Abstract
Objective
To assess effects of off-centering, automatic exposure control, and padding on attenuation values, noise, and radiation dose when using in-plane bismuth-based shields for CT scanning.
Materials and Methods
A 30 cm anthropomorphic chest phantom was scanned on a 64-multidetector CT, with the center of the phantom aligned to the gantry isocenter. Scanning was repeated after placing a bismuth breast shield on the anterior surface with no gap and with 1, 2, and 6 cm of padding between the shield and the phantom surface. The "shielded" phantom was also scanned with combined modulation and off-centering of the phantom at 2 cm, 4 cm and 6 cm below the gantry isocenter. CT numbers, noise, and surface radiation dose were measured. The data were analyzed using an analysis of variance.
Results
The in-plane shield was not associated with any significant increment for the surface dose or CT dose index volume, which was achieved by comparing the radiation dose measured by combined modulation technique to the fixed mAs (p > 0.05). Irrespective of the gap or the surface CT numbers, surface noise increased to a larger extent compared to Hounsfield unit (HU) (0-6 cm, 26-55%) and noise (0-6 cm, 30-40%) in the center. With off-centering, in-plane shielding devices are associated with less dose savings, although dose reduction was still higher than in the absence of shielding (0 cm off-center, 90% dose reduction; 2 cm, 61%) (p < 0.0001). Streak artifacts were noted at 0 cm and 1 cm gaps but not at 2 cm and 6 cm gaps of shielding to the surface distances.
Several techniques have been introduced to appropriately manage CT radiation dose such as adapting scan parameters to patient size and using automatic exposure control techniques (1). As dose reduction techniques affect attributes of image quality, before applying these techniques, their effect on image quality needs to be assessed so that lesions or abnormalities are not missed or become non-interpretable. In addition to these user-controlled techniques for dose optimization, modern multidetector CT scanners also have other hardware to reduce radiation dose. For instance, pre-patient X-ray filters which prevent "soft," low energy X-rays from reaching the patient and contributing to radiation dose without taking part in actual image formation. On the other hand, bowtie filters shape the X-ray beam so that thinner peripheral parts of the patient's cross section receive a lower radiation dose compared to the thicker central parts (2).
Recently, the United States Food and Drug Administration (FDA) has approved the bismuth based in-plane shields for CT scan radiation protection. The current literature suggests that these shields can also reduce CT radiation dose, particularly to the superficial radiosensitive organs such as the breasts, eye-lens, thyroid gland, and gonads (3-7). Insufficient evidence exists about radiation dose and image quality effects as a result of shielding with different padding (distance between the shield and patient surface) and off-centering (extent of mis-centering of patients relative to the gantry iso-center) distances. The purpose of our study was to assess the effect of off-centering, automatic exposure control, and padding on CT numbers, noise, and radiation dose when using in-plane bismuth based shields.
Authors do not have any financial relationships associated with this study. As no animal data, human data, or studies were acquired or used, the present study was exempt from Institutional Review Board approval.
We used a 30 cm × 20 cm × 10 cm (transverse diameter × anterior posterior diameter × length) anthropomorphic chest phantom (QRM-Thorax, QRM GmBH, Mohrendorf, Germany) comprising of artificial lung lobes, shell of soft tissue equivalent material, tissue equivalent solid core cardiac insert (35 Hounsfield unit [HU] ± 5 HU), and a spine insert. The base material for the phantom was a resin weighing approximately 3,620 grams. The use of this phantom for assessing CT image quality has been described in prior studies (8).
A commercially available bismuth based in-plane breast shield (Breast Shield Medium, F & L Medical Products, Vandergrift, PA) was used for this study. This shield consists of a 1 mm thick bismuth (1.7 gram bismuth/cm2 which is equivalent to 0.45 mg/cm3 of lead), which was impregnated with a synthetic rubber covering and mounts firmly on a 0.635 cm foam base offset. As per the vendor (personal communication, F & L Medical Products), the foam offset was added to decrease the streak artifacts noted in the body region adjacent to the contact surface with the shield. As specified in the product brochure, these breast shields are disposable.
