Abstract
Objective
In order to investigate the functional brain anatomy associated with verbal and visual working memory, functional magnetic resonance imaging was performed.
Materials and Methods
In ten normal right handed subjects, functional MR images were obtained using a 1.5-T MR scanner and the EPI BOLD technique. An item recognition task was used for stimulation, and during the activation period of the verbal working memory task, consonant letters were used. During the activation period of the visual working memory task, symbols or diagrams were employed instead of letters. For the post-processing of images, the SPM program was used, with the threshold of significance set at p < .001. We assessed activated brain areas during the two stimulation tasks and compared the activated regions between the two tasks.
Results
The prefrontal cortex and secondary visual cortex were activated bilaterally by both verbal and visual working memory tasks, and the patterns of activated signals were similar in both tasks. The superior parietal cortex was also activated by both tasks, with lateralization to the left in the verbal task, and bilaterally without lateralization in the visual task. The inferior frontal cortex, inferior parietal cortex and temporal gyrus were activated exclusively by the verbal working memory task, predominantly in the left hemisphere.
Conclusion
The prefrontal cortex is activated by two stimulation tasks, and this is related to the function of the central executive. The language areas activated by the verbal working memory task may be a function of the phonological loop. Bilateral prefrontal and superior parietal cortices activated by the visual working memory task may be related to the visual maintenance of objects, representing visual working memory.
Positron emission tomography (PET) and functional MR (fMR) are noninvasive tools for functional mapping of the brain. At present, fMR is widely used for the investigation of neuroanatomical basis and for clinical presurgical mapping. fMR and PET are powerful tools for exploring cognitive function, especially language and memory, as well as the somatosensory function of the brain. Working memory is a term referring to a brain system that provides temporary storage and active manipulation of the information necessary for such complex cognitive tasks as language comprehension, learning, and reasoning (1-4). Working memory is known to have three subsystems: the central executive, the visuospatial sketch pad, and the phonological loop. The central executive is an attentional-controlling system, and two subsystems, the visuospatial sketch pad and the phonological loop, play a role in the manipulation of visuospatial and verbal informaiton, respectively (1, 2). Although PET and fMR studies of working memory have shown that functional areas of the brain activated by working memory tasks are different according to the stimulation task (5-8), the functional neuroanatomy of a working memory task is still under investigation.
The purpose of this study is to investigate the functional brain anatomy associated with visual and verbal working memory, using fMR.
Ten healthy subjects (male, 5; female, 5; average age, 24 ± 4 years) were included in this study. All were university students with no history of neurological or psychiatric disorder, and gave informed written consent. All were right-handed as determined by the Edinburgh inventory (9). The subjects received detailed instructions regarding the experimental conditions and had a brief practice session before entering the scanner.
Working memory tasks consisted of verbal and visual stimuli. During the activation period of the verbal working memory task, randomized sequences of five phonologically dissimilar consonants of the Korean language were displayed on the screen every second. In order to detect whether a target constant appeared 1 second after a series of five consonants was presented, subjects were instructed to rehearse the stimuli silently and to remember them serially. During the activation period of visual working memory task, serial diagrams or symbols that could not be phonologically transcoded were presented instead of consonants. Subjects were asked to remember each stimulus using a visual code. The visual working memory task was otherwise identical to the verbal task. For both types of tasks, each sequence of stimuli was repeated five times during the activation period. For the identification of each sequence of stimuli, one blank slide was presented for 1 second after one sequence of stimuli during an activation task period. Subjects responded by pressing a squeeze ball. Under control conditions of visual or verbal working memory task, the subjects were instructed to visually fixate on a crosshair.
