Abstract
Objective
We wanted to evaluate the effect of the number of diffusion-sensitizing gradient directions on the image quality for evaluating myocardial anisotropy and fiber tracking by using in vitro diffusion tensor MR imaging (DT-MRI).
Materials and Methods
The DT-MR images, using a SENSE-based echoplanar imaging technique, were acquired from ten excised porcine hearts by using a 3T MR scanner. With a b-value of 800 s/mm2, the diffusion tensor images were obtained for 6, 15 and 32 diffusion-sensitizing gradient directions at the midventricular level. The number of tracked fibers, the fractional anisotropy (FA), and the length of the tracked fibers were measured for the quantitative analysis. Two radiologists assessed the image quality of the fiber tractography for the qualitative analysis.
Results
By increasing the number of diffusion-sensitizing gradient directions from 6 to 15, and then to 32, the FA and standard deviation were significantly reduced (p < 0.01), and the number of tracked fibers and the length of the tracked fibers were significantly increased (p < 0.01). The image quality of the fiber tractography was significantly increased with the increased number of diffusion-sensitizing gradient directions (p < 0.01).
It is well known that the three-dimensional organization of the myocardial fiber structure is a key determinant of ventricular torsion, strain and stress (1, 2). When viewed from the apex, the orientation of the left ventricular (LV) myocardial fibers changes smoothly from a left-handed helix in the epicardium to a right-handed helix in the subendocardium (3, 4).
Diffusion tensor MR imaging (DT-MRI) has provided a means for rapidly and noninvasively evaluating the cardiac geometry and the three-dimensional fiber structure (5-8). It has been shown that there is a direct correlation between DT-MRI and the histological fiber directions, and this has validated this approach for characterizing the myocardial fiber orientation (9-11). From a clinical point of view, understanding the altered tissue integrity and fiber architecture in diseased myocardium, such as in a patient who has suffered a myocardial infarction, is critically important because it might shed light on the mechanism of structure-function remodeling after myocardial infarction (12).
Theoretically, a higher number of diffusion-sensitizing gradient directions yields more accurate diffusion tensor information (13). However, most cardiac DT-MRI studies have used low diffusion resolution such as 6 directions, which has have been arbitrarily proposed for examining an animal's fixed heart. To the best of our knowledge, there has been no systematic study for determining the effect of the number of diffusion-sensitizing gradient directions through the DT-MRI acquisition schemes that are used for the image quality of cardiac DT-MRI. There is only one report on the utility of DT-MRI with medium diffusion resolution with using 15 diffusion-sensitizing gradient directions (14). Furthermore, the use of high-field-strength MR imaging in clinical settings has become practical with the recent advances in actively shielded 3T magnets (15, 16). Therefore, the purpose of this study is to evaluate the effect of the number of diffusion-sensitizing gradient directions on the image quality for the evaluation of myocardial anisotropy and fiber tracking by using in vitro DT-MRI at 3T.
Our study was performed on the basis of the guidelines set both by the U.S. National Institute of Health and the recommendations of the Committee on Animal Research at our institution. The study protocol was approved by the local Institutional Review Committee on Animal Care. The heart specimens were obtained from a separate study. Ten pigs (n = 10, 30-35 kg) were subjected to 90 minutes of occlusion of the left anterior descending coronary artery, and this was followed by 90 minutes of reperfusion. After performing a left lateral thoracotomy along the fifth intercostal space, the left anterior descending coronary artery was isolated distal to the first diagonal branch, and a snare loop made with 4-0 silk was placed in a slender plastic tube. Occlusion or reperfusion of the left anterior descending coronary artery was produced by simply fastening or releasing the snare loop. This procedure was previously described in detail (17, 18). The hearts were rapidly excised and placed in a bath of cold (4℃) cardioplegic solution. The hearts were stored in formalin solution for at least 18 hour to let the possible early ventricular geometry changes occur before MR imaging, as was previously suggested (9, 14).