A 64-channel multidetector row CT scanner (Somatom Sensation 64 Cardiac, Siemens Medical Solutions, Forchheim, Germany) was used for scanning the phantom, with and without the shield. The scanner was calibrated just prior to the study and the entire scanning was performed in the same scanning session.
We used a calibrated, solid-state metal oxide semiconductor field effect transistor (MOSFET dosimeter, EDD-30; Unfors Instruments, Billdal, Sweden) dosimeter to measure radiation dose in the study. The MOSFET dosimeters have been validated in prior studies for CT dose measurements and are sensitive to very small doses of radiation with a linear dose response (3, 9).
We evaluated the effect of three predictive variables including distance between the shield and the surface of the phantom, extent of off-centering the phantom, and automatic exposure control technique on three outcome variables. In turn the three outcome variables include the CT numbers (Hounsfield units [HU]), quantitative image noise (standard deviation of the CT numbers), and the surface radiation dose as measured by the MOSFET dosimeter.
Prior to scanning, four MOSFET dosimeters were deployed at a distance of 5 cm from each other on the anterior surface of the phantom. The dosimeters were taped to the phantom to avoid dislodging and to maintain their constant position throughout the entire study. The absorbed doses at the surface were measured for each scan series, with the dosimeters set to baseline zero prior to acquisition of each scan series. The reason for measuring surface dose and not absorbed organ dose was attributed to the fact that bismuth shields are primarily intended to reduce surface dose.
First, the phantom was centered in the gantry isocenter and scanned without the shield using a routine non-contrast chest CT protocol. The helical scan parameters comprised of 120 kVp, fixed effective mAs of 100 (effective mAs is defined as ratio of tube current time product to pitch), 0.9: 1 pitch, 5 mm slice reconstructed section thickness, 5 mm reconstructed section interval, 64 × 0.6 detector configuration, 0.5 second gantry rotation time, and a B30f reconstruction filter (corresponding to a generic soft tissue reconstruction algorithm).
After the baseline scanning, the shield was placed directly on the anterior surface of the phantom with no gap or foam pad provided by the vendor for offset (offset distance = 0 cm). Next, we increased the offset distance between the shield and the phantom by inserting foam pads of different thicknesses (1, 2, and 6 cm) between the shield and phantom surface. Thus, the shielded phantom was scanned four times at each of the offset distances using identical scan parameters. Although the vendor recommends only a 1 cm offset between the shield and the patient's surface, we used different offset distances in this study to assess their effect on image quality and surface dose reduction. Direct contact (offset distance = 0 cm) was selected to assess the effect of direct placement of the shield over the phantom surface, whereas a 1 cm offset distance was selected to approximate the vendor recommended shield to surface distance. The remaining two offset distances were arbitrarily selected to assess the effect of a minor offset increase (2 cm) over the vendor recommended offset and to determine what effect a much higher offset (6 cm) would have on image quality and radiation dose. The phantom was centered in the gantry isocenter for these four scan series. Repeat scanning was performed over the identical position and length of the phantom.
Next, after scanning at an offset distance between the shield and the anterior surface of the phantom of 1 cm, we rescanned the phantom three more times, with the center of the phantom at 2, 4, and 6 cm below the gantry isocenter. These off-centering distances have also been used in a prior study to assess effect of off-centering on CT image quality and radiation dose without the use of shields (2). The scan parameters for each acquisition was identical to the one used with the phantom centered at the gantry isocenter, as detailed above.
Finally, the phantom was scanned twice using the combined modulation technique (CARE Dose 4D, Siemens Medical Solutions) with a reference effective mAs of 100, first without the shield and then with the shield at 1 cm offset distance from the anterior phantom surface. The modulation strengths of CARE Dose 4D, which adjust the dose based on the size of the patient (weaker dose for slim patients and stronger dose for obese patients), were identical to those used for routine clinical CT examinations performed with CARE Dose 4D. The remaining scan parameters were held identical. The combined modulation technique and CARE Dose 4D performs automatic modulation of the tube current along both the z-axis based on patients with cross sectional attenuation information from localizer radiograph, and along the x-y axes based on data collected during the initial half rotation around the area of interest being scanned (readers are referred to prior publications for technical details of CARE Dose 4D) (10).
A 1 cm offset distance between the shield and the phantom surface was employed for off-centering. Furthermore, the automatic exposure control portion of this study used an identical offset to a prior publication (11).
