p < 0.05).
Transthoracic needle biopsy (TNB) under image guidance has been a highly accurate tool for diagnosing pulmonary lesions with a relatively low complication rate (1-5). TNB can be performed under various image guidance including fluoroscopy, CT, and ultrasonography. The decision as to which technique to use usually depends on the characteristics of pulmonary lesions such as size and location, as well as a radiologists' preference or accessibility to imaging systems.
Computed tomography (CT) or CT fluoroscopy has become the most accurate and widely accepted method of image guidance for TNB. However, conventional fluoroscopy-guided TNB (F-TNB) remains a valuable alternative, because of its short procedure time, lower cost, and lower radiation dose.
State-of-the art C-arm cone beam CT system is a form of flat-panel volume CT, in which a cone-beam X-ray tube and a flat-panel detector are integrated with a C-arm gantry. They have both real time fluoroscopic and CT imaging capabilities, enabling both CT-TNB and F-TNB (6).
In this study, we have developed a new biopsy technique; by combining fluoroscopy- and CT-guided TNB (FC-TNB), in which a biopsy is performed under alternative fluoroscopy and cone beam CT guidance using a C-arm cone beam CT system in order to take the advantages of both F-TNB and CT-TNB. Lastly, we evaluated the efficacy and safety of this technique compared with F-TNB.
At our institution, the use of TNB using C-arm cone beam CT system began in January 2009, and a total 74 FC-TNB cases and 17 F-TNB cases for pulmonary lesions using this system were performed until August 2009 by one experienced thoracic radiologist (14 years of experience with TNB).
Two hundred and eighty five cases of F-TNB were performed on 267 patients between July 2005 and December 2008 by three radiologists, and of these patients, 80 were performed by the same radiologist who performed FC-TNB. The cases of F-TNB performed by the other two thoracic radiologists with different biopsy techniques and types of needle were not included in this study.
We retrospectively evaluated 74 FC-TNB cases performed on 68 patients (M:F = 37:31; age range, 27-87 years; average age, 62.0 years) (group A) and 97 F-TNB cases performed on 90 patients (M:F = 59:31; age range, 31-91 years; average age, 52.1 years) (group B) by one radiologist.
For the pulmonary lesions only, which were visible on fluoroscopy, F-TNB or FC-TNB was performed. Indications for FC-TNB included pulmonary lesions which were small (less than 30 mm), deeply located from the chest wall (more than 5 cm in length), located posterior to the heart, attached to the mediastinum or hilum, or necrotic masses which need specific target areas for biopsy. Our Institutional Review Board approved this retrospective study and waived informed patient consent.
A digital fluoroscopy unit (Integris C2000, Philips Medical Systems, Best, The Netherlands) was used for F-TNB from July 2005 and December 2008 and a C-arm cone-beam CT system (AXIOM Artis dBA [VB31E], Siemens Medical Solutions, Forchheim, Germany) for both FC-TNB and F-TNB from January 2009 on.
All TNB was done using a 20-gauge automated cutting needle without a coaxial technique.
The procedure for FC-TNB was performed under alternative fluoroscopy and under cone beam CT guidance. For the first step, a metallic line marker was placed on an expected cutaneous entry point of the thorax under fluoroscopy. Then, a cone-beam CT scan was performed, and the exact entrance point and puncture depth of the needle were determined based on the analysis of multiplanar reformation CT images rendered on a computer workstation. The last step involved a biopsy which was done using a 20-gauge automated cutting needle without a coaxial technique under fluoroscopic guidance (Fig. 1).
Cone-beam CT was scanned with 0.36 µGy per pulse, 30 pulse per sec, and a scan time of 8 sec. Post-processing of the image data to a volume dataset was performed on a multimodality workplace (syngo MMWP software, series number 11690), and the images were subsequently transferred onto a computer workstation (Leonardo, Siemens Medical System, Germany) and analyzed using the multiplanar reformation technique.