MR images were generated on a 1.5-T MR (General Electric Medical Systems, Milwaukee, WI). A series of echoplanar gradient echo images was acquired at intervals of 3 seconds (TR/TE, 3000/60 msec; flip angle, 90°; matrix, 64×64; slice thickness, 5mm; no slice gap; FOV, 24×24 cm). Twenty brain slices were obtained parallel to the plane of the anterior commissure (AC) and posterior commissure (PC) line. Before the acquisition of functional images, T1-weighted images (TR/TE, 417/9 msec; matrix, 256×256; slice thickness, 5 mm; no slice gap; FOV, 24×24 cm) were obtained as anatomic reference images. During the acquisition of functional images, there were two periods of task performance and three resting periods (off-on-off-on-off). Each period of activation or rest was 39 sec and 13 series of functional images were acquired during each period. Dummy scans were obtained during an initial 12-second period of echo-planar imaging, and during a verbal or visual task the total time for acquisition of the whole functional image was 207 sec.
Each subject's echoplanar functional images were corrected for possible head movements using automated image reconstruction (AIR) software (10). Functional image analysis was performed using statistical parametric mapping (SPM) software 96 (SPM96, MRC Cyclotron Unit, London, U.K.). To smooth each image a Gaussian filter was applied, and to suppress the low frequency physiologic noise in the functional images, a high-pass filter was used. Functional maps were created overlapping of functional echoplanar images with anatomic T1-weighted images, and the activated signals were thresholded at p < .001 (Z score > 3.1). Brodmann areas (BAs) of activated signals in the two working memory tasks were visually determined by means of a standard stereotactic space (11). For the activated regions, a comparison was also made between verbal and visual working memory tasks.
In all subjects, errors in both verbal and visual working memory tasks were less than 20%. Aactivated regions of the brain are summarized in Table 1.
During the two working memory tasks, activated signals were seen in the frontal, parietal, temporal, and occipital lobes, and the insula, cingulate gyri and cerebellum. Activated regions of the frontal lobe were the supplementary motor area (BA 6), motor and premotor (BA 4, 6), prefrontal cortex (BA 9, 10, 46), and inferior frontal gyri (BA 44, 45, 47). Activated regions of the parietal lobe were the superior parietal lobe (BA 7) along the intraparietal sulci, the supramarginal gyrus (BA 40), and the angular gyrus (BA 39) (Fig. 1).
When the visual working memory task was compared with the verbal task, common regions activated by the two tasks were the supplementary motor area, the motor and premotor area, the prefrontal cortex, the superior parietal gyri, and the posterior fusiform gyri. Although these common regions were activated by both working memory tasks, there was a difference in the distribution of activated signals between each hemisphere. In the verbal working memory task, bilaterally activated signals that were similar between each hemisphere were seen in the prefrontal cortices (8 of 10 subjects), fusiform gyri (7 of 8 subjects), and occipital cortices (all 10 subjects). Activated signals in the supplementary motor area (9 of 10 subjects), premotor area (all subjects), and superior parietal cortices (7 of 10 subjects) were lateralized to the left hemisphere during the verbal working memory task. In the visual task, signals activated in the prefrontal cortex and occipital lobe were similar in both hemispheres. In the visual task, signals activated in the fusiform gyri were stronger in the right hemisphere in 3 of 8 subjects and were similar between each hemisphere in other subjects. Although bilaterally activated cerebellar signals were found in both visual and verbal working memory tasks, those activated by the visual task were less strong than those activated by the verbal task.
In activated regions which included the inferior frontal gyri, inferior parietal cortex, temporal lobe and insula, there was a difference between the verbal and visual working memory task. In the former, these activated regions were, in most subjects, lateralized to the left hemisphere. In the visual task, however, signals in these regions were found in only a few subjects and they were not distinctly lateralized. During the verbal working memory task, activated signals of the inferior frontal gyri were seen in all ten subjects and these were lateralized to the left hemisphere in eight. Meanwhile, during the visual task, activated signals were seen in the inferior frontal gyri in only two subjects. In the inferior parietal gyri, activated signals were noted in nine subjects during the verbal working memory task, but in only two during the visual task. In this same area, activated signals were seen mainly in the left supramarginal gyri (BA 40) during the verbal working memory task. In the temporal lobe, activated signals were seen in the middle temporal gyri in all subjects during the verbal working memory task, but in only three during the visual task. In nine subjects, signals were also activated in the insula during the verbal working memory task, but in only one during the visual task.
