Abstract
This study evaluated the anti-asthmatic activities of 2,6-di-tert-butyl-4-hydroxymethylphenol (DBHP) that is a potent phenolic antioxidant in edible vegetable oil. The effects of DBHP on bronchial asthma were evaluated by determining the specific airway resistance (sRaw) and tidal volume (TV) during the immediate asthmatic response (IAR) and the late-phase asthmatic response (LAR) in guinea pigs with aerosolized ovalbumin-induced asthma. Recruitment of leukocytes and the levels of biochemical inflammatory mediators were determined in the bronchoalveolar lavage fluids (BALFs), and histopathological surveys performed in lung tissues. DBHP significantly inhibited the increased sRaw and improved the decreased TV on IAR and LAR, and also inhibited recruitment of eosinophils and neutrophils into the lung, and release of biochemical inflammatory mediators such as histamine and phospholipase A2 from these infiltrated leukocytes, and improved pathological changes. However, anti-asthmatic activities of DBHP at oral doses of 12.5 to 50 mg/kg was less than those of dexamethasone (5 mg/kg, p.o.) and cromoglycate (10 mg/kg, p.o.), but more potent or similar to that of salbutamol (5 mg/kg, p.o.). These results in the present study suggest that anti-asthmatic effects of DBHP in the guinea pigs model of OVA-induced asthmatic responses principally are mediated by inhibiting the recruitments of the leukocytes and the release of biochemical inflammatory mediators from these infiltrated leukocytes.
Allergens activate inflammatory cells in the airways, leading to the release of pro-inflammatory mediators, which in turn cause vascular leakage, contraction of bronchial smooth muscle, infiltration of inflammatory cells, and airway inflammation and remodeling [12]. Allergen provocation induces an immediate asthmatic response (IAR) that peaks at 15 to 30 min and resolves within 2 h, and a late-phase asthmatic response (LAR) that begins 4 to 12 h after allergen challenge [34].
Bronchial asthma is considered to be a chronic inflammatory disease of the airway. Thus, anti-inflammatory therapy is considered central for its long-term asthma management [5]. Corticosteroids inhalations are highly effective for chronic treatments, and are capable of suppressing the underlying inflammatory process. Furthermore, combination of inhalers containing corticosteroids and long-acting β2-selective adrenoceptor agonists are the principle approach to asthma therapy [6]. However, concern persists regarding the use of inhaled corticosteroids, because significant side effects are occasionally exhibited by long-term use, for example, osteoporosis and growth stunting in children. Although these effective medications are delivered by inhalation, compliance is surprisingly poor [7]. In addition, cromoglycate, a dichromone flavonoid derivative, has a similar chemical structure to flavonoids and inhibits the release of chemical mediators, and has been used as an anti-asthmatic drug, especially the prophylactic treatment of allergic asthma [8]. Prophylactic treatment with anti-allergic drugs such as cromoglycate and corticosteroids inhibits both IAR and LAR, whereas β2-selective adrenoceptor agonists such as salbutamol inhibits IAR but not LAR [910].
In approximately 60% of all asthmatic subjects, the IAR is followed by a LAR, and LAR is also accompanied by an increase in bronchial responsiveness to nonspecific stimuli and thought to lead to serious and chronic asthma. Therefore, agents that can inhibit the LAR may be of therapeutic benefit in the treatment of bronchial asthma [3]. A number of animal models have been developed in which LAR occurs in the airways after exposure to antigen, and many studies concerned about LAR suggest the contribution of many kinds of mediators, cytokines and inflammatory cells, especially eosinophils in the development of LAR in humans [3]. However, the pharmacological characteristic of LAR on the animal models is not documented satisfactorily, and the mechanisms underlying LAR are not clear. Moreover, despite the enormous efforts that have been put into developing anti-asthmatic agents, to date, there has been limited success in developing therapeutics for long-term treatment of the bronchial asthma without significant side effect. We previously reported that the plant-based flavonoids, quercetin and rutin which has significantly inhibited asthmatic responses, based on observed inhibition of chemical mediator release in bronchoalveolar lavage fluids (BALFs) [1112]. Furthermore, it was found that a methanolic extraction of Aralia cordata Thunb. (Araliaceae), which is used as a traditional herbal medicine for disorders such as inflammation, fever, and pain, has significant anti-asthmatic activity in guinea pigs with IgE-mediated asthma [13].
In the present study, we described that 1) a guinea pig model of bronchial asthma, and associated leukocyte infiltration and biochemical mediators releases in the airways as measured by BALF as well as histopathology of the asthmatic lung tissue, and 2) the effect of 2,6-di-tert-butyl-4-hydroxymethyl phenol (DBHP) (Fig. 1A), an antioxidant aromatic compounds used in foods and belongs to the family of cumenes, against IAR and LAR upon exposure of aerosolized ovalbumin (OVA) to conscious OVA-sensitized guinea pigs in the double-chambered plethysmograph (Fig. 1B).
2,6-di-tert-butyl-4-hydroxymethylphenol (molecular weight 236.35; ≥97%) (Cat# 446424, Aldrich), ovalbumin grade V from hen egg white (molecular weight 44,287; lyophilized powder, ≥98%; agarose gel electrophoresis) (Cat# A5503, Sigma) and other chemicals were purchased from Sigma-Aldrich Chemical Co. (St. Louis, MO, USA). All other chemicals purchased were of analytical grade.
Specific pathogen-free male Dunkin-Hartley guinea pigs were (250–300 g) were obtained from the HanLim animal house facility (Hwa Sung-Gun, Kyung Ki-Do, South Korea), and housed under standard laboratory conditions (temperature 24±2℃, humidity 50±5℃, illumination 300–500 Lux) with free access to pathogen-free food and water ad libitum. The animal studies were approved by the Institutional Animal Care and Use Committee of Chung-Ang University (IACUC-2015-00068).
Guinea Pigs were actively sensitized by i.p. and s.c. injections of 0.5 ml of 10% (w/v) OVA in saline on the same day. Twenty-one days after sensitization, these animals were selected on the basis of positive skin response to i.d. injection of 1% OVA (0.1 ml per site), and the sensitized animal was challenged with 10 ml inhalation of 1% OVA which was generated by compressed air with an aerosol nebulizer (Module PY2-73-1963, Hugo Sachs Elektronik, Germany) connected to the nasal chamber in a double-chambered plethysmograph (HSE type 855 and PLUGSYS 603, Hugo Sachs Elektronik, Germany) for 5 min after measuring baseline airway function. An operating air pressure was approximately 1.5 bar, and the generated particle size was below 10 µm, with a portion of particles having a diameter ≤2.5 µm of 60% [34]. Test drugs suspended in 5% carboxymethylcellulose solution were administered orally 1 h prior to OVA challenge, and again 12 h later.