Radiation doses and procedure time were measured for cases of FC-TNB and F-TNB which were performed using the C-arm cone beam CT system. Patient skin doses (mGy) were automatically measured by AXIOM Artis. The biopsy procedure time was measured from the attachment of the radiopaque line marker to the patient's skin under fluoroscopic guidance, to the removal of the needle. On-site cytopathologic reviews were not performed during the procedure and pneumothorax was assessed on a chest radiograph obtained within 1-4 hours after a biopsy.
The size and depth of the lesion were evaluated on CT scans, which were obtained 1 day-1 month before TNB. Lesion size was defined as the maximum diameter of lesions on lung window setting images (window level, 700 Hounsfield unit [HU]: window width, 1500 HU) for lesion size. The depth of the lesion was measured from the pleural surface to the pulmonary lesion along the planned needle path. Data concerning the biopsy results, complications, and final diagnosis were collected through a review of patient medical records.
Using the final histologic diagnoses and the clinical and radiological course of the diseases as references, we analyzed the sensitivity, specificity, and diagnostic accuracy according to the size and depth of lesions, as well as the overall diagnostic accuracies in both groups. All statistical analyses were performed using SPSS version 17.0 for Windows (SPSS Inc., Chicago, IL). Differences between categorical variables were analyzed by a chi-square test or Fisher's exact test, and those between continuous variables were analyzed using the unpaired Student t test.
The mean skin entrance dose was significantly higher in group A (157.1 ± 76.5 mGy [mean ± SD]; range, 46.3-389.0 mGy) than group B (21.9 ± 15.2 mGy; range, 5.2-58.2 mGy) (p < 0.001).
Although both groups permit biopsies with short procedure times, the mean procedure time was also significantly higher in group A (13.6 ± 4.0 minutes; range, 7-25 minutes) than group B (9.0 ± 3.5 minutes; range, 5-15 minutes) (p < 0.001).
Final diagnoses were made based on the results of TNB (n = 117), operation (n = 12), pleural biopsy (n = 2), open lung biopsy (n = 2), bronchoscopic biopsy (n = 6), sputum cytology (n = 3), biopsy of a metastatic lesion (n = 1), culture of sputum or TNB specimen (n = 17), and clinical follow-up (n = 11).
The final diagnosis revealed 53 malignant and 21 benign cases in group A (Table 1). The FC-TNB results of 53 malignant lesions were malignancy in 48 cases, with five false-negatives. The sensitivity and specificity for the malignant lesions were 91% and 100%, respectively. The biopsy results of the 21 benign lesions were benign in all cases with 100% sensitivity and specificity, and no false-negative results. The overall diagnostic accuracy of FC-TNB was 93% (Table 2). The five false-negative cases included four small nodules (2.1 ± 1.0 cm; range 0.7-3.3 cm) and one large mass (6.7 cm); the biopsy specimens all showed nonspecific inflammation on cytopathological review.
There were 73 malignant and 24 benign cases in group B (Table 3). The F-TNB results of the 73 malignant lesions were malignant in 62 cases, with 11 false-negatives. The sensitivity and specificity for malignant lesions were 85% and 100% in group B, respectively. Both the sensitivity and specificity for benign lesions were 100% with no false-negative results. The overall diagnostic accuracy was 89% (Table 2). The 11 false-negative cases included eight small nodules (2.1 ± 1.0 cm; range 1.0-3.3 cm) and three large masses (7.3 ± 1.3 cm; range 6.2-8.8 cm), and the biopsy results were nonspecific inflammation in six, necrosis in two, and no malignant cells in three.
The sensitivity for malignant lesions and diagnostic accuracy were higher in group A than in group B, however not statistically significant (sensitivity, p = 0.424; diagnostic accuracy, p = 0.428).
The size and depth of lesions are described in Table 4. The mean diameter of the pulmonary lesions was significantly smaller in group A (32 ± 22 mm; 7-75 mm) than group B (43 ± 22 mm; range, 10-95 mm) (p = 0.01). Further, the proportion of lesions < 30 mm is significantly higher in group A (47%) than in group B (32%) (p = 0.041), because biopsies of small or deep lesions were usually performed under combined fluoroscopy and cone-beam CT scan guidance rather than fluoroscopy during the period in which group A patients underwent TNB.