The images were transferred onto an image post processing workstation (Leonardo, Siemens Medical Solutions, Forchhiem, Germany) for analysis. The CT numbers and quantitative image noise were recorded in each scan series at identical slice locations and positions with the uniform regions of interests (ROIs) in the anterior aspect (portion of the phantom underlying the shield, 25 mm2 oval ROI), center (50 mm2 circular ROI) and posterior aspect (away from shield, 20 mm2 oval ROI) (Fig. 1).
In addition, one radiologist (Cardiac Imaging subspecialty with 2 years of experience) graded the streak artifacts in each series acquired with and without the shield. The streak artifacts were graded as 0 (no streak artifacts), 1 (mild streaking in the immediate vicinity of the phantom), and 2 (severe streaking on any region of the phantom impairing ability to see the tissue interfaces). Moreover, these artifacts were assessed at soft tissue windows only (window width 350 H, window level 60 H).
To assess the effect of shielding on the CT numbers in the different parts of the images, we obtained surface plots of the DICOM images (Fig. 1) using a public domain freeware image processing software, ImageJ (http://rsb.info.nih.gov/ij/index.html, National Institute of Health, USA). For the surface plots of the CT numbers, DICOM images were opened in the ImageJ program and the "Surface plot" option was selected from the "Analyze" menu.
The data were entered into Microsoft Excel (Microsoft Inc., Redmond, WA) for analysis.
The mean and standard deviations were calculated for the outcome variables (CT numbers, quantitative image noise and radiation doses). In addition, the different predictive factors (distance between shield and phantom surface, off-centering, and combined modulation) were estimated along with the percent change between the outcome and predictive variables. These analyses were performed on Microsoft Excel worksheets.
We performed an analysis of variance (ANOVA) to determine the statistical difference between the different outcome variables at different shield to phantom surface distances and off-centering positions. In addition, an R x C contingency Chi-square test was performed to compare the effects of combined modulation techniques on radiation dose with and without the shield. The ANOVA and Chi-square tests were performed using the SAS software (SAS Inc., Cary, NC). To correct for multiple testing (n = 5), Bonferroni's correction was performed to redefine the probability value for significant statistical difference (p < 0.01).
The effect of shield offset distance on radiation dose is summarized (Table 1). Regardless of the shield to surface distance, radiation dose was significantly lower with shields than without shields (36.9 - 40.5%; p < 0.0031). However, there was no significant change in radiation dose with increasing offset distance between the shield and the anterior surface of the phantom (p = 0.2).
A substantial increase in CT numbers and image noise in the images occurred when scanning was performed with the shield compared to without the shield (p < 0.0001). The increase in CT numbers and image noise became progressively less pronounced with increasing shield to surface distance (Tables 2, 3). Moreover, the maximum increase in CT number and image noise was noted in images with direct contact between the shield and phantom surface. Streak artifacts were only noted at the anterior aspect of the phantom with no offset or a 1 cm offset, with the former being associated with more pronounced streaking (score = 2) compared to the latter (score = 1).
The surface plots of the CT numbers showed a substantial increase in the anterior aspect of the phantom with no offset of the shield, which decreased with increasing shield to surface offset (Fig. 2).
The effect of off-centering on CT numbers, quantitative image noise, and surface radiation dose for the shielded phantom is summarized (Fig. 3). As expected, the quantitative image noise increased with increasing off-centering distance, but there was no change in the CT numbers with changes in the off-centering distance. Moreover, no significant trend or statistical difference was noted in either of these three outcome variables (p > 0.08). The severity of streak artifacts did not change with increase in off-centering distance (score = 1 at all off-centering distances).
Regardless of the off-centering distance, both the CT numbers and image noise were greater and the radiation dose was lower for phantoms with shield compared to scanning without the shield (p < 0.0001).
The effects of a combined modulation technique on the CT numbers, quantitative image noise, and surface radiation dose are summarized (Fig. 4). No significant difference in the CT numbers was noted between the images obtained from scanning the shielded phantom at constant tube current and the combined modulation technique (p = 0.2). However, image noise was considerably higher for images acquired with the combined modulation technique compared to the constant tube current scanning (p < 0.0001). Likewise, surface radiation dose was also significantly less for the combined modulation technique compared to the constant tube current technique (p < 0.0001).