To determine pulmonary and airway functions of conscious guinea pigs to the OVA challenge, tidal volume (TV), tidal airflow, respiratory rate, and specific airway resistance (sRaw) were measured using a barometric double-chambered plethysmograph (HSE type 855 and PLUGSYS 603, Hugo Sachs Elektronik, Germany) connected to volumetric differential pressure transducer (PT5, Grass Instrument Co., USA) and noninvasive respiratory analyzer (Model 7E Polygraph, Grass Instrument Co., USA) 5 min (for IAR) and 24 h (LAR) after the OVA [34]. The sRaw, the main index of airway responsiveness, was calculated by Pennock Program 89 and expressed in mmHg×sec [14].
After measurement of pulmonary function parameters at LAR, animals were anesthetized with a mixture of zolazepam and tiletamine (20 mg/kg, i.p.) and lungs were lavaged four times with 5 ml aliquots of Ca2+/Mg2+-free Hank's balanced salt solution (HBSS) containing EDTA (10 mM), HEPES (20 mM) and bovine serum albumin (1%). Bronchoalveolar lavage fluids (BALFs) were centrifuged (200×g for 10 min at 4℃), and the cell pellets were resuspended in 1 ml of HBSS for cellular analysis. Supernatants were stored at −80℃ for biochemical analysis. Total leukocytes in BALF were counted manually with hemocytometer, and differential cell counts were performed on cytocentrifuged preparations (Cytospin II, Shandon Southern Instruments, PA, USA) after modified Wright-Giemsa staining. A minimum of 300 cells were counted and classified as eosinophils, neutrophils, macrophages, or lymphocytes based on standard morphological criteria [4].
The released histamine content in BALF was determined by O-phthalaldehyde (OPA) spectrofluorometric method [15]. Briefly, 1 ml BALF was transferred to a test tube and 1 ml H2O, and 0.4 ml of 1N NaOH was added, followed 4 min later by 0.1 ml of OPA reagent and 0.2 ml of 3N HCl. The reaction mixture was transferred to a microplate and fluorescence measured at excitation 350 nm/emission 650 nm with a spectrophotometer (FL600 Microplate Fluorescence Reader, Bio-Tek, USA).
Phospholipase A2 activity in BALF was determined with a pyrene-labeled phosphatidylcholine (10-pyrene PC) in the presence of serum albumin with a spectrophotometer at excitation 345 nm/emission 398 nm [16]. Spectrofluorometric analysis of pyrene phospholipids and fatty acids was carried out in reaction mixture. The 10-pyrene PC was dried under nitrogen and suspended in ethanol at 0.2 mM. Reaction solution was prepared by sequential addition of 1 ml buffer containing 50 mM Tris-HCl (pH 7.5), 100 mM NaCl, 1 mM EDTA; 10 µl of substrate (2 µM, final concentration); 10 µl of substrate (to 0.1% final concentration) and 6 µl of 1 M CaCl2 (to 6 mM, final concentration). The fluorescence of the reaction medium (black) was recorded and the reaction was initiated by the addition of BALF. The specific activity in nanomoles per minute and per milligram protein was obtained by dividing the activity by the amount of protein in mg.
To determine the protein exudates quantitation in BALF was determined with bicinchoninic acid (BCA) colorimetric method [17]. Briefly, a 50 µl of aliquot of a 10-fold dilution of BALF was incubated with 1 ml of BCA reagent for 15 min at 37℃. After re-equilibration to room temperature, the reaction mixture was quantified spectrophotometrically at 595 nm. Because this is a kinetic assay, a set of standards must be run at the beginning and end as well as at appropriate intervals between the reaction mixtures.
For histologic analysis, lung tissues were fixed by infiltrating the lung with phosphate-buffered formalin saline, and dehydrated with a graded aqueous ethanol series and embedded in paraffin. The embedded lung tissues were sectioned (4 µm), and then stained with hematoxylin and eosin (H&E) to visualize inflammatory responses and pathological changes in the lung tissue.
Airway hyperreactivity following the aerosolized OVA inhalation was determined as aRaw. The sRaw of the OVA-sensitized guinea pigs was significantly increased by 297% (from 3.2±0.3 to 12.6±1.0 mmHg×sec, p<0.01) on IAR, and 147% (from 7.9±0.8 to 3.2±0.3 mmHg×sec, p<0.01) on LAR, respectively (Fig. 2). Furthermore, the mean baseline value of TV in vehicle control and OVA control were 2.8±0.1 and 3.5±0.2 ml, respectively. After challenge of ovalbumin, the TV of OVA control were decreased by 59% on IAR and 30% on LAR, respectively (Fig. 3). DBHP at an oral dose of 12.5 mg/kg significantly decreased the sRaw by 31.0% during IAR (p<0.05) and 38% during LAR (p<0.05) compared with OVA control, but also increased significantly the decreased TV by 19% during IAR (p<0.05). However, its inhibitory activities were less than that of anti-asthmatic drugs, dexamethasone (5 mg/kg, p.o.), cromoglycate (10 mg/kg, p.o.) and salbutamol (5 mg/kg, p.o.) (Figs. 2 and 3).
Antigen challenge of OVA-sensitized guinea pigs significantly increased the total leukocytes in BALF approximately five-fold, compared with vehicle control (7.4×105 versus 35.3×105 cells/ml, p<0.05) (Fig. 4A). Especially, eosinophils and neutrophils significantly increased compared with vehicle control (p50.05) (Figs. 4B and C). These data showed that antigen challenge increased eosinophils in BALF by sixteen-fold (0.5×105 to 7.7×105 cells), and also neutrophils by three-fold 2.2×105 to 7.9×105 cells) over the vehicle control. DBHP at the oral doses of 12.5 and 25 mg/kg significantly inhibited the recruitment of total leukocytes (35.3×105 to 28.3×105 cells/ml, p<0.05), eosinophils (7.7×105 to 7.0×105 cells, p<0.05) and neutrophils (7.9×105 to 6.9×105 cells, p<0.05) into the lung compared with OVA control, whereas salbutamol (5 mg/kg, p.o.) was not significantly.
Antigen challenged guinea pigs during LAR showed significant increases in the released levels of biochemical inflammatory mediators, histamine, protein and PLA2. Histamine contents in the BALF of vehicle- and OVA-challenged guinea pigs were 125±7 and 425±13 ng/ml during LAR, respectively, which was equivalent to an increase of 340% in OVA-challenged guinea pigs as compared with vehicle control (Fig. 5A). Moreover, protein contents, which was considered the component of exudates in BALF by airway inflammatory reaction of vehicle control and OVA control were 111±39 and 432±23 µg/ml on LAR, respectively. It was equivalent to an increase of 389% in OVA-challenged guinea pigs as compared with vehicle control (Fig. 5B). In addition, PLA2 activity in the BALF of vehicle control was 2.9±0.1 nmol/min/mg, increasing by 250% in the OVA control to 7.1±0.2 nmol/min/mg during LAR (Fig. 5A). It means PLA2 activity related to eicosanoid inflammatory mediators increased during an asthmatic response induced by aerosolized antigen in OVA-sensitized guinea pigs. DBHP inhibited the releases of biochemical inflammatory mediators and protein exudation. DBHP at an oral dose of 25 mg/kg significantly inhibited histamine release into BALF (425±13 to 346±15 ng/ml, p<0.05), but also significantly decreased protein exudates (432±23 to 307±28 ng/ml, p<0.05) and PLA2 activity (7.1±0.2 to 6.2±0.2 nmol/min/mg, p<0.05), respectively. However, its activities were less than those of dexamethasone (5 mg/kg, p.o.) and cromoglycate (10 mg/kg, p.o.).
The lungs of antigen challenged guinea pigs showed eosinophil and neutrophil recruitment in the alveolar sacs, peripheral vasculature and terminal bronchioles, compared with vehicle control (Figs. 6A and B), which is consistent with previous reports [1118]. Lung of vehicle control on LAR showed a normal structural architecture, and no inflammatory cell infiltration around terminal bronchioles (Fig. 6A). The oral administration of DBHP at a 50 mg/kg reduced the recruitment of leukocyte, particularly, eosinophils and neutrophils into alveolar sacs and peripheral vasculature on LAR (Fig. 6C), and dexamethasone (5 mg/kg, p.o.) also significantly improved pathological changes with mild leukocytes infiltration around bronchioles on LAR, compared with OVA-challenged control (Fig. 6D).