A widely recognized distinction within memory is a dichotomy based upon temporal duration, namely short-term and long-term. According to the accessibility of the retrieved elements to consciousness, long-term memory can be subdivided into declarative, explicit memory, and procedural, implicit memory (12). Short-term memory is a system that provides temporary storage and active manipulation of limited information. At present, the concept of working memory has increasingly replaced the concept of short-term memory. After Atkinson and Shiffrin (13) proposed the modal model that postulated a unique short-term store, the concept of working memory was suggested by Baddely and Hitch (1). This is a temporally and spatially limited capacity that includes multicomponents of short-term storage systems and is capable of simultaneously storing and manipulating the information used in complex cognitive functions (2). Working memory also invloves the cognitive function of continuously updating and actively maintaining information, as well as the temporary storage of digits or words. Working memory is, therefore, a system necessarily required for complex cognitive tasks (3), and is known to have three subsystems: the central executive, the visuospatial sketch pad and the phonological loop. The visuospatial sketch pad manipulates visuospatial information, and the phonological loop stores and rehearses verbal informations. The central executive control and integrates the other two subsystems (1-3).
In the present study, the paradigm of working memory task was modified from the item-recognition task described by Paulesu (14, 15). The experimental design of this study was based on a subtraction format in which both visual and verbal working memory tasks were designed as activation tasks, and visual fixation as a control task for the avoidance of subtraction of common activated signals. In this study, the verbal stimuli of Korean consonants activated the function of verbal working memory of the phonological store and subvocal rehearsal of verbal information, and the visual stimuli of symbols or diagrams activated the function of maintenance of visual images. The central executive function may be activated by both visual and verbal working memory tasks.
The results of this study showed that common regions activated by both verbal and visual stimuli were the prefrontal cortices (BA 9, 10, 46) and secondary visual cortices (BA 18, 19), in which the activated signals were similar between each hemisphere during both verbal and visual working memory tasks. These common regions are consistent with the brain regions activated during previous PET and fMR studies (16-20). Previous studies (16-20) also showed that the prefrontal cortex was activated by a task that stimulates the updating or active maintenance of verbal or visual information. The lateral occipital cortex is an area of visual pathway that is activated by various visual stimuli (21, 22). In this study, bilateral activation of lateral occipital cortices seems to have been caused by the perceptual processing of visual stimuli of the verbal or visual working memory task. According to a study of visual working memory (19), occipitotemporal areas in the ventral object vision pathway showed mostly transient responses to visual stimuli, thus indicating their predominant role in the perceptual process, whereas prefrontal areas demonstrated sustained activity, indicating their predominant role in working memory. The prefrontal cortices activated by the visual and verbal working memory tasks may, therefore, play a predominant role in the executive control of working memory.
In our study of verbal working memory, signals which activated in the superior parietal cortex were dominant in the left hemisphere. This left dominance seems to related to the neural network of the phonological loop of verbal working memory (20). However, signals which activated in the superior parietal cortex during the visual task were similar between each hemisphere. Activation of the bilateral superior cortex during the visual working memory task may be explained by the findings of a PET study (12) showing that bilateral superior parietal cortices are activated by a visual working memory task, whereas simple visual stimuli activate only bilateral occipital cortices. Activation of the right parietal cortex may also be related to the neural network of sustained attention involved in the function of working memory (23). In the present study, the cerebellum was activated by both tasks and activated cerebellar signals were stronger in the verbal working memory task than in the visual task. Activation of the cerebellum is known to be related to motor function, and its activation may be related to the internal articulation involved in the verbal working memory task (20). The mechanism by which the cerebellum is activated during the visual working memory task is not clear, but it may be that in some subjects, a diagram or symbol is partly phonologically transcoded.