The DT-MRI were acquired from the ten excised hearts by using a Philips 3T Achieva scanner (Philips Medical System, Best, Netherlands) and an eight-channel head coil. The formalin-fixed porcine heart specimens were suspended in a cylinder filled with formalin to avoid tissue-air susceptibility artifacts. After the scout images were acquired on the four-chamber and two-chamber views, the short axis images of the left ventricle were obtained at the midventricular level for DT-MRI.
The sensitivity encoding (SENSE)-based echo-planar imaging technique was applied to shorten the image acquisition time and to alleviate any image distortion caused by susceptibility artifacts from the single-shot echoplanar imaging sequences. The SENSE factor was chosen to be 2.4 in this study as a trade off between image distortion due to the high echo-planar imaging factor and the SENSE artifacts caused by a high SENSE factor, as was previously suggested (14).
With a b-value of 800 s/mm2, the diffusion tensor images were obtained, respectively, for 6, 15 and 32 diffusion gradient directions at the same midventricular level of each specimen. The imaging parameters were as follows: TE = 55 ms, TR = 5000 ms, number of slices = 5, slice thickness = 1.13 mm, slice gap = 0 mm and the number of excitations = 1.
Three-dimensional reconstruction of the myocardial fibers was done by using the commercially available PRIDE software package (Philips Medical Systems, Best, Netherlands). For each of the short-axis slices, the right ventricles were removed in the images and then excluded from the analysis. The numbers of tracked fibers, the fractional anisotropy (FA) and the length of the tracked fibers in the left ventricle were measured using the PRIDE software by an experienced cardiac radiologist who has 12 years of experience with quantitative analysis. The fiber distribution was investigated with the FA magnitude and directional thresholds set at 0.15 and 40°, respectively (14).
The image quality of the fiber tractography was assessed by two radiologists for qualitative analysis. They scored each image on a 3-point scale: 1 (poor), 2 (moderate) and 3 (good). The definition of each rating score is as follows: 'poor' was visualization of the myocardium with frequent discontinuity except for in the infarcted myocardium; 'moderate' was visualization of the myocardium with partial discontinuity except for in the infarcted myocardium; 'excellent' was visualization of myocardial fibers without or with minimal discontinuity except in the infarcted myocardium. The average of the scores for each image was used for qualitative analysis. For statistical analysis among the three paired data sets, all quantitative and qualitative comparisons were analyzed by using Friedmann's test and the Wilcoxon-signed rank test for post-hoc consideration. P values < 0.05 were considered significant. SPSS version 12.0 (SPSS, Chicago, IL) was used for the statistical calculation. The data is presented as means ± standard deviations (SDs).
The results of the quantitative analysis are summarized in Table 1. By increasing the numbers of diffusion-sensitizing gradient directions from 6 to 15 and to 32 on DT-MRI, the mean FA and standard deviation were significantly reduced (p < 0.01). For the evaluation of fiber tracking, the number of tracked fibers was significantly increased (p < 0.01) and the length of the tracked fibers was also increased (p < 0.01) with the increased number of the diffusion-sensitizing gradient directions (Figs. 1, 2, 3). In the qualitative analysis, the image quality of the fiber tractography was significantly increased according to the increased number of the diffusion-sensitizing gradient directions (p < 0.01) (Table 2).
Our result showed that higher numbers of diffusion-sensitizing gradient directions can provide more detailed information about the myocardial fiber structure on in vitro DT-MRI at 3T.
To the best our current knowledge, no consensus exists for the optimal number of diffusion-sensitizing gradient directions for DT-MRI. Jones (19) insisted that the mean diffusivity, FA and tensor orientation requires a DT-MRI sampling scheme in which there are at least 30 diffusion-sensitizing gradient directions by using Monte Carlo simulations. Our study also suggested that one should use as many unique sampling orientations as time will allow for applications such as fiber tractography. Lee et al. (13) demonstrated that DT-MRI in the cervical spinal cord with using 15 diffusion-sensitizing gradient directions showed significantly better quality than that using 6 directions. Yet DTI using 32 directions did not show a significant improvement of quality over that using 15 directions. They suggested that the increased numbers of diffusion-sensitizing gradient directions in vivo DT-MRI require longer scan times and there may be a greater risk for motion artifacts.