We have developed a guinea pig model of IAR and LAR through the inhalation of antigen OVA in OVA-sensitized guinea pigs. The guinea pig has generally been chosen as an experimental animal because of histological similarities that exist between lungs of antigen exposed guinea pigs and asthmatic lungs in human because this species can exhibit early and late-phase airway obstruction, bronchial eosinophilia and increased airway reactivity following exposure of sensitized animals to antigen in the putative involvement of chemical mediator, cytokines and inflammatory cells on the development of LAR, compared to other species such as mouse, rat, primate or rabbit [419]. Furthermore, the double-chambered plethysmograph system for restrained guinea pigs in this study has been specially developed for the investigation of bronchospasm-molytically active substances on a conscious animal, and it has been proven as a reliable and standard method in the study of pulmonary functions in a non-invasive approach. In spite of the higher sensitivity and specificity of invasive lung function tests in anesthetized animals, the non-invasive technique highly useful to access effects on breathing pattern and to detect pulmonary irritation and airflow limitation, and to test on adverse effects of chemicals and drugs. Moreover, this technique is simple to handle and the breathing pattern is nearly natural since no anesthesia is required [2021].
In animals sensitized with 10 µg of OVA, aerosolized OVA inhalation increased sRaw by 3-fold in IAR and 2-fold in LAR, respectively, compared with sRaw before OVA challenge. Furthermore, the well-established BAL technique in the present study and in subsequent histopathological study showed recruitment of leukocytes and eosinophils into the lung increased by 5-fold and 16-fold, respectively, also similar to a segmental challenge in human lung [22]. Of particular interest, bronchoconstriction can be associated with leukocyte infiltration, leading to the release of biochemical mediators such as histamine, leukotriene B4 (LTB4), and PLA2 [23]. In addition, eosinophils, neutrophils, lymphocytes, and macrophages are important cellular mediators of the allergic inflammation through the production of inflammatory cytokines [24].
In the present study, the oral administration of DBHP significantly inhibited the increased sRaw induced by antigen OVA challenge in IAR and LAR, but also improved the histopathologic impairments, with less effect than that of anti-asthmatic drugs, dexamethasone, cromoglycate and salbutamol. Furthermore, DBHP significantly decreased the levels of cellular and biochemical mediators induced by OVA challenge in BALF like other phenolic compounds, as in other previous reports [1825]. These results suggest that DBHP exerts significant anti-asthmatic activity during IAR and LAR in this in vivo model, probably due to the inhibition of histamine content in BALF. DBHP may act as mast cell stabilizers and bronchodilators through the downregulation of mast cell activation. Moreover, DBHP significantly decreased the OVA-induced PLA2 activity in BALF, which generates chemokines in eosinophil. In particular LTB4 could be derived from arachidonic acid through the PLA2 pathway at a cell membrane. LTB4 also mediates constriction of airway smooth muscle, leukocytes chemotaxis, and vascular permeability [2627]. In agreement with these rationales, we suggest that the increase of sRaw by antigen challenge is caused by the release of biochemical inflammatory mediators such as histamine and PLA2 metabolites such as leukotrienes, resulting in bronchi contraction, and DBHP has an anti-asthmatic effect on antigen-induced bronchoconstriction, which is mediated primarily by histamine and leukotrienes [282930]. Indeed, DBHP significantly inhibited the recruitment of total and subtypes of leukocytes, particularly eosinophils in BALF, which are also consistent with the histopathological survey. As well known, eosinophil accumulation into the inflamed tissue leads to damage in the asthmatic lung tissue by released eosinophil-derived cationic proteins, and eosinophilic inflammation is a hallmark of bronchial asthma [3132]. In the present study, our findings indicated that DBHP may inhibit eosinophilic allergic inflammation in human asthma [3334].
Of additional biological significance in other reports, is that DBHP, as a lipid-soluble phenolic antioxidant in edible vegetable oil, plays conflicting and complex role to inhibit lipid oxidation toward the oil-water interface and scavenge free radicals. In general, free radical scavenging activity of phenolic antioxidants improves as the number of hydroxyl and methyl groups [35]. It was also reported DBHP completely inhibited recombinant TNF-α-induced cytotoxicity in L929 cells, while another butylated hydroxytoluene compound had minimal effect due to the only different molecular structure of DBHP, which is a hydroxymethyl substituent instead of a hydroxyl group on the phenolic ring [36]. It is also well known that the lungs are always exposed to higher levels of oxygen than most other tissues, and toxic free radicals in human lungs have been implicated as an important pathologic factors in pulmonary disorders. An imbalance between the reducing and oxidizing systems is present in asthma, and this oxidative stress can trigger chronic inflammatory disorders including pulmonary diseases which can be counteracted by various antioxidant effects [3738]. We previously reported that flavones containing more hydroxyl radicals had greater anti-asthmatic effects in the asthmatic model [18], and this result is in agreement with other studies about the anti-asthmatic activities of natural antioxidant and anti-inflammatory products [39].
Collectively, in the present study, we showed that DBHP exerted significant anti-asthmatic effect in the guinea pigs model of OVA-induced asthmatic responses, and its inhibitory effect principally mediated by inhibiting the recruitments of the leukocytes and the release of biochemical inflammatory mediators from these infiltrated leukocytes, which leads to damage in the asthmatic lung. Moreover, the anti-asthmatic effect of DBHP may be mainly attributed to its potent antioxidant activity through the hydroxyl or methyl substituents are placed in the phenolic ring. In addition, our study might provide a novel insight into the pathological mechanisms underlying the inhibition of bronchial asthma via suppression of eosinophilic inflammatory mediators release for the development of new strategies in treatment of the eosinophilic bronchial asthma.
ACKNOWLEDGEMENTS
This research was supported by the Chung-Ang University Research Scholarship Grants in 2015.
Notes
References
1. Leick EA, Reis FG, Honorio-Neves FA, Almeida-Reis R, Prado CM, Martins MA, Tibério IF. Effects of repeated stress on distal airway inflammation, remodeling and mechanics in an animal model of chronic airway inflammation. Neuroimmunomodulation. 2012; 19:1–9. PMID: 22067616.