Bilateral activation of the posterior fusiform gyri might be due to the existence of a ventral visual pathway that is involved in the perceptual processing of visual objects and the visual recognition of verbal stimuli (22, 24).
The activation of premotor and motor areas during the verbal working memory task may be related to the motor function of the phonological loop, and the activation of these areas during the visual task might be related to the use of phonological loop or secondary activation originating in the prefrontal cortex during the visual task (20).
Activated areas which were distinct between verbal and visual working memory tasks were the inferior frontal gyrus, inferior parietal cortex, superior and middle temporal gyri, and the insula. In most subjects, these areas were strongly activated by verbal working memory task, whereas during the visual task, weak activated signals were seen in these same areas in just a few subjects. This result shows that these areas have a predominant role in the phonological loop of the working memory task. According to a previous PET study (14), the inferior frontal gyri (Broca's area) may play a role in subvocal rehearsal, and the supramarginal gyrus may play a role in phonological store.
In summary, fMR was used to investigate the functional brain anatomy associated with visual and verbal working memory. Based on converging results in this and previous studies, prefrontal cortices activated by both visual and verbal stimuli appear to play a role in the central executive function of active maintenance of information. The left inferior frontal gyri and inferior parietal cortex were dominantly activated by verbal task, forming part of the phonological loop of verbal working memory. The bilateral prefrontal and superior parietal cortices, activated by visual stimuli, seem to be related to the neural pathway involved in the visual working memory task. Our study demonstrates that functional areas of working memory could be identified by fMR and that different regions of the brain involved in visual and verbal working memory.
Figures and Tables
Fig. 1
Functional MR imaging of a healthy 26-year-old male obtained during verbal (A-C) and visual working memory tasks (D-F).
A-C. Activation map images obtained during verbal working memory task.
Map image of the upper level of the brain (A) demonstrates bilateral activated signals in the premotor area (BA 6)(long arrows), supramarginal gyrus (BA 40)(short arrows), and superior parietal cortex (BA 7)(arrow heads). The activated signals are stronger in the left premotor and superior parietal cortex than in the right hemisphere. An activated signal is also seen in the right prefrontal cortex (BA 9)(curved arrow) and anterior cingulate gyrus (BA 24). Map image of the middle level of the brain (B) shows activated signals in the left inferior frontal lobe (BA 44, 45)(long arrow), left superior temporal gyrus (BA 22)(short arrow), middle temporal gyrus (BA 21)(thick short arrows), and prefrontal cortex(BA 10)(curved arrow). Map image of the lower level of the brain (C) shows activated signals in the right and left lateral occipital cortices(BA 18,19) and posterior fusiform gyrus (BA 37)(arrows). Small activated signals are also seen in the left insula and middle temporal gyrus.
D-F. Activation map images obtained during visual working memory task. Map image of the upper level of the brain (D) shows neither activated signals in the supramarginal gyrus nor lateralization of activated signals in the frontal and parietal lobes. Map image of the middle level of the brain (E) shows no activated signals in the left inferior frontal or temporal gyrus. An activated signal (arrow) in the prefrontal cortex corresponds to the signal activated during the verbal working memory task (D, E). Map image of the lower level of the brain(F) shows bilateral activated signals similar to those seen during the verbal working memory task in the right and left occipital cortices and posterior fusiform gyri.
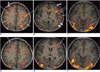
Table 1
Summary of Brain Areas Activated during Working Memory Tasks in Ten Normal Volunteers
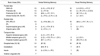
Note.-BA = Brodmann's area, SMA = supplementary motor area, SPL-IPS = superior parietal lobe and intraparietal sulcus, IPL = inferior parietal lobe. *= lateralization of activated signals, L or R = unilateral activated signals in the right or left hemisphere; L > R or R > L = bilateral signals mainly in either the right or left hemisphere; B = bilateral signals activated to a similar extent in both hemispheres.