The mean depth of the lesions was significantly larger in group A (58 ± 21 mm; 17-107 mm) than in group B (51 ± 21 mm; 12-105 mm) (p = 0.02). In addition, the proportion of lesions with a depth > 50 mm was significantly higher in group A (69%) than in group B (51%) (p = 0.019).
The sensitivity and diagnostic accuracy for malignant pulmonary lesions < 30 mm in size were higher in group A (91% and 94%) than in group B (73% and 81%), however not statistically significant (sensitivity, p = 0.240; diagnostic accuracy, p = 0.134) (Table 2). Those for pulmonary lesions ≥ 30 mm in size were similar in both groups (group A, 91% and 92%; group B, 90% and 92%).
With respect to lesion depth, the sensitivity and diagnostic accuracy for those ≥ 50 mm in depth were higher in group A (92% and 94%) than in group B (84% and 88%) (p = 0.303), while depths < 50 mm had sensitivity and diagnostic accuracies higher in group B (86% and 90%) than in group A (80% and 87%) (p = 0.679).
Pneumothorax was observed after a biopsy in 19 cases from group A (26%) and 14 cases from group B (14%). Among them, chest tube placement was required in one group A case (1%) and four group B cases (4%). The distribution of the cases of pneumothorax according to the size and depth of lesions is provided in Table 5. Most cases in both groups exhibited lesion depths of > 50 mm (group A, 74%; group B, 93%; p = 0.209). With respect to the relationship between lesion size and pneumothorax, the pneumothorax rate for the biopsy of lesions less than 20 mm in size was significantly lower in the FC-TNB group (1 of 19) than in the F-TNB group (6 of 17) (p = 0.037). There were no other complications that required treatment.
Fluoroscopic guidance has been the traditional imaging technique for percutaneous lung biopsy, but its disadvantages including difficulty in visualizing small lesions less than 10 mm in size or lesions located adjacent to the vascular or mediastinal structures in orthogonal projections, limit the use of F-TNB (7).
CT is increasingly being used in many centers, replacing conventional fluoroscopy, as the primary method of guidance. However, CT cannot provide real time visualization of a needle, therefore, localization of pulmonary lesions in the lower lobes under CT guidance can be time consuming and difficult, particularly in uncooperative patients (2, 3, 8-11). Nowadays, CT fluoroscopy is the preferred method for image guidance for TNB, but it exposes the radiologist to radiation, as the operator is in the room at the time (12-14).
For over a decade, there have been rapid developments and emerging capabilities of the CT technology, with innovative and improving structural designs of the CT scanner. A unique CT scanner design is the flat-panel volume CT using a flat-panel detector. Flat-panel volume CT allows coverage of a large volume per rotation, fluoroscopic and dynamic imaging, and high spatial resolution (6).
C-arm cone beam CT is a system in which a cone-beam X-ray tube and a flat-panel detector are integrated with a C-arm gantry. It has both CT and fluoroscopy image capabilities (6). Therefore, radiologists can choose only fluoroscopy or both fluoroscopy and CT for guidance, according to the characteristics of pulmonary lesions including size, depth, and location. In our institution, since the installment of c-arm cone beam CT system, TNB for small or deep pulmonary lesions has usually been performed under combined fluoroscopy and CT guidance, while for large and superficial lesions, we have used only fluoroscopy guidance. In addition, the great flexibility of the C-arm cone beam CT system in orienting the detector around the patient compared to the closed CT gantries and its simplicity in the acquisition of CT and fluoroscopy images allow for the shortening of procedure time. In this study, the mean procedure time of FC-TNB was 13.6 ± 4.0 minutes, which is comparable to that of CT fluoroscopy-guided TNB (12.3-23.8 min) (12, 15, 16) and much less than that of CT-guided TNB (25.0-26.7 min) (15, 16).
