Abstract
Objective
The purpose of this study was to compare the antitumor effect and hepatotoxicity of an intraarterial delivery of low-dose and high-dose 3-bromopyruvate (3-BrPA) and those of a conventional Lipiodol-doxorubicin emulsion in a rabbit VX2 hepatoma model.
Materials and Methods
This experiment was approved by the animal care committee at our institution. VX2 carcinoma was implanted in the livers of 36 rabbits. Transcatheter intraarterial administration was performed using low dose 3-BrPA (25 mL in a 1 mM concentration, n = 10), high dose 3-BrPA (25 mL in a 5 mM concentration, n = 10) and Lipiodol-doxorubicin emulsion (1.6 mg doxorubicin/ 0.4 mL Lipiodol, n = 10), and six rabbits were treated with normal saline alone as a control group. One week later, the proportion of tumor necrosis was calculated based on histopathologic examination. The hepatotoxicity was evaluated by biochemical analysis. The differences between these groups were statistically assessed with using Mann-Whitney U tests and Kruskal-Wallis tests.
Results
The tumor necrosis rate was significantly higher in the high dose group (93% ± 7.6 [mean ± SD]) than that in the control group (48% ± 21.7) (p = 0.0002), but the tumor necrosis rate was not significantly higher in the low dose group (62% ± 20.0) (p = 0.2780). However, the tumor necrosis rate of the high dose group was significantly lower than that of the Lipiodol-doxorubicin treatment group (99% ± 2.7) (p = 0.0015). The hepatotoxicity observed in the 3-BrPA groups was comparable to that of the Lipiodol-doxorubicin group.
Hepatocellular carcinoma (HCC) is one of the most common malignant tumors in the world and the estimated number of cancer-related deaths currently exceeds 500,000 per year (1, 2). Although surgery is the preferred treatment for HCC, only 10-20% of all patients with HCC are suitable candidates for either hepatic resection or transplantation because of tumor extension, poor hepatic function or both (3-5). Among several therapeutic options that have been advocated for the treatment of unresectable HCC, transcatheter arterial chemoembolization (TACE) has been widely performed and various cytotoxic agents have been used, including doxorubicin, cisplatin and 5-fluorouracil. However the therapeutic efficacy of TACE is still limited and the overall results of treatments for HCC remain unsatisfactory (6). Therefore, a new therapeutic strategy is needed for the management of HCC.
One of the most common and profound biochemical phenotypes of animal and human cancer cells is their ability to metabolize glucose at high rates, and even under aerobic conditions (7-10). In contrast to normal cells, tumor cells are mainly dependent on the glycolysis pathway for meeting their adenosine triphosphate (ATP) requirements. Moreover, glycolytic intermediates are important precursors for the biosynthesis of lipids and nucleic acids that are essential for cell growth and division (11). Hexokinase is one of the most important enzymes for maintaining a high rate of glycolytic energy-production. This enzyme phosphorylates the incoming glucose to glucose-6-phosphate, thus trapping the substrate intracellularly and committing it to further glycolysis. Among the four-hexokinase isotypes (hexokinase I-IV) in mammalian tissues (12), hexokinase II, which is located on the outer mitochondrial membrane, is frequently the predominant overexpressed form in malignant cells, including HCC (13-16), and hexokinase II normally exists in adipose and muscle tissues (12, 17).
3-bromopyruvate (3-BrPA) is a pyruvate analog and a strong alkylating agent, and it was recently found to be a potent inhibitor of glycolysis and oxidative phosphorylation by targeting hexokinase II in in vitro experiments with using rat AS-30D cells and rabbit VX2 carcinoma cells (18-22). In an in vivo experiment using a VX2 carcinoma cells and AS-30D cells implanted in rabbit's liver and rats' abdomens, respectively, the intraarterial, intravenous and intraperitoneal delivery of 3-BrPA has showed significant anticancer effects without damage to normal tissues (19,22). Therefore, the blockage of energy metabolism by hexokinase II inhibitors seems to be a promising strategy to treat highly malignant tumors.