References
1. Baddely AD, Hitch G. Bower GA, editor. Working memory. The psychology of learning and motivation. 1974. New York: Academic Press;47–89.
2. Baddely AD. Working memory. Science. 1992. 255:556–559.
3. Baddely AD. Gazzaniga MS, editor. Working memory. The cognitive neurosciences. 1996. London: MIT press;755–764.
4. Baddely AD. Recent developments in working memory. Curr Opin Neurobiol. 1998. 8:234–238.
5. Paulesu E. The neural correlates of the verbal component of working memory. Nature. 1993. 362:342–345.
6. Smith EE, Jonides J, Koeppe RA, Awh E, Schumacher EH, Minoshima S. Spatial versus object working memory: PET investigations. J Cogn Neurosci. 1995. 7:337–356.
7. Jonides J, Smith DD, Marshuetz C, Koeppe RA. Components of verbal working memory: evidence from neuroimaging. Proc Natl Acad Sci USA. 1998. 95:876–882.
8. Ungerleider LG, Courtney SM, Haxby JV. A neural system for human visual working memory. Proc Natl Acad Sci USA. 1998. 95:883–890.
9. Oldfield RC. The assessment and analysis of handedness: the Edinburgh inventory. Neuropsychologia. 1971. 9:97–113.
10. Woods RP, Cherry SR, Mazziota JC. A rapid automated algorithm for accurately aligning and reslicing PET images. J Comput Assist Tomogr. 1992. 16:620–633.
11. Talairach J, Tournoux P. Co-planar stereotaxic atlas of the human brain. 1988. New York: Thieme.
12. Frackowiak RSJ, Friston KJ, Frith CD, Dolan Raymond, Mazziotta JC. Human brain function. 1997. Toronto: Academic Press;367–404.
13. Atkinson RC, Shiffrin RM. Specne KW, editor. Human memory: a proposed system and its control processes. The psychology of learning and motivation: advances in research and theory. 1968. New York: Academic Press;89–195.
14. Paulesu E, Frith CD, Frackowiak RSJ. The neural correlates of the verbal component of working memory. Nature. 1993. 362:342–345.
15. Paulesu E, Connelly A, Frith CD, et al. Functional MR imaging correlation with positron emission tomography. Neuroimag Clin N Am. 1995. 5:207–225.
16. Salmon E, Linden VL, Delfiore G, et al. Regional brain activity during working memory task. Brain. 1996. 119:1617–1625.
17. Kammer T, Bellemann ME, Gukel F, et al. Functional MR imaging of the prefrontal cortex: specific activation in a working memory task. Magn Reson Imaging. 1997. 15:879–889.
18. Cohen JD, Peristein WM, Braver TS, et al. Temporal dynamics of brain activation during a working memroy task. Nature. 1997. 386:604–608.
19. Courtney SM, Ungerleider LG, Keil K, Haxby JV. Transient and sustained activity in a distributed neural system for human working memory. Nature. 1997. 386:608–610.
20. Fiez JA, Raife EA, Balota DA, et al. A positron emission tomography study of the short-term maintenance of verbal information. J Neurosci. 1996. 16:808–822.
21. Haxby JV, Grady CL, Horowitz B, et al. Dissociation of object and spatial visual processing pathways in the human extrastriate cortex. Proc Natl Acad Sci USA. 1991. 88:1621–1625.
22. Ungerleider LG. Functional brain imaging studies of cortical mechanisms for memory. Science. 1995. 270:769–775.
23. Coull JT, Frith CD, Frackowiak RSJ, Grasby PM. A fronto-parietal network for rapid visual information processing: a PET study of sustained attention and working memory. Neuropsychologia. 1996. 34:1085–1089.
24. Buchel C, Price C, Frith CD. A multimodal language region in the ventral visual pathway. Nature. 1998. 394:274–277.