Lead based shielding radiation protection devices have been in use for decades in conventional radiography and fluoroscopy. These devices provide radiation protection against scattered radiation and have also been assessed for radiation protection from scattered radiation or out-of-plane radiation associated with CT scanning (3-7, 11, 12). However, modern day multidetector CT scanners have high radiation efficiency and little scattered radiation beyond the scanning plane. As a result, in-plane bismuth shields were introduced in the 1990s to reduce the CT radiation dose to radiosensitive body parts (5, 6).
Several studies have described the benefits of in-plane bismuth shields in CT scanning (3-7, 11, 12). Substantially in both adult and children, CT radiation dose studies have documented benefits of these shields by showing substantial dose reduction by reducing direct incident X-rays to important radiosensitive parts of the body including breasts, the thyroid gland, and eyes (3-7, 11, 12). There are conflicting reports on the effect of shields on image quality (image noise and streaking), with at least one prior study reporting a potential limitation of the bismuth based in-plane shields for CT radiation dose reduction as a result of greater image noise and streak artifacts (11, 13). However, to the best of our knowledge, no prior study has investigated how different offset distances, off-centering distances, and automatic exposure control affect image quality and radiation dose when these shields are used in CT imagery.
Our study is consistent with prior studies which found that in-plane shields do substantially decrease radiation dose. Furthermore, we confirmed that the shields reduce surface radiation dose even when there is greater offset from the surface (up to 6 cm) and off-centering. Likewise, with the combined modulation technique used in our study, shielding results in additional radiation dose savings. However, our study raises a number of concerns about the use of shields in CT imagery. We noted a substantial increase in the CT numbers for both the shielded surface and the center of the phantom (at about a 10-15 cm depth from the shielded surface). Although this effect was less pronounced at a 6 cm offset distance, the CT numbers at the anterior surface and the central portion of the phantom were still about 66% and 26% greater with shield than without the shield, respectively.
A differential increase in CT numbers, particularly at the shielded surface of the phantom, is most likely due to increased attenuation of the incident X-ray beam at the shielded surface compared to the opposite non-shielded aspect of the phantom. On the contrary, less pronounced but definite increase in the central CT numbers may be explained by the greater contribution of X-ray beams from the non-shielded aspect of the phantom compared to that from the shielded aspect. No increase in the CT numbers was found with off-centering or automatic exposure control.
The increase in image noise at the different offset distances noted in our study was likely related to increased beam hardening and scattering associated with the shield. The combined modulation technique further decreased the surface radiation dose, which led to an increase in image noise compared to the constant tube current. Our results are not consistent with Fricke et al. who reported no increase in image noise with and without shielding (11). This might be due to the fact that this prior study assessed shields in children who are typically scanned at a low radiation dose and have a higher image noise, whereas we assessed an adult anthropomorphic phantom at a standard adult CT protocol, which involves a higher radiation dose (11). On the other hand, our observation of increased image noise with shielding is consistent with Geleijns et al. (13), who also recommended against the use of bismuth shields.
Our study has important clinical implications. We caution radiologists against the use of in-plane bismuth shields for routine CT studies primarily because they can adversely affect the CT numbers and image noise in the vicinity of shielded surface as well as in more important central parts of the image. In particular, shields should be avoided when a CT is being performed for the evaluation of abnormalities located in the vicinity of the surface over which the shield will be placed. In such instances, radiation dose should be reduced by other means such as reducing tube current. Otherwise, care should be taken to increase the distance between the shield and the surface. Direct contact between the shield and patient's surface should be avoided under all circumstances. The shield to surface offset distance should be maximized by using a vendor supplied foam rest (typically less than 1 cm in thickness) and adding further cushion between the shield and the surface with foam or rolled sheets.
It is also important for radiologists to exercise caution when using absolute CT numbers in images acquired in shielded regions. For this reason, it is also important to avoid the use of shields when the absolute CT numbers need to be used for clinical interpretation such as for coronary calcium scoring, renal cyst, or adrenal mass characterization. For the two latter situations, technologists must be instructed to always ensure use of a proper sized breast shield and to avoid misplacement of shield over the abdomen.