This is a first report on using a higher number of diffusion-sensitizing gradient directions for cardiac DT-MRI. Most studies have used low diffusion resolution with employing 6 diffusion-sensitizing gradient directions for cardiac DT-MRI. Wu et al. (14) recently used DT-MRI with medium diffusion resolution with employing 15 diffusion-sensitizing gradient directions for the evaluation of myocardial fiber pathways in canine heart samples fixed in formalin. They revealed that the long fiber pathways were found to predominantly run circumferentially with small helix angles, and this dominated the fiber architecture in the myocardium. However, they only investigated the myocardial fiber pathway distribution and they did not analyze the effect of medium diffusion resolution for the DT-MRI quality for the evaluation of myocardial anisotropy and fiber tracking. In this study, we demonstrated the effect of high diffusion resolution by using 32 diffusion-sensitizing gradient directions for in vitro DT-MRI. We suggest that high resolution DT-MRI may be used as a tool for delineating and monitoring the detailed myocardial fiber structure after a myocardial infarction has responded to various treatments such as stem cell therapy.
Although the advantages of MRI at 3T over the 1.5T systems have not been established for the use of cardiac DT-MRI, a better depiction of fiber tracts at 3T compared with 1.5T has been demonstrated with performing high spatial resolution DT-MRI in brain (20-22). The sensitivity-encoded (SENSE) MR technique, as a parallel imaging method, is now feasible with using a commercial machine and with no dedicated hardware. Using the SENSE technique, the duration of the echo train is reduced by faster filling of the k-space and so this results in a wider bandwidth in the phase-encoded direction; therefore, the susceptibility artifacts are reduced (23, 24).
To the best of our knowledge, there is no consensus concerning the optimum method for evaluating the quality of DT-MRI. Naganawa et al. (25) investigated the optimum imaging parameters of DT-MRI in brain, and they quantitatively evaluated the numbers of reconstructed fibers and the visual image quality. In a similar fashion, we evaluated the qualities of the DT-MRI with using different numbers of diffusion-sensitizing gradient directions by quantifying the numbers and length of the tracked fibers, as well as by visually comparing the image quality of the fiber tractography. Our results demonstrated that the numbers and length of the tracked fibers were significantly increased with the increased number of diffusion-sensitizing gradient directions, which suggests that the image quality of the fiber tractography was significantly improved. However, the validity of using fiber tracking as a means to determine the accuracy of DT-MRI measurements was not proven experimentally.
Without loss of generality, the DTI-derived FA index (26), which is a commonly used rotationally invariant index of diffusion anisotropy, is examined as a function of the left ventricular wall depth and the circumferential and longitudinal locations. Jiang et al. (27) reported that the diffusion anisotropy measured by DT-MRI in the fixed sheep myocardium remained relatively constant from the epicardium to the midwall and then it decreased steadily toward the endocardium. Wu et al. (12) also demonstrated that in vivo DT-MRI of the postinfarct myocardium in patients with myocardial infarction revealed a significant decrease in FA, indicating there was altered tissue integrity. Furthermore, the redistribution of the fiber architecture correlated with the infarct size and the left ventricular function. Therefore, exactly measuring the FA is important for characterizing such tissue parameters as the extracellular volume fraction as well as the fiber orientation on cardiac DT-MRI. Chang et al. (28) revealed, by using the histogram method, that the image quality of the FA map of a brain was significantly improved as the number of diffusion-sensitizing gradient directions increased. They also reported that that the histogram showed that the FA values and their variance were increased as the number of diffusion gradient directions decreased, and this was probably due to the overestimation of the FA values. It was also shown in our study that the mean FA and its standard deviation were significantly reduced with the increased number of diffusion-sensitizing gradient directions.