In this study, FC-TNB showed high diagnostic accuracy comparable to CT-TNB, ranging from 83 to 95% (1, 17-19). For small pulmonary lesions, in a series of CT-guided biopsies, vanSonnenberg et al. (5) reported a mean diagnostic accuracy of 84% for lesions 1.1-2.0 cm in diameter. Bladt et al. (20) reported that the accuracy of CT-fluoroscopic biopsy for lesions between 10 and 30 mm was 79%. In this study, the diagnostic accuracy of FC-TNB was 90% for lesions less than 20 mm, and 94% for lesions less than 30 mm. Presumably, the lower diagnostic accuracy of CT-guided TNB is explained by the lack of real-time visualization of the needle during the biopsy procedure. Under CT guidance, localization of small pulmonary nodules, particularly those located in the lower lobes and significantly influenced by respiratory movement, can be time-consuming and difficult in uncooperative patients (2, 21).
Although this study showed a higher sensitivity and greater diagnostic accuracy for small and deep lesions in the FC-TNB group than in the F-TNB group, the difference between the methods was not statistically significant. However, this may have been caused by too few false-negative cases to give significance in the statistical analysis, rather than the absence of a significant difference between the two groups.
This study revealed sensitivities of 91% in FC-TNB and 85% in F-TNB, which were lower than reported previously (22, 23). This was presumably related to the small size of the biopsy needle and lack of on-site cytopathological review. In this study, TNB was performed using a 20-gauge needle. Needle size affects the sensitivity of TNB, and the sensitivities reported in series using 18-gauge needles usually exceed 90% and are higher than those of TNB using smaller biopsy needles (1, 10). Ohno et al. (2) and Kurban et al. (24) reported sensitivities of less than 90% in their studies using 22-gauge fine needles. In our study, the false-negative cases could be divided into two groups: small nodules (mean size, 2.1 cm) and large masses (> 6 cm). Small nodules are related to the low sensitivity of TNB (2, 21), and large masses may give false-negative results due to tumor necrosis. In these cases, the quick staining of the specimen during the procedure may reduce false-negative rates.
Limited studies have been performed about the radiation dose of TNB. Teeuwisse et al. (25) reported that the entrance skin dose of CT fluoroscopy-guided TNB (mean, 130 mGy) was significantly lower than that of CT-TNB (330 mGy). In the current study, the mean skin dose of FC-TNB was 157 mGy. For FC-TNB procedure, radiation dose could be reduced by using fluoroscopy guidance instead of CT guidance, in the steps when CT guidance is not necessary.
Compared to F-TNB, FC-TNB showed higher diagnostic accuracy for small (< 30 mm in size) and deep (> 50 mm in depth) lesions. That may stem from FC-TNB allowing a more exact measurement of lesion depth through the use of multiplanar reformation CT images, which has a larger effect on the success of biopsy procedures in small or deeply located pulmonary lesions than in large or superficial lesions. However, for larger and more superficial lesions, F-TNB showed high diagnostic accuracy comparable to that of FC-TNB. In our opinion, considering the very low radiation dose, short procedure time, lower costs, and easy accessibility, F-TNB is still a useful method for these pulmonary lesions (24).
The pneumothorax rate in our series of FC-TNB was 26%, which falls within the previously reported ranges for CT-TNB: 13-45% (1, 2, 10) and CTF: 22-32% (1, 12). Factors contributing to an increased risk of pneumothorax include preexisting lung disease, increased number of needle passes, increased lesion depth, increasing patient age, and needle size (2, 8, 26, 27). In the current study, the pneumothorax rate was mainly affected by the needle path length. Most cases of pneumothorax from both groups had a sizeable lesion depth of more than 50 mm.
In this study, the higher incidence of pneumothorax after FC-TNB compared to F-TNB may be explained by a significantly higher number of cases with sizeable lesion depths in the FC-TNB group. The pneumothorax rate with respect to the relationship between lesion size and pneumothorax for the biopsy of lesions less than 20 mm in size was significantly lower in the FC-TNB group than in the F-TNB group; perhaps indicating that FC-TNB may reduce pneumothorax in cases with small pulmonary lesions. That may be explained through the accurate localization of pulmonary lesions and precisely measuring lesion depth using cone-beam CT images, thereby avoiding the biopsy of unnecessary normal tissue as well as reducing the number of needle passes and puncture times.