2. Hyung KE, Kim SJ, Jang YW, Lee DK, Hyun KH, Moon BS, Kim B, Ahn H, Park SY, Sohn UD, Park ES, Hwang KW. Therapeutic effects of orally administered CJLP55 for atopic dermatitis via the regulation of immune response. Korean J Physiol Pharmacol. 2017; 21:335–343. PMID: 28461776.


3. Matsumoto T, Ashida Y, Tsukuda R. Pharmacological modulation of immediate and late airway response and leukocyte infiltration in the guinea pig. J Pharmacol Exp Ther. 1994; 269:1236–1244. PMID: 8014867.
4. Sanjar S, Aoki S, Kristersson A, Smith D, Morley J. Antigen challenge induces pulmonary airway eosinophil accumulation and airway hyperreactivity in sensitized guinea-pigs: the effect of anti-asthma drugs. Br J Pharmacol. 1990; 99:679–686. PMID: 2361168.


5. Hanania NA. Targeting airway inflammation in asthma: current and future therapies. Chest. 2008; 133:989–998. PMID: 18398119.
6. Saji J, Yamamoto T, Arai M, Mineshita M, Miyazawa T. Efficacy of long-term omalizumab therapy in patients with severe asthma. Respir Investig. 2017; 55:114–120.


7. Alves MF, da Fonsec DV, de Melo SAL, Scotti MT, Scotti L, Dos Santos SG, de Fátima Formiga Melo Diniz M. New therapeutic targets and drugs for the treatment of asthma. Mini Rev Med Chem. 2017; 100:317.


8. Wex E, Thaler E, Blum S, Lamb D. A novel model of IgE-mediated passive pulmonary anaphylaxis in rats. PLoS One. 2014; 9:e116166. PMID: 25541997.


10. Ducharme FM, Ni Chroinin M, Greenstone I, Lasserson TJ. Addition of long-acting beta2-agonists to inhaled corticosteroids versus same dose inhaled corticosteroids for chronic asthma in adults and children. Cochrane Database Syst Rev. 2010; (5):CD005535. PMID: 20464739.


11. Jung CH, Lee JY, Cho CH, Kim CJ. Anti-asthmatic action of quercetin and rutin in conscious guinea-pigs challenged with aerosolized ovalbumin. Arch Pharm Res. 2007; 30:1599–1607. PMID: 18254248.


12. Moon H, Choi HH, Lee JY, Moon HJ, Sim SS, Kim CJ. Quercetin inhalation inhibits the asthmatic responses by exposure to aerosolized-ovalbumin in conscious guinea-pigs. Arch Pharm Res. 2008; 31:771–778. PMID: 18563360.