According to several recent experimental studies concerned with the intraarterial administration of 3-BrPA in liver cancer, a single session of 3-BrPA injection showed no significant antitumor effect compared with that of saline injection (23) and Vali et al. (24) determined the optimal therapeutic dose of 3-BrPA. However, to the best of our knowledge, the therapeutic efficacy of 3-BrPA in comparison with doxorubicin, which is a currently established agent in TACE, has not yet been evaluated.
The purpose of this study was to evaluate the antitumor effects and hepatotoxicity of intraarterial delivery of lowdose 3-BrPA and high-dose 3-BrPA and to compare them with the antitumor effects and hepatotoxicity of TACE with using an established chemotherapeutic agent doxorubicin hydrochloride and iodized oil in a rabbit VX2 hepatoma model.
The 3-BrPA (Sigma Chemical Co., St. Louis, MO) solutions were prepared in phosphate buffer solution (PBS) and then they were adjusted to pH 7.4 with sodium hydroxide (NAOH). The solutions were sterilized with using a 0.2 µm filter unit (Sartorius' Minisart, Cat. No.16534). 3-BrPA solutions with 1 mM and 5 mM concentrations were prepared by mixing the 3-BrPA with a sterile PBS just before intraarterial infusion, and the solutions were used immediately. We used a freshly made 3-BrPA solution in all the experiments because of chemical instability.
This experiment was approved by the animal care committee of our institution. A total of 36 adult New Zealand white rabbits weighing 2.5-3.0 kg each were used for this experiment. The VX2 carcinoma strain had been maintained by successive transplantations into the hind limb of a carrier rabbit.
Anesthesia was introduced through an intramuscular injection of 50 mg of ketamine hydrochloride (Ketalar®; Yuhan Yanghang, Seoul, Korea) and 20 mg of xylazine hydrochloride (Rompun®; Bayer Korea, Seoul, Korea) at 0.5 mL per kilogram of body weight. After making a midline subxyphoid abdominal incision, 0.1 mL of minced VX2 carcinoma was directly implanted into the subcapsular parenchyma of the left medial lobe of the liver. All the procedures were performed aseptically. The tumors were allowed to grow for 2-3 weeks to attain a size of 15-30 mm in diameter.
One day prior to the intraarterial injection of the agents, a liver computed tomography (CT) scan was performed (MX 8000; Philips Medical Systems, Best, The Netherlands) with the animals in the supine position. Nonenhanced CT was performed to localize the liver. For contrast material-enhanced CT, 13 mL of contrast material (iopromide, Ultravist; Schering, Berlin, Germany) was injected at a rate of 0.5 mL/sec through the auricular vein. With using the bolus tracking technique, a hepatic arterial phase scan and a portal venous phase scan were obtained at approximately 7-8 seconds and 24-25 seconds respectively, after the injection of the contrast media. The scanning parameters we used were 3-mm collimation, 1.5 pitch and a 1-mm reconstruction interval.
On the CT scan, the location and size of the tumor were measured by two authors working in consensus. The volume (V) of the tumor was calculated according to the equation V = L × S2/2, where L is the longest diameter and S is the shortest diameter of the tumor (25, 26). The animals with similar-size tumors were distributed evenly to each group on the basis of the calculated tumor volume because the results of antitumor treatment can be influenced by the tumor volume (27, 28). Thirty-six rabbits were divided into four groups according to the intraarterially administered agents as follows: low dose 3-BrPA (25 mL in a 1 mM concentration, n = 10), high dose 3-BrPA (25 mL in a 5 mM concentration, n = 10), a Lipiodol (Andre Guerbet, Aulnay-sous-Bois, France)-doxorubicin (Adriamycin; Il Dong Pharmaceutical Co, Seoul, Korea) emulsion (1.6 mg doxorubicin/0.4 mL Lipiodol, n = 10) and the control group (25 mL normal saline, n = 6). The volumes of tumors were 3,977.9 mm3 ± 2,268.6 [mean ± standard deviation [SD]) with the low dose 3-BrPA (n = 10), 2,988.9 mm3 ± 2,921.1 with high dose 3-BrPA (n = 10), 4,360.3 mm3 ± 3,658.7 with Lipiodol-doxorubicin emulsion (n = 10) and 2,648.1 mm3 ± 2,374.6 in the control group (n = 6). The tumor volumes were not statistically different among the four groups (Kruskal-Wallis test, p = 0.3869).