This study has some limitations. First, there were significant differences in the size and depth of lesions in both groups. The FC-TNB group included a significantly larger number of lesions of small size and large depth, and therefore, the overall diagnostic accuracy of FC-TNB may underestimate that of the general population undergoing biopsy. Second, another limitation of this retrospective study was that the number of needle passes per biopsy, which is an important factor for the occurrence of complications after TNB, was not compared between the two groups in this study because it was not recorded for most F-TNB cases.
In conclusion, the C-arm cone beam system enables both FC-TNB and F-TNB. FC-TNB allows biopsy of small (< 30 mm) and deep (≥ 50 mm) lesions with high diagnostic accuracy and short procedure time, and F-TNB is still a useful method for larger and more superficial lesions with a very small radiation dose.
Figures and Tables
Fig. 1
Combined fluoroscopy- and CT-guided transthoracic needle biopsy technique using C-arm cone beam CT system.
A. For first step, metallic line marker was attached to patient's skin, covering anticipated entrance point of needle, under fluoroscopy guidance. B. CT scan was performed, and exact entrance point and puncture depth of needle were determined based on analysis of multiplanar reformation images on computer workstation. C. Last step involved transthoracic needle biopsy under fluoroscopy guidance.
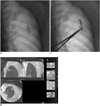
Table 1
Results of Combined Fluoroscopy- and CT-Guided Transthoracic Needle Biopsy as well as Final Diagnosis for Group A Patients
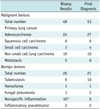
Table 2
Sensitivity of Malignancy and Overall Diagnostic Accuracy according to Characteristics of Pulmonary Lesions
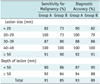
Table 3
Results of Fluoroscopy-Guided Transthoracic Needle Biopsies and Final Diagnoses in Group B Patients
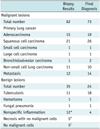
References
1. Heck SL, Blom P, Berstad A. Accuracy and complications in computed tomography fluoroscopy-guided needle biopsies of lung masses. Eur Radiol. 2006. 16:1387–1392.
2. Ohno Y, Hatabu H, Takenaka D, Higashino T, Watanabe H, Ohbayashi C, et al. CT-guided transthoracic needle aspiration biopsy of small (< or = 20 mm) solitary pulmonary nodules. AJR Am J Roentgenol. 2003. 180:1665–1669.
3. Li H, Boiselle PM, Shepard JO, Trotman-Dickenson B, McLoud TC. Diagnostic accuracy and safety of CT-guided percutaneous needle aspiration biopsy of the lung: comparison of small and large pulmonary nodules. AJR Am J Roentgenol. 1996. 167:105–109.
4. Westcott JL. Direct percutaneous needle aspiration of localized pulmonary lesions: result in 422 patients. Radiology. 1980. 137:31–35.
5. vanSonnenberg E, Casola G, Ho M, Neff CC, Varney RR, Wittich GR, et al. Difficult thoracic lesions: CT-guided biopsy experience in 150 cases. Radiology. 1988. 167:457–461.
6. Gupta R, Cheung AC, Bartling SH, Lisauskas J, Grasruck M, Leidecker C, et al. Flat-panel volume CT: fundamental principles, technology, and applications. Radiographics. 2008. 28:2009–2022.
7. Aviram G, Schwartz DS, Meirsdorf S, Rosen G, Greif J, Graif M. Transthoracic needle biopsy of lung masses: a survey of techniques. Clin Radiol. 2005. 60:370–374.
8. Geraghty PR, Kee ST, McFarlane G, Razavi MK, Sze DY, Dake MD. CT-guided transthoracic needle aspiration biopsy of pulmonary nodules: needle size and pneumothorax rate. Radiology. 2003. 229:475–481.
9. Haaga JR, Reich NE, Havrilla TR, Alfidi RJ, Meaney TF. CT guided biopsy. Cleve Clin Q. 1977. 44:27–33.
10. Kinoshita F, Kato T, Sugiura K, Nishimura M, Kinoshita T, Hashimoto M, et al. CT-guided transthoracic needle biopsy using a puncture site-down positioning technique. AJR Am J Roentgenol. 2006. 187:926–932.