The present study has some limitations. First, we have no direct histopathological correlations to validate our findings. However, it is difficult to precisely quantify the numbers and the length of myocardial fibers on histopathological exam. Second, our study was not performed by using in vivo DT-MRI. The increased scan time may have produced more motion-related artifacts on the in vivo DT-MRI. The scan time of DT-MRI is also limited in most clinical situations. Optimizing the number of diffusion-sensitizing gradient directions should be considered as a tradeoff between increasing the image quality and minimizing the scan time for in vivo DT-MRI. Advanced MR technology will be required to overcome such problems as the low signal-to-noise ratio and the long scan time for in vivo DT-MRI. Third, we did not consider several important parameters such as the b-value, the numbers of excitations and the slice thickness for cardiac DT-MRI. Further study will be needed to combine several individual parameters for optimizing the acquisition of in vivo DT-MRI before it can be applied to clinical settings. Finally, we did not separately evaluate the alterations in tissue integrity, such as the FA of both the infarcted and normal myocardium, because the purpose of our study was only to evaluate the effect of the number of diffusion-sensitizing gradient directions on the image quality of DT-MRI.
From this study, we conclude that the image quality of in vitro DT-MRI is significantly improved as the number of diffusion-sensitizing gradient directions is increased.
Figures and Tables
![]() | Fig. 1Fiber tractographies of oblique view (A, B, C) and anterior-posterior view (D, E, F) on basis of different number of diffusion gradient directions. Note significantly increased number and length of tracked fibers with increased number of diffusion gradient directions. (A, D: n = 6, B, E: n = 15, C, F: n = 32 directions). |
![]() | Fig. 2Color tensor fiber tractographies as based on different number of diffusion gradient directions. (A: n = 6, B: n = 15, C: n = 32 directions). |
![]() | Fig. 3Schematic cross section of heart at midventricular level (A, modified from ref. 3) and corresponding examples of fiber tractography using 32 diffusion gradient directions (B). Both oblique fibers (long arrows) and circumferential fibers (short arrow) are nicely demonstrated on diffusion-tensor image. |
Table 1
Quantitative Analysis of Fractional Anisotropy and Fiber Tracking According to Number of Diffusion Gradient Directions
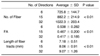
References
1. Rademakers FE, Rogers WJ, Guier WH, Hutchins GM, Siu CO, Weisfeldt ML, et al. Relation of regional cross-fiber shortening to wall thickening in the intact heart. Three-dimensional strain analysis by NMR tagging. Circulation. 1994. 89:1174–1182.
2. Taber LA, Yang M, Podszus WW. Mechanics of ventricular torsion. J Biomech. 1996. 29:745–752.
3. Spotnitz HM. Macrodesign, structure, and mechanics of the left ventricle. J Thorac Cardiovasc Surg. 2000. 119:1053–1057.
4. Streeter DD Jr, Spotnitz HM, Patel DP, Ross J Jr, Sonnenblick EH. Fiber orientation in the canine left ventricle during diastole and systole. Circ Res. 1969. 24:339–347.
5. Wu EX, Wu Y, Nicholls JM, Wang J, Liao S, Zhu S, et al. MR diffusion tensor imaging study of postinfarct myocardium structural remodeling in a porcine model. Magn Reson Med. 2007. 58:687–695.
6. Edelman RR, Gaa J, Wedeen VJ, Loh E, Hare JM, Prasad P, et al. In vivo measurement of water diffusion in the human heart. Magn Reson Med. 1994. 32:423–428.
7. Reese TG, Weisskoff RM, Smith RN, Rosen BR, Dinsmore RE, Wedeen VJ. Imaging myocardial fiber architecture in vivo with magnetic resonance. Magn Reson Med. 1995. 34:786–791.
8. Tseng WY, Reese TG, Weisskoff RM, Wedeen VJ. Cardiac diffusion tensor MRI in vivo without strain correction. Magn Reson Med. 1999. 42:393–403.
9. Holmes AA, Scollan DF, Winslow RL. Direct histological validation of diffusion tensor MRI in formaldehyde-fixed myocardium. Magn Reson Med. 2000. 44:157–161.
10. Scollan DF, Holmes A, Winslow R, Forder J. Histological validation of myocardial microstructure obtained from diffusion tensor magnetic resonance imaging. Am J Physiol. 1998. 275:H2308–H2318.
11. Tseng WY, Wedeen VJ, Reese TG, Smith RN, Halpern EF. Diffusion tensor MRI of myocardial fibers and sheets: correspondence with visible cut-face texture. J Magn Reson Imaging. 2003. 17:31–42.