The rabbits were sacrificed for histologic investigation with a lethal dose (90 mg/kg) of intravenously administered sodium pentobarbital (Pentothal; Choong Wae Pharmacy, Seoul, Korea) at seven days after the intraarterial procedure.
Transcatheter intraarterial administration was performed under fluoroscopic guidance by two authors who worked as a team. The same anesthesia protocol as carcinoma implantation was used, and the right femoral artery was exposed. A 5-Fr diameter dilator, as a substitute for an arterial sheath, was inserted into the right femoral vein. With using a 2.4-Fr microcatheter (Progreat; Terumo, Tokyo, Japan), celiac angiography was performed to identify the hepatic arterial anatomy and the feeder artery of the tumor. The left hepatic artery, which exclusively supplied blood flow to the tumor, was selectively catheterized. After the catheter was advanced to an adequate position in the left hepatic artery, the prepared 3-BrPA solution or normal saline was then infused for 2 minutes. The Lipiodol-doxorubicin emulsion was carefully injected to avoid efflux of the embolic materials out of the artery. The catheter was then removed and the femoral artery was ligated.
The antitumor effect was evaluated according to the tumor necrosis rates that were assessed by microscopic methods. The rabbits were sacrificed at seven days after transcatheter intraarterial administration and the livers of the rabbits were explanted and fixed in 10% buffered formaldehyde solution. The part of the liver that contained the tumor was sliced at 5 mm-intervals in the axial plane that corresponded to the plane of the CT scan, and the sections were completely embedded in paraffin for histologic examination, and after which the 4 µm-thick sections were stained with hematoxylin and eosin. The histologic changes and the extent of necrosis in the tumors were evaluated by an experienced board-certified pathologist. To quantify the antitumor effects, the proportion of the necrotic area relative to the entire tumor area was estimated with using Image-Pro Plus software (Media Cybernetics, Silver Spring, MD). The overall percentage of viable tumor was calculated based on the average of the percentage of viable tumor in each slice.
To determine the hepatic toxicities caused by the treatment, peripheral blood was drawn from the auricular vein in all the animals before and at 1, 2 and 7 days after intraarterial administration. The plasma aspartate aminotransferase (AST) level and the plasma alanine aminotransferase (ALT) level were measured with using a biochemical autoanalyzer (Hitachi Koki, Tokyo, Japan).
The SPSS software package (version 11.0; SPSS, Chicago, IL) was used for statistical data analysis. The Mann-Whitney U test and the Kruskal-Wallis test were applied to assess the statistical significance of the tumor necrosis rates and the plasma biochemical levels among the experimental groups. Statistical significance was set at p values < 0.05.
Transcatheter intraarterial administration was successfully performed in all animals, and none of the animals died during the experimental period. The tumor necrosis rates of the low dose 3-BrPA group (1 mM concentration, n = 10), the high dose 3-BrPA group (5 mM concentration, n = 10), the Lipiodol-doxorubicin emulsion group (0.4 mL Lipiodol /1.6 mg doxorubicin, n = 10) and the control group (25 mL normal saline, n = 6) are shown in Figure 1.
Compared with the tumor necrosis rates of the control group (48% ± 21.7), those of the low dose 3-BrPA group (62% ± 20.0) did not show a statistically significant difference (p = 0.2780) (Fig. 2). But the high dose 3-BrPA group (93% ± 7.6) (Fig. 3) and the Lipiodol-doxorubicin emulsion group (99% ± 2.7) showed higher tumor necrosis rates than that of the control group with statistical significance (p = 0.0002 and p = 0.0002, respectively). The complete eradication of the tumor was achieved only in the animals in the Lipiodol-doxorubicin emulsion group (n = 5). The tumor necrosis rate of the Lipiodol-doxorubicin emulsion group was significantly higher than that of the high dose 3-BrPA group (p = 0.0015). Therefore, the antitumor effect in the conventional TACE with Lipiodol-doxorubicin emulsion group is superior to that of the high dose 3-BrPA group.