11. Ohno Y, Hatabu H, Takenaka D, Imai M, Ohbayashi C, Sugimura K. Transthoracic CT-guided biopsy with multiplanar reconstruction image improves diagnostic accuracy of solitary pulmonary nodules. Eur J Radiol. 2004. 51:160–168.
12. Carlson SK, Felmlee JP, Bender CE, Ehman RL, Classic KL, Hoskin TL, et al. CT fluoroscopy-guided biopsy of the lung or upper abdomen with a breath-hold monitoring and feedback system: a prospective randomized controlled clinical trial. Radiology. 2005. 237:701–708.
13. Heck SL, Blom P, Berstad A. Accuracy and complications in computed tomography fluoroscopy-guided needle biopsies of lung masses. Eur Radiol. 2006. 16:1387–1392.
14. Irie T, Kajitani M, Matsueda K, Arai Y, Inaba Y, Kujiraoka Y, et al. Biopsy of lung nodules with use of I-I device under intermittent CT fluoroscopic guidance: preliminary clinical study. J Vasc Interv Radiol. 2001. 12:215–219.
15. Carlson SK, Bender CE, Classic KL, Zink FE, Quam JP, Ward EM, et al. Benefits and safety of CT fluoroscopy in interventional radiologic procedures. Radiology. 2001. 219:515–520.
16. Froelich JJ, Ishaque N, Regn J, Saar B, Walthers EM, Klose KJ. Guidance of percutaneous pulmonary biopsies with real-time CT fluoroscopy. Eur J Radiol. 2002. 42:74–79.
17. Gianfelice D, Lepanto L, Perreault P, Chartrand-Lefebvre C, Milette PC. Value of CT fluoroscopy for percutaneous biopsy procedures. J Vasc Interv Radiol. 2000. 11:879–884.
18. Yamagami T, Iida S, Kato T, Tanaka O, Toda S, Kato D, et al. Usefulness of new automated cutting needle for tissue-core biopsy of lung nodules under CT fluoroscopic guidance. Chest. 2003. 124:147–154.
19. Tsukada H, Satou T, Iwashima A, Souma T. Diagnostic accuracy of CT-guided automated needle biopsy of lung nodules. AJR Am J Roentgenol. 2000. 175:239–243.
20. Bladt O, De Wever W. Additional value of CT-fluoroscopic biopsy of pulmonary lesions: a retrospective study of 69 patients. JBR-BTR. 2006. 89:298–302.
21. Li H, Boiselle PM, Shepard JO, Trotman-Dickenson B, McLoud TC. Diagnostic accuracy and safety of CT-guided percutaneous needle aspiration biopsy of the lung: comparison of small and large pulmonary nodules. AJR Am J Roentgenol. 1996. 167:105–109.
22. Khouri NF, Stitik FP, Erozan YS, Gupta PK, Kim WS, Scott WW Jr, et al. Transthoracic needle aspiration biopsy of benign and malignant lung lesions. AJR Am J Roentgenol. 1985. 144:281–288.
23. Sagel SS, Ferguson TB, Forrest JV, Roper CL, Weldon CS, Clark RE. Percutaneous transthoracic aspiration needle biopsy. Ann Thorac Surg. 1978. 26:399–405.
24. Kurban LA, Gomersall L, Weir J, Wade P. Fluoroscopy-guided percutaneous lung biopsy: a valuable alternative to computed tomography. Acta Radiol. 2008. 49:876–882.
25. Teeuwisse WM, Geleijns J, Broerse JJ, Obermann WR, van Persijn van Meerten EL. Patient and staff dose during CT guided biopsy, drainage and coagulation. Br J Radiol. 2001. 74:720–726.
26. Manhire A, Charig M, Clelland C, Gleeson F, Miller R, Moss H, et al. Guidelines for radiologically guided lung biopsy. Thorax. 2003. 58:920–936.
27. Khan MF, Straub R, Moghaddam SR, Maataoui A, Gurung J, Wagner TO, et al. Variables affecting the risk of pneumothorax and intrapulmonal hemorrhage in CT-guided transthoracic biopsy. Eur Radiol. 2008. 18:1356–1363.