13. Suh SJ, Kwak CH, Chung TW, Park SJ, Cheeeei M, Park SS, Seo CS, Son JK, Chang YC, Park YG, Lee YC, Chang HW, Kim CH. Pimaric acid from Aralia cordata has an inhibitory effect on TNF-α-induced MMP-9 production and HASMC migration via down-regulated NF-κB and AP-1. Chem Biol Interact. 2012; 199:112–119. PMID: 22705379.
14. Pennock BE, Cox CP, Rogers RM, Cain WA, Wells JH. A noninvasive technique for measurement of changes in specific airway resistance. J Appl Physiol Respir Environ Exerc Physiol. 1979; 46:399–406. PMID: 422457.


15. Comas-Basté O, Latorre-Moratalla ML, Bernacchia R, Veciana-Nogués MT, Vidal-Carou MC. New approach for the diagnosis of histamine intolerance based on the determination of histamine and methylhistamine in urine. J Pharm Biomed Anal. 2017; 145:379–385. PMID: 28715791.


16. Song HS, Park SH, Ko MS, Jeong JM, Sohn UD, Sim SS. Morinda citrifolia inhibits both cytosolic Ca2+-dependent phospholipase A2 and secretory Ca2+-dependent phospholipase A2. Korean J Physiol Pharmacol. 2010; 14:163–167. PMID: 20631889.
17. Saeed A, Khan SU, Mahesar PA, Channar PA, Shabir G, Iqbal J. Substituted (E)-2-(2-benzylidenehydrazinyl)-4-methylthiazole-5-carboxylates as dual inhibitors of 15-lipoxygenase & carbonic anhydrase II: synthesis, biochemical evaluation and docking studies. Biochem Biophys Res Commun. 2017; 482:176–181. PMID: 27836541.
18. Lee JY, Kim JM, Kim CJ. Flavones derived from nature attenuate the immediate and late-phase asthmatic responses to aerosolized-ovalbumin exposure in conscious guinea pigs. Inflamm Res. 2014; 63:53–60. PMID: 24142298.


19. Antwi AO, Obiri DD, Osafo N. Stigmasterol modulates allergic airway inflammation in guinea pig model of ovalbumin-induced asthma. Mediators Inflamm. 2017; 2017:2953930. PMID: 28555089.


20. Hoymann HG. Lung function measurements in rodents in safety pharmacology studies. Front Pharmacol. 2012; 3:156. PMID: 22973226.


21. Chong BT, Agrawal DK, Romero FA, Townley RG. Measurement of bronchoconstriction using whole-body plethysmograph: comparison of freely moving versus restrained guinea pigs. J Pharmacol Toxicol Methods. 1998; 39:163–168. PMID: 9741391.


22. Jang TY, Jung AY, Kyung TS, Kim DY, Hwang JH, Kim YH. Anti-allergic effect of luteolin in mice with allergic asthma and rhinitis. Cent Eur J Immunol. 2017; 42:24–29. PMID: 28680328.


23. Ujino M, Sugimoto N, Koizumi Y, Ro S, Kojima Y, Asae KH, Yamashita N, Ohta K, Nagase H. Leukotriene receptor antagonist attenuated airway inflammation and hyperresponsiveness in a double-stranded RNA-induced asthma exacerbation model. Allergol Int. 2017; 66S:S21–S26. PMID: 28647381.


24. Yan S, Ci X, Chen N, Chen C, Li X, Chu X, Li J, Deng X. Anti-inflammatory effects of ivermectin in mouse model of allergic asthma. Inflamm Res. 2011; 60:589–596. PMID: 21279416.


25. Jang YW, Lee JY, Kim CJ. Anti-asthmatic activity of phenolic compounds from the roots of Gastrodia elata Bl. Int Immunopharmacol. 2010; 10:147–154. PMID: 19874915.
26. Xavier CV, da S Setúbal S, Lacouth-Silva F, Pontes AS, Nery NM, de Castro OB, Fernandes CFC, Soares AM, Fortes-Dias CL, Zuliani JP. Phospholipase A2 inhibitor from Crotalus durissus terrificus rattle-snake: Effects on human peripheral blood mononuclear cells and human neutrophils cells. Int J Biol Macromol. 2017; 105:1117–1125. PMID: 28743568.
27. Keapai W, Apichai S, Amornlerdpison D, Lailerd N. Evaluation of fish oil-rich in MUFAs for anti-diabetic and anti-inflammation potential in experimental type 2 diabetic rats. Korean J Physiol Pharmacol. 2016; 20:581–593. PMID: 27847435.


28. Lukic A, Ji J, Idborg H, Samuelsson B, Palmberg L, Gabrielsson S, Radmark O. Pulmonary epithelial cancer cells and their exosomes metabolize myeloid cell-derived leukotriene C4 to leukotriene D4. J Lipid Res. 2016; 57:1659–1669. PMID: 27436590.


29. Kim HJ, Yoo HY. Hypoxic pulmonary vasoconstriction and vascular contractility in monocrotaline-induced pulmonary arterial hypertensive rats. Korean J Physiol Pharmacol. 2016; 20:641–647. PMID: 27847441.


30. Sultan MT, Li HM, Lee YZ, Lim SS, Song DK. Identification of Lys49-PLA2 from crude venom of Crotalus atrox as a human neutrophil-calcium modulating protein. Korean J Physiol Pharmacol. 2016; 20:177–183. PMID: 26937214.
31. Min YD, Choi CH, Bark H, Son HY, Park HH, Lee S, Park JW, Park EK, Shin HI, Kim SH. Quercetin inhibits expression of inflammatory cytokines through attenuation of NF-κB and p38 MAPK in HMC-1 human mast cell line. Inflamm Res. 2007; 56:210–215. PMID: 17588137.


32. Li X, Shen Y, Lu Y, Yang J. Amelioration of bleomycin-induced pulmonary fibrosis of rats by an aldose reductase inhibitor, epalrestat. Korean J Physiol Pharmacol. 2015; 19:401–411. PMID: 26330752.