Figure 4 shows the plasma (AST) level and the plasma level (ALT) before and at 1, 2 and 7 days after intraarterial administration. For both AST and ALT, the enzyme levels were elevated at the first day and then they decreased to levels close to normal at seven days after intraarterial administration. The levels of AST and ALT in the high dose 3-BrPA group and the Lipiodol-doxorubicin emulsion group were significantly elevated compared to those levels of the control group at one day (Kruskal-Wallis test, p < 0.05), whereas those levels of the low dose 3-BrPA group did not show a statistically significant difference compared with those levels of the control group (p > 0.05). There was also no significant difference between both the AST and ALT enzyme levels of the high dose 3-BrPA group and those levels of the Lipiodol-doxorubicin emulsion group (p > 0.05).
Although rabbit VX2 carcinoma did not originate from hepatocytes, it has been widely used as an experimental model for HCC because of its exclusive hepatic arterial supply and rapid growth pattern. Moreover, according to the reports about the biochemical characteristics of VX2 tumor, its glucose metabolic characteristics are similar to those of human HCC, with a high glycolytic/high hexokinase phenotype (8, 9, 20). These findings suggest that the rabbit VX2 hepatoma model can be used for evaluating the therapeutic effect of hexokinase inhibitor, which has the possibility to be an antitumor agent for HCC.
Hepatic arterial administration of 3-BrPA is a more efficient drug delivery method for treating hepatic tumors by providing regional delivery of 3-BrPA while avoiding the concomitant systemic toxicity. Furthermore, the normal liver parenchyma receives a dual blood supply from the hepatic artery and the portal vein, and the hepatic tumors are almost exclusively supplied by the hepatic artery. Therefore, hepatic arterial injection of 3-BrPA can attack hepatic tumors at a higher concentration and the damage to the normal liver tissue is minimized.
Geschwind et al. (22) reported that treatment with a single intraarterial injection of 3-BrPA (25 mL in a 0.5 mM concentration) achieved an 84% average tumor necrosis rate in the VX2 hepatoma model, as compared with a 26% average tumor necrosis rate in the nontreated control group. However, in our study, we failed to demonstrate significant anti-tumor effects after an intraarterial injection of low-dose 3-BrPA (25 mL in a 1 mM concentration), when compared with the injection of normal saline (61% versus 48%, respectively). The discrepancy between the studies may be explained in different ways. 3-BrPA is chemically unstable and its cellular uptake and biodistribution after intraarterial injection have not been clarified. 3-BrPA may undergo rapid neutralization if it encounters blood flow. After intraarterial injection of 3-BrPA, the concentration of 3-BrPA reaching the tumor vessels can be decreased due to dilution, to a variable degree, according to the amount of pericatheter flow. Another explanation is the possible ischemic necrosis of VX2 tumors in our control group during normal saline injection. This result is in accord with that of a previous study by Shin et al, in which the mean tumor necrosis rate in the saline group reached up to 68% (23). The authors inferred that the catheter that was nearly wedged in the small lumen of the hepatic artery induced a temporary occlusion of the hepatic artery and therefore this caused ischemic damage to the tumor (23).