12. Wu MT, Tseng WY, Su MY, Liu CP, Chiou KR, Wedeen VJ, et al. Diffusion tensor magnetic resonance imaging mapping the fiber architecture remodeling in human myocardium after infarction: correlation with viability and wall motion. Circulation. 2006. 114:1036–1045.
13. Lee JW, Kim JH, Kang HS, Lee JS, Choi JY, Yeom JS, et al. Optimization of acquisition parameters of diffusion-tensor magnetic resonance imaging in the spinal cord. Invest Radiol. 2006. 41:553–559.
14. Wu EX, Wu Y, Tang H, Wang J, Yang J, Ng MC, et al. Study of myocardial fiber pathway using magnetic resonance diffusion tensor imaging. Magn Reson Imaging. 2007. 25:1048–1057.
15. Tanenbaum LN. 3-T MR imaging: ready for clinical practice. AJNR Am J Neuroradiol. 2004. 25:1626–1627.
16. Zhai G, Lin W, Wilber KP, Gerig G, Gilmore JH. Comparisons of regional white matter diffusion in healthy neonates and adults performed with a 3.0-T head-only MR imaging unit. Radiology. 2003. 229:673–681.
17. Choi SI, Choi SH, Kim ST, Lim KH, Lim CH, Gong GY, et al. Irreversibly damaged myocardium at MR imaging with a necrotic tissue-specific contrast agent in a cat model. Radiology. 2000. 215:863–868.
18. Jeong AK, Choi SI, Kim DH, Park SB, Lee SS, Choi SH, et al. Evaluation by contrast-enhanced MR imaging of the lateral border zone in reperfused myocardial infarction in a cat model. Korean J Radiol. 2001. 2:21–27.
19. Jones DK. The effect of gradient sampling schemes on measures derived from diffusion tensor MRI: a Monte Carlo study. Magn Reson Med. 2004. 51:807–815.
20. Okada T, Miki Y, Fushimi Y, Hanakawa T, Kanagaki M, Yamamoto A, et al. Diffusion-tensor fiber tractography: intraindividual comparison of 30-T and 15-T MR imaging. Radiology. 2006. 238:668–678.
21. Jaermann T, Crelier G, Pruessmann KP, Golay X, Netsch T, van Muiswinkel AM, et al. SENSE-DTI at 3 T. Magn Reson Med. 2004. 51:230–236.
22. Nagae-Poetscher LM, Jiang H, Wakana S, Golay X, van Zijl PC, Mori S. High-resolution diffusion tensor imaging of the brain stem at 3 T. AJNR Am J Neuroradiol. 2004. 25:1325–1330.
23. Pruessmann KP, Weiger M, Scheidegger MB, Boesiger P. SENSE: sensitivity encoding for fast MRI. Magn Reson Med. 1999. 42:952–962.
24. Cercignani M, Horsfield MA, Agosta F, Filippi M. Sensitivity-encoded diffusion tensor MR imaging of the cervical cord. AJNR Am J Neuroradiol. 2003. 24:1254–1256.
25. Naganawa S, Koshikawa T, Kawai H, Fukatsu H, Ishigaki T, Maruyama K, et al. Optimization of diffusion-tensor MR imaging data acquisition parameters for brain fiber tracking using parallel imaging at 3 T. Eur Radiol. 2004. 14:234–238.
26. Pierpaoli C, Basser PJ. Toward a quantitative assessment of diffusion anisotropy. Magn Reson Med. 1996. 36:893–906.
27. Jiang Y, Guccione JM, Ratcliffe MB, Hsu EW. Transmural heterogeneity of diffusion anisotropy in the sheep myocardium characterized by MR diffusion tensor imaging. Am J Physiol Heart Circ Physiol. 2007. 293:H2377–H2384.
28. Chang Y, Lee YJ, Kim YS, Kang DS, Sohn CH, Woo SK, et al. Histogram analysis of noise performance on fractional anisotropy brain MR image with different diffusion gradient numbers. J Korean Radiol Soc. 2005. 52:87–92. [Korean].