33. Li N, Qiu R, Yang Z, Li J, Chung KF, Zhong N, Zhang Q. Sputum microbiota in severe asthma patients: relationship to eosinophilic inflammation. Respir Med. 2017; 131:192–198. PMID: 28947029.


34. Baldissera L Jr, Squebola-Cola DM, Calixto MC, Lima-Barbosa AP, Rennó AL, Anhê GF, Condino-Neto A, De Nucci G, Antunes E. The soluble guanylyl cyclase activator BAY 60-2770 inhibits murine allergic airways inflammation and human eosinophil chemotaxis. Pulm Pharmacol Ther. 2016; 41:86–95. PMID: 27816773.
35. Chaiyasit W, McClements DJ, Decker EA. The relationship between the physicochemical properties of antioxidants and their ability to inhibit lipid oxidation in bulk oil and oil-in-water emulsions. J Agric Food Chem. 2005; 53:4982–4988. PMID: 15941345.


36. Brekke OL, Shalaby MR, Sundan A, Espevik T, Bjerve KS. Butylated hydroxyanisole specifically inhibits tumor necrosis factor-induced cytotoxicity and growth enhancement. Cytokine. 1992; 4:269–280. PMID: 1515551.


37. Halliwell B. Free radicals, antioxidants, and human disease: curiosity, cause, or consequence. Lancet. 1994; 344:721–724. PMID: 7915779.


38. Houssen ME, Ragab A, Mesbah A, El-Samanoudy AZ, Othman G, Moustafa AF, Badria FA. Natural anti-inflammatory products and leukotriene inhibitors as complementary therapy for bronchial asthma. Clin Biochem. 2010; 43:887–890. PMID: 20430018.


Fig. 1
Chemical structure of 2,6-di-tert-bytyl-4-hydroxymethylphenol (A) and diagram of the double-chambered plethysmograph (B) used in this experiment.
This double-chambered plethysmograph is a basic method for measurement of the specific airway resistance (sRaw) together with the standard parameters tidal volume and respiratory rate in conscious animals placed into the plethysmograph box (HSE type 855, Hugo Sachs Elektronik, Germany). The phase shift between two the nasal and thoracic respiratory flows is measured with two differential pressure transducers (PT5, Grass Instument Co., USA). The PULMODYN “PENNOCK 89” software is able to record the signals and calculate the sRaw with a respiratory analyzer (7E polygraph, Grass Instument Co., USA), and the PLUGSYS 603 system is used to control the valves from the plethysmograph boxes and interface the boxes to the computer (This diagram modified from an original kindly provided by Hugo Sachs Elektronik company, Germany).
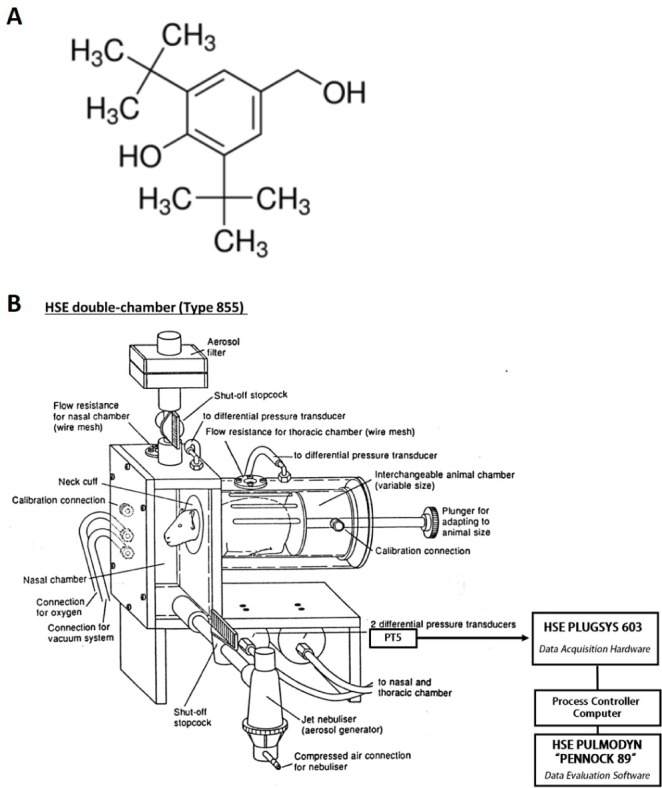
Fig. 2
Effect of DBHP on specific airway resistance (sRaw) to the antigen challenge in IAR (A) and LAR (B).
Test drugs were orally administered 1 h prior to OVA challenge, additionally 12 h later. Percentage increase of sRaw (%)=[(sRaw after OVA challenge/sRaw before OVA challenge)−1]×100. Each value represents the mean±SEM (n=6). Values are statistically significant at #p<0.05 compared with the vehicle control; *p<0.05 and **p<0.01 compared with OVA control. DBHP, 2,6-di-tert-butyl-4-hydroxymethylphenol; DEX, dexamethasone; CRO, cromoglycate; SAL, salbutamol.
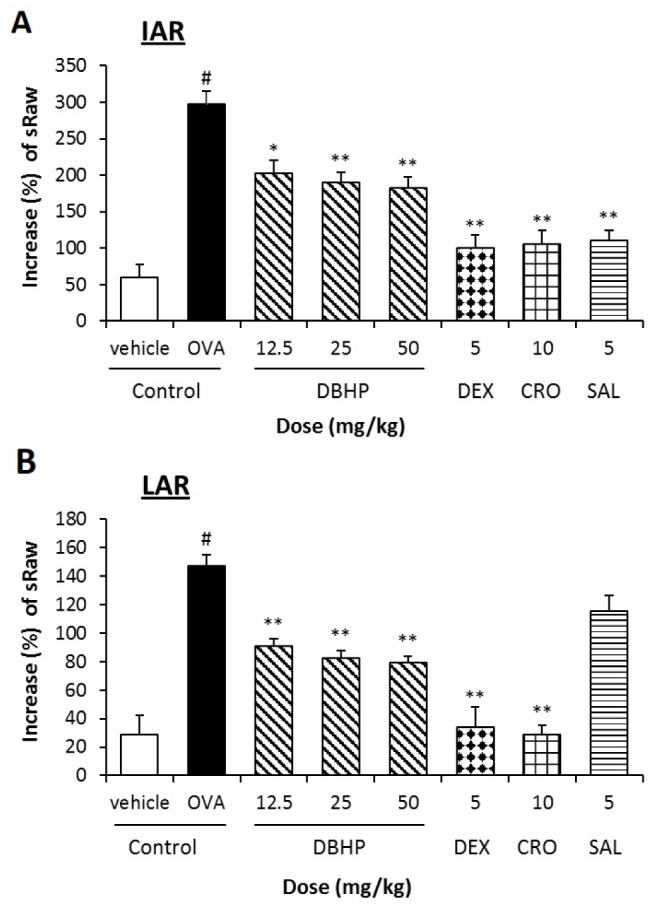
Fig. 3
Effect of DBHP on the tidal volume (TV) to the antigen challenge in IAR (A) and LAR (B).
Test drugs were orally administered 1 h prior to OVA challenge, additionally 12 h later. Percentage decrease of TV (%)=[1−(TV after OVA challenge/TV before OVA challenge)]×100. Each value represents the mean±SEM (n=6). Values are statistically significant at #p<0.05 compared with the vehicle control; *p<0.05 and **p<0.01 compared with OVA control. DBHP, 2,6-di-tert-butyl-4-hydroxymethylphenol; DEX, dexamethasone; CRO, cromoglycate; SAL, salbutamol.
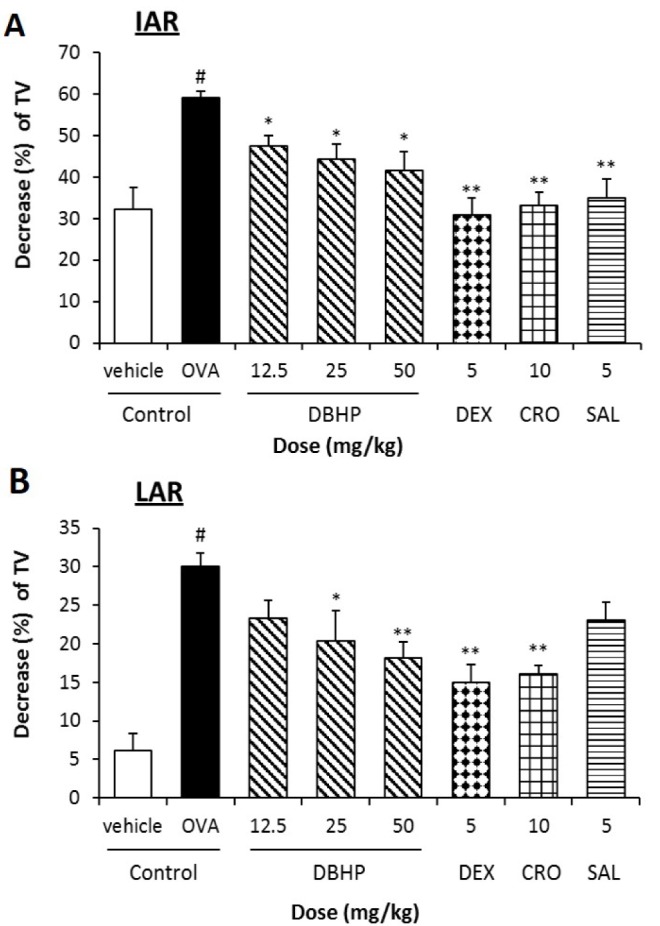
Fig. 4
Effect of DBHP on the recruitment of leukocytes in BALF.
The number of total leukocytes (A), eosinophils (B), and neutrophils (C) were counted in BALF. Test drugs were orally administered 1 h prior to OVA challenge, additionally 12 h later. Each value represents the mean±SEM (n=6). Values are statistically significant at #p<0.05 compared with the vehicle control; *p<0.05 and **p<0.01 compared with OVA control. DBHP, 2,6-di-tert-butyl-4-hydroxymethylphenol; DEX, dexamethasone; CRO, cromoglycate; SAL, salbutamol.
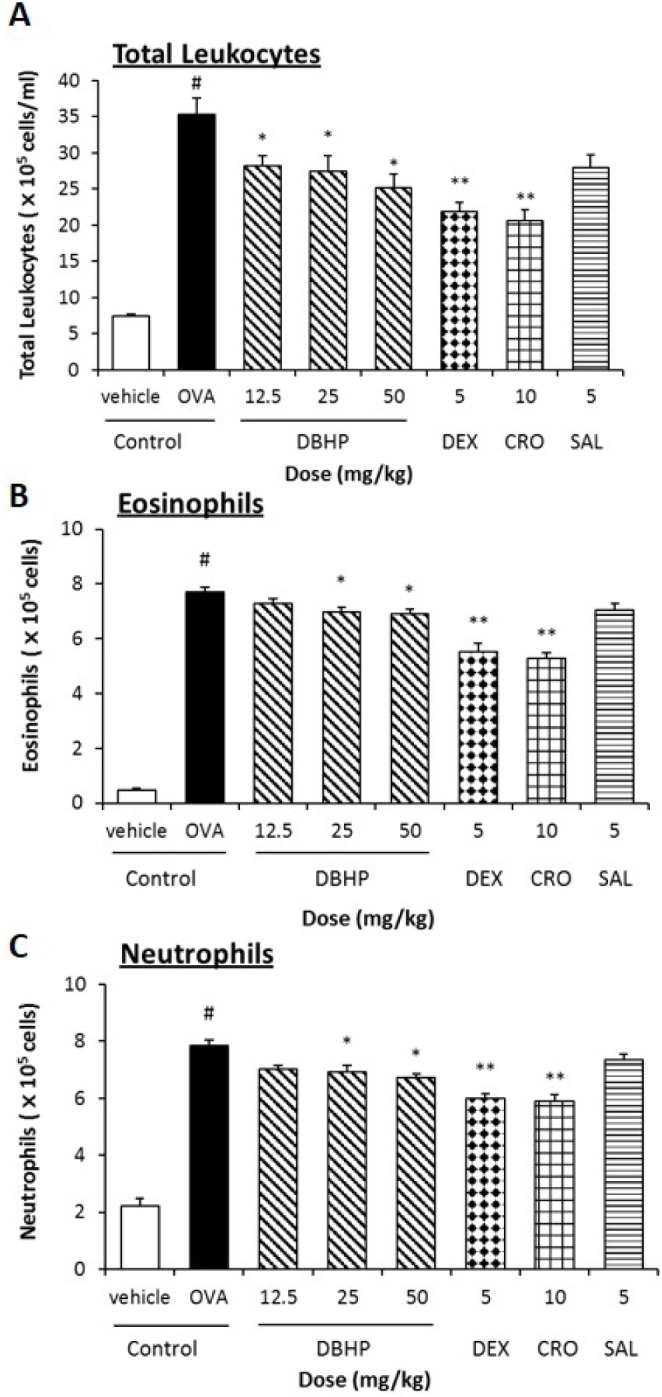
Fig. 5
Effect of DBHP on the release of biochemical mediators in BALF.
Histamine content (A), protein exudates (B), and the specific activity of PLA2 (C) were measured fluorometrically and colorimetrically in BALF. Test drugs were orally administered 1 h prior to OVA challenge, additionally 12 h later. Each value represents the mean±SEM (n=6). Values are statistically significant at #p<0.05 compared with the vehicle control; *p<0.05 and **p<0.01 compared with OVA control. DBHP, 2,6-di-tert-butyl-4-hydroxymethylphenol; DEX, dexamethasone; CRO, cromoglycate; SAL, salbutamol.
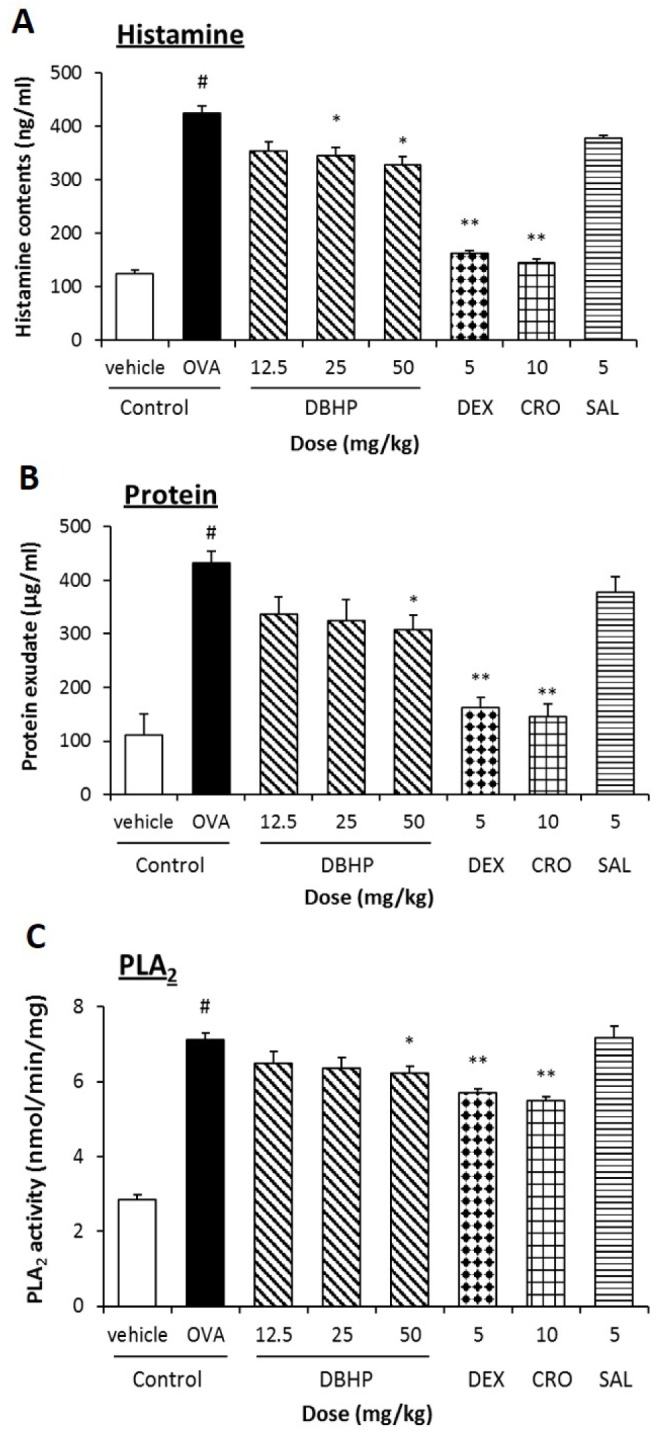
Fig. 6
Effect of DBHP on the histopathological survey in lung tissue.
Representative images of the H&E-stained sections of lung tissue from; (A) vehicle control, showed a normal architecture and non-infiltration of inflammatory cells. (B) OVA control, exhibited the recruitment of leukocytes in alveolar sac (black arrow), peripheral vasculature (red arrow) and terminal bronchioles (yellow arrow). (C) asthmatic lung tissue treated with DBHP (50 mg/kg, p.o.) at 1 h before, and 12 h after OVA challenge, was reduced the recruitment of leukocytes into alveolar sacs (pink arrow). (D) asthmatic lung tissue treated with dexamethasone (5 mg/kg, p.o.) at 1 h before, and 12 h after OVA challenge, was also improved pathological changes with mild infiltration of leukocytes around bronchioles (purple arrow) (original magnification; ×100).