In our study, we could demonstrate significant antitumor effects after intraarterial injection of high-dose 3-BrPA (25 mL in a 5 mM concentration). However, the achieved tumor necrosis rate was significantly lower than that of the established Lipiodol-doxorubicin emulsion (93% versus 99%, respectively). The limited therapeutic efficacy of a single intraarterial injection of 3-BrPA requires further development of a formulation that's suitable for intraarterial administration, and effort are needed to optimize the injection protocols for the injection duration and concentration, the total injected amount and the number of sessions and the interval between sessions. Of course, the mechanisms of cellular uptake, biodistribution and actions of 3-BrPA should be clarified with further investigations. As mechanisms of cell death, Gwak et al. (29) have shown that 3-BrPA induced apoptosis of HCC cell lines through activating the mitochondrial apoptotic signaling cascades. HCC cells have been shown to exhibit very high concentrations of hexokinase II (more than 100 fold of normal), and especially in the presence of hypoxia (20, 30). Therefore, the intraarterial administration of 3-BrPA after inducing tumor hypoxia, such as with balloon occlusion of the hepatic artery, may increase the therapeutic efficacy to the level seen in in vitro experiments (29).
Preserving the residual liver function is one of most important prognostic factors for liver cancer (31). It must be clarified whether 3-BrPA can preserve the normal liver cell function without significant cytotoxicity even with administering effective tumoricidal doses. Theoretically, normal hepatocytes can survive under hexokinase II inhibition because their energy metabolism does not depend on a hexokinase II expression. However, our results for the hepatocellular toxicity of 3-BrPA are not consistent with the previous in vivo study (22). Geschwind et al. (22) said that 3-BrPA showed minimal cytotoxicity on normal liver cells and the near tissues at a low concentration of 0.5 mM because normal hepatocytes do not express high glycolytic activity with glucokinase, and there is not much hexokinase II in their cytoplasm (19, 20). It has been reported that normal liver cells, the portal veins, the sinusoids and the bile ducts remained completely intact, with the only apparent damage occasionally occurring in the peribiliary arteriolar complexes, at much higher concentrations of 5 mM, 3-BrPA (22). In our study, significant elevations of liver enzymes were noted in the high dose 3-BrPA group, whereas there was no significant difference of liver enzymes between the low dose 3-BrPA group and the control group (p > 0.05). These results imply that 3-BrPA shows dose-related hepatotoxicity with its intraarterial administration. Although the hepatic toxicity induced by intraarterial administration of 3-BrPA did not exceed that of the Lipiodol-doxorubicin group and the liver enzymes were nearly normalized within one week in this in vivo study, higher doses of this agent should be studied to determine the maximum tolerated dose and the potential hepatotoxic effect.
This study has several limitations to be mentioned. First, an investigation concerned with the survival of tumorbearing animals was not done. A recent study has shown that intraarterial infusion of 3-BrPA in VX2 liver tumors proved to be beneficial due to the significant survival benefit without any treatment-related toxicity (32). Further investigations seem to be needed to verify the drug's dose-related survival differences. Second, even though the tumors with similar size were evenly distributed to each group to exclude any bias, the standard deviation of the tumor size in each group was relatively large. However, as a practical matter, the tumor growth rate is hardly under control and a further study with a larger population would minimize the size variability.
In conclusion, intraarterial delivery of 3-BrPA showed a dose-related antitumor effect and a single session treatment seems to have limited efficacy when compared with the conventional TACE. Further studies for determining the optimal treatment protocol to enhance the therapeutic effect and to minimize the hepatotoxicity are warranted.
References
1. Bosch FX, Ribes J, Borràs J. Epidemiology of primary liver cancer. Semin Liver Dis. 1999; 19:271–285. PMID: 10518307.


3. Bismuth H, Houssin D, Ornowski J, Meriggi F. Liver resections in cirrhotic patients: a Western experience. World J Surg. 1986; 10:311–317. PMID: 3010585.


4. Bruix J, Cirera I, Calvet X, Fuster J, Bru C, Ayuso C, et al. Surgical resection and survival in Western patients with hepatocellular carcinoma. J Hepatol. 1992; 15:350–355. PMID: 1332998.


5. Stuart KE, Anand AJ, Jenkins RL. Hepatocellular carcinoma in the United States. Prognostic features, treatment outcome, and survival. Cancer. 1996; 77:2217–2222. PMID: 8635087.


7. Weinhouse S. Glycolysis, respiration, and anomalous gene expression in experimental hepatomas: G.H.A. Clowes memorial lecture. Cancer Res. 1972; 32:2007–2016. PMID: 4343003.
8. Pedersen PL. Tumor mitochondria and the bioenergetics of cancer cells. Prog Exp Tumor Res. 1978; 22:190–274. PMID: 149996.


9. Bustamante E, Morris HP, Pedersen PL. Energy metabolism of tumor cells. Requirement for a form of hexokinase with a propensity for mitochondrial binding. J Biol Chem. 1981; 256:8699–8704. PMID: 7263678.


10. Dang CV, Semenza GL. Oncogenic alterations of metabolism. Trends Biochem Sci. 1999; 24:68–72. PMID: 10098401.


11. Rempel A, Mathupala SP, Griffin CA, Hawkins AL, Pedersen PL. Glucose catabolism in cancer cells: amplification of the gene encoding type II hexokinase. Cancer Res. 1996; 56:2468–2471. PMID: 8653677.
12. Smith TA. Mammalian hexokinases and their abnormal expression in cancer. Br J Biomed Sci. 2000; 57:170–178. PMID: 10912295.
13. Mathupala SP, Rempel A, Pedersen PL. Glucose catabolism in cancer cells. Isolation, sequence, and activity of the promoter for type II hexokinase. J Biol Chem. 1995; 270:16918–16925. PMID: 7622509.
14. Johansson T, Berrez JM, Nelson BD. Evidence that transcription of the hexokinase gene is increased in a rapidly growing rat hepatoma. Biochem Biophys Res Commun. 1985; 133:608–613. PMID: 4084289.


15. Rempel A, Bannasch P, Mayer D. Differences in expression and intracellular distribution of hexokinase isoenzymes in rat liver cells of different transformation stages. Biochim Biophys Acta. 1994; 1219:660–668. PMID: 7948023.


16. Shinohara Y, Ichihara J, Terada H. Remarkably enhanced expression of the type II hexokinase in rat hepatoma cell line AH130. FEBS Lett. 1991; 291:55–57. PMID: 1936251.


17. Viitanen PV, Geiger PJ, Erickson-Viitanen S, Bessman SP. Evidence for functional hexokinase compartmentation in rat skeletal muscle mitochondria. J Biol Chem. 1984; 259:9679–9686. PMID: 6746664.


18. Pedersen PL. Mitochondrial events in the life and death of animal cells: a brief overview. J Bioenerg Biomembr. 1999; 31:291–304. PMID: 10665520.
19. Ko YH, Smith BL, Wang Y, Pomper MG, Rini DA, Torbenson MS, et al. Advanced cancers: eradication in all cases using 3-bromopyruvate therapy to deplete ATP. Biochem Biophys Res Commun. 2004; 324:269–275. PMID: 15465013.


20. Ko YH, Pedersen PL, Geschwind JF. Glucose catabolism in the rabbit VX2 tumor model for liver cancer: characterization and targeting hexokinase. Cancer Lett. 2001; 173:83–91. PMID: 11578813.


21. Ko YH, McFadden BA. Alkylation of isocitrate lyase from Escherichia coli by 3-bromopyruvate. Arch Biochem Biophys. 1990; 278:373–380. PMID: 2183722.


22. Geschwind JF, Ko YH, Torbenson MS, Magee C, Pedersen PL. Novel therapy for liver cancer: direct intraarterial injection of a potent inhibitor of ATP production. Cancer Res. 2002; 62:3909–3913. PMID: 12124317.
23. Shin SW, Han H, Choo SW, Yoo BC, Park CK, Do YS, et al. Hepatic intra-arterial injection of 3-bromopyruvate in rabbit VX2 tumor. Acta Radiol. 2006; 47:1036–1041. PMID: 17135005.


24. Vali M, Liapi E, Kowalski J, Hong K, Khwaja A, Torbenson MS, et al. Intraarterial therapy with a new potent inhibitor of tumor metabolism (3-bromopyruvate): identification of therapeutic dose and method of injection in an animal model of liver cancer. J Vasc Interv Radiol. 2007; 18:95–101. PMID: 17296709.


25. Watanabe D, Ueo H, Inoue H, Matsuoka H, Honda M, Shinomiya Y, et al. Antitumor effects of intraarterial infusion of tumor necrosis factor/lipiodol emulsion on hepatic tumor in rabbits. Oncology. 1995; 52:76–81. PMID: 7800348.


26. Okada M, Kudo S, Miyazaki O, Saino T, Ekimoto H, Iguchi H, et al. Antitumoral efficacy and pharmacokinetic properties of pirarubicin upon hepatic intra-arterial injection in the rabbit VX2 tumour model. Br J Cancer. 1995; 71:518–524. PMID: 7880733.
27. Park HS, Chung JW, Jae HJ, Kim YI, Son KR, Lee MJ, et al. FDG-PET for evaluating the antitumor effect of intraarterial 3-bromopyruvate administration in a rabbit VX2 liver tumor model. Korean J Radiol. 2007; 8:216–224. PMID: 17554189.


28. Yoon CJ, Chung JW, Park JH, Yoon YH, Lee JW, Jeong SY, et al. Transcatheter arterial chemoembolization with paclitaxel-lipiodol solution in rabbit VX2 liver tumor. Radiology. 2003; 229:126–131. PMID: 12944599.


29. Gwak GY, Yoon JH, Kim KM, Lee HS, Chung JW, Gores GJ. Hypoxia stimulates proliferation of human hepatoma cells through the induction of hexokinase II expression. J Hepatol. 2005; 42:358–364. PMID: 15710218.


30. Pedersen PL, Mathupala S, Rempel A, Geschwind JF, Ko YH. Mitochondrial bound type II hexokinase: a key player in the growth and survival of many cancers and an ideal prospect for therapeutic intervention. Biochim Biophys Acta. 2002; 1555:14–20. PMID: 12206885.


31. Llovet JM, Burroughs A, Bruix J. Hepatocellular carcinoma. Lancet. 2003; 362:1907–1917. PMID: 14667750.


32. Vali M, Vossen JA, Buijs M, Engles JM, Liapi E, Ventura VP, et al. Targeting of VX2 rabbit liver tumor by selective delivery of 3-bromopyruvate: a biodistribution and survival study. J Pharmacol Exp Ther. 2008; 327:32–37. PMID: 18591216.


Fig. 1
Tumor necrosis rates (%) of experimental groups (expressed as mean tumor necrosis rate ± SD). Tumor necrosis rate was significantly higher in high dose group (93% ± 7.6) than that in control group (48% ± 21.7) (p = 0.0002), but tumor necrosis rate was not significantly higher in low dose group (62% ± 20.0) (p = 0.2780). However, tumor necrosis rate of high dose group was significantly lower than that of Lipiodol-doxorubicin treatment group (99% ± 2.7) (p = 0.0015).
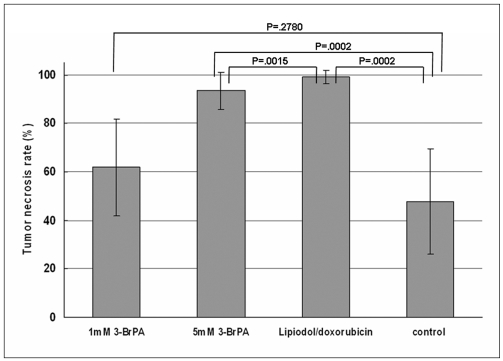
Fig. 2
Representative case of 3-BrPA 1 mM group.
A. Contrast-enhanced CT image obtained one day before intraarterial administration shows low attenuated VX2 carcinoma in left hepatic lobe of rabbit (arrow).
B. On selective left hepatic angiography that was done just before transcatheter intraarterial administration, hypervascular tumor staining is seen in left hepatic lobe (arrows). After microcatheter was advanced to left hepatic artery, low dose 3-BrPA solution (25 mL in 1 mM concentration) was infused for 2 minutes.
C. Photograph of resected specimen of tumor. Pathologic specimens containing tumor were sectioned in same transverse plane that was used for CT scan with 5-mm intervals, and slice with largest tumor area was embedded in paraffin for histologic examination.
D. Photomicroscopic slide of same specimen (Hematoxylin & Eosin staining, ×1). Necrosis rate of tumor is 81%.
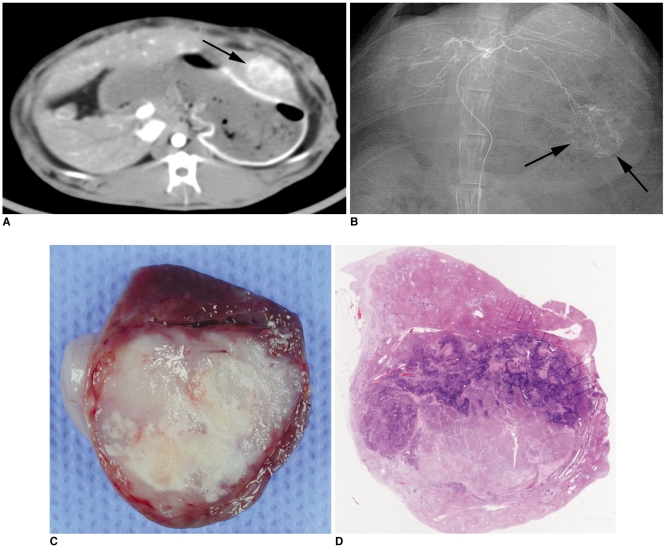
Fig. 3
Representative case of 3-BrPA 5 mM group.
A. Contrast-enhanced CT image obtained one day before intraarterial administration shows low attenuated mass with enhancing rim in left hepatic lobe (arrow).
B. On selective hepatic arteriography, hypervascular tumor staining is seen in left hepatic lobe (arrows).
C. Photograph of resected specimen of tumor.
D. Photomicroscopic slide of same specimen (Hematoxylin & Eosin staining, ×1). Necrosis rate of tumor is 99%.
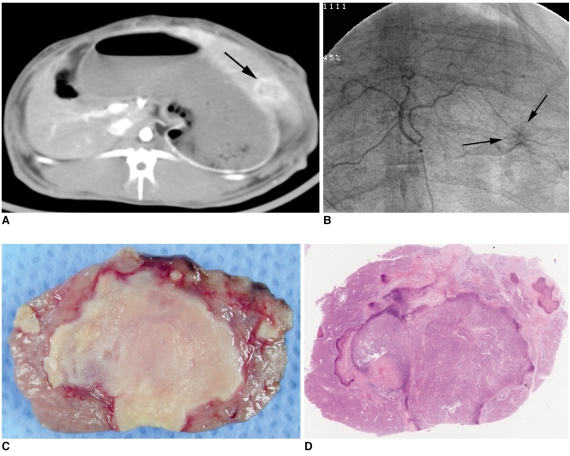
Fig. 4
Biochemical analysis of liver enzymes; mean values of liver enzymes in each group. Line graph shows that aspartate aminotransferase (AST) and alanine aminotransferase (ALT) levels tended to transiently increase at one day after intraarterial administration and they decreased to levels close to normal at seven days. Levels of AST and ALT of high dose 3-BrPA group and Lipiodol-doxorubicin emulsion group were significantly elevated as compared to those levels of control group at one day, whereas those levels of low dose 3-BrPA group did not show statistically significant difference as compared with those levels of control group. There was also no significant difference between both AST and ALT enzyme levels of high dose 3-BrPA group and those of Lipiodol-doxorubicin emulsion group.
A. AST levels.
B. ALT levels.
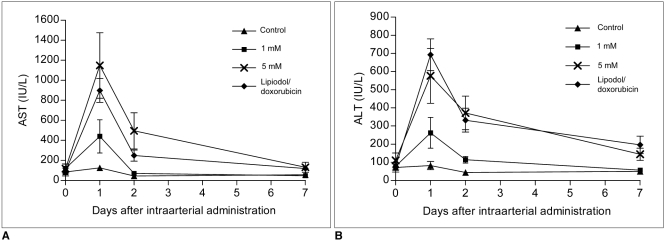