When using the shields, radiologists must take into account the possibility of interaction with the automatic exposure control technique. Our study does prove that the automatic exposure control technique (CARE Dose 4D) does exclude the high attenuation bismuth shield from confounding the estimation of the appropriate tube current and reduces the radiation dose even further compared to constant tube current scanning. However, the drawback could be an inadvertent deterioration in image quality at such lower radiation doses due to greater image noise. Thus, appropriate adjustments must be made to any low dose CT techniques when using shields.
Although shields do impose limitations on regional image quality, we believe that they should still be employed in several clinical situations as demonstrated in prior studies as well as the current study (3-8, 11, 13). Foremost, shields must be used in addition to other radiation dose reduction techniques such as automatic exposure control when a low dose CT is unlikely to compromise the required information such as for a pulmonary nodule follow up, evaluation of a bony thorax, and other follow up chest CT studies. Another situation where shields may be beneficial would be for shielding breasts in abdominal CT studies as well as shielding the thyroid gland for chest CT studies. For other routine chest CT images, the use of shields may be avoided in favor of other dose reduction techniques such as the use of a low tube current, kilovoltage, or automatic exposure control. For the aforementioned reasons, our study does not provide any evidence for or against the use of shields for pediatric CT radiation dose reduction. However, according to the vendor of the shields assessed in the present study, these shields are disposable, which can add to the cost of CT exams.
Few variable were considered in our study. For example, the effect of shields on image quality in clinical CT examinations was not evaluated. Moreover, the greatest limitation of this study was not evaluating the effect of shields in patients undergoing a CT scan. This was primarily due to the fact that we decided against the use of the shields for clinical studies based on the results of our phantom studies. Also, we chose a single ply in-plane bismuth shield thickness for our study and did not assess other thicknesses or other shields (i.e., tungsten antimony shield). Based on our results, it is reasonable to assume that given the greater lead equivalency of the shields not assessed in our study would have had a greater effect on CT numbers, noise, and radiation dose. Moreover, the effect of shield offset distance greater than 6 cm was not assessed. As in most patients, it will not be possible to interpose such large and potentially heavier foam or offset device between their body and the shields.
Another concern with the study design is that we did not assess the effect of shields on CT scanners from different vendors. It is however, unlikely that the effect would have been different given the fundamental mechanism of CT image generation and X-ray beam attenuation by the shield. Likewise, other automatic exposure controls from different vendors were not assessed. Although not seen in our study, it is conceivable that greater X-ray beam attenuation by the shielded phantom could have resulted in a higher tube current and radiation dose with automatic exposure control techniques from other vendors.
In conclusion, in-plane shields are associated with a substantial increase in the CT numbers and image noise, irrespective of an offset distance of up to 6 cm. Although shields reduce the radiation dose even with the use of an automatic exposure control technique, users must be cautious about artifactual increases in CT numbers and image noise. Lastly, shields also reduce CT radiation dose regardless of the extent of off-centering.
Figures and Tables
Fig. 1
Transverse CT image and surface plot of phantom without shield. Three regions of interest were drawn on CT images at same level and position in anterior (A), central (C) and posterior (P) regions of phantom. As expected, vertebra has highest CT numbers on surface plot image.
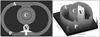
Fig. 2
Original transverse CT images of phantom (*) acquired at 0 cm (Ao), 1 cm (Bo), 2 cm (Co), and 6 cm (Do) offsets (black arrows) and their corresponding CT numbers' contour maps (A, B, C, D). Compared to image acquired with 6 cm offset (D), images with 0-2 cm offsets have considerably higher CT numbers and lighter shade of gray on surface plots (A, B, C). Also note difference in CT numbers in anterior and posterior aspects of phantom.
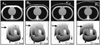
Fig. 3
Off-centering of shielded phantom below gantry isocenter did not cause any substantial change in CT numbers (HU ant/center in anterior or central regions of the phantom), noise, and average surface radiation dose compared to scanning with appropriate phantom centering.
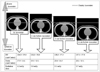
Fig. 4
Combined modulation technique (AEC) and plane shielding.
A. Compared to images acquired without shield, CT numbers in anterior (HU ant) and central (HU center) regions of phantom are substantially higher with shield, with and without combined modulation, whereas CT numbers are similar in posterior region (HU post) of phantom.
B. Compared to images acquired without shield, image noise in anterior (SD ant) and central (SD center) regions of phantom are substantially higher with shield, with and without combined modulation, whereas image noise is similar in posterior region (SD post) of phantom. In particular, combined modulation scanning was associated with greater image noise compared to fixed tube current scanning when phantom was scanned with shield for both techniques.
C. Comparison of images acquired with or without shield, with both conditions having constant fixed tube current, combined modulation technique resulted in significantly lower individual (doses 1, 2, 3, 4) and average surface radiation doses (AVG DOSE).
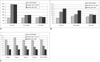
Table 1
Effect of Shield Offset Distance on Radiation Dose (mGy) Noted at Four (doses 1, 2, 3 and 4) Points on Anterior Surface of Phantom
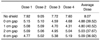
Table 2
Effect of Shield Offset Distance on CT Numbers Noted in Anterior (HU anterior), Central (HU Center) and Posterior (HU posterior) Regions of Phantom
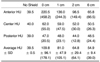
References
1. Kalra MK, Maher MM, Toth TL, Hamberg LM, Blake MA, Shepard JA, et al. Strategies for CT radiation dose optimization. Radiology. 2004. 230:619–628.
2. Li J, Udayasankar UK, Toth TL, Seamans J, Small WC, Kalra MK. Automatic patient centering for MDCT: effect on radiation dose. AJR Am J Roentgenol. 2007. 188:547–552.
3. Mukundan S Jr, Wang PI, Frush DP, Yoshizumi T, Marcus J, Kloeblen E, et al. MOSFET dosimetry for radiation dose assessment of bismuth shielding of the eye in children. AJR Am J Roentgenol. 2007. 188:1648–1650.
4. Colombo P, Pedroli G, Nicoloso M, Re S, Valvassori L, Vanzulli A. Evaluation of the efficacy of a bismuth shield during CT examinations. Radiol Med (Torino). 2004. 108:560–568.
5. Hopper KD, Neuman JD, King SH, Kunselman AR. Radioprotection to the eye during CT scanning. AJNR Am J Neuroradiol. 2001. 22:1194–1198.
6. Hopper KD. Orbital, thyroid, and breast superficial radiation shielding for patients undergoing diagnostic CT. Semin Ultrasound CT MR. 2002. 23:423–427.
7. Dauer LT, Casciotta KA, Erdi YE, Rothenberg LN. Radiation dose reduction at a price: the effectiveness of a male gonadal shield during helical CT scans. BMC Med Imaging. 2007. 7:5.
8. Primak AN, McCollough CH, Bruesewitz MR, Zhang J, Fletcher JG. Relationship between noise, dose, and pitch in cardiac multi-detector row CT. Radiographics. 2006. 26:1785–1794.
9. Yoshizumi TT, Goodman PC, Frush DP, Nguyen G, Toncheva G, Sarder M, et al. Validation of metal oxide semiconductor field effect transistor technology for organ dose assessment during CT: comparison with thermoluminescent dosimetry. AJR Am J Roentgenol. 2007. 188:1332–1336.
10. Rizzo S, Kalra M, Schmidt B, Dalal T, Suess C, Flohr T, et al. Comparison of angular and combined automatic tube current modulation techniques with constant tube current CT of the abdomen and pelvis. AJR Am J Roentgenol. 2006. 186:673–679.
11. Fricke BL, Donnelly LF, Frush DP, Yoshizumi T, Varchena V, Poe SA, et al. In-plane bismuth breast shields for pediatric CT: effects on radiation dose and image quality using experimental and clinical data. AJR Am J Roentgenol. 2003. 180:407–411.
12. McLaughlin DJ, Mooney RB. Dose reduction to radiosensitive tissues in CT. Do commercially available shields meet the users' needs? Clin Radiol. 2004. 59:446–450.
13. Geleijns J, Salvadó Artells M, Veldkamp WJ, López Tortosa M, Calzado Cantera A. Quantitative assessment of selective in-plane shielding of tissues in computed tomography through evaluation of absorbed dose and image quality. Eur Radiol. 2006. 16:2334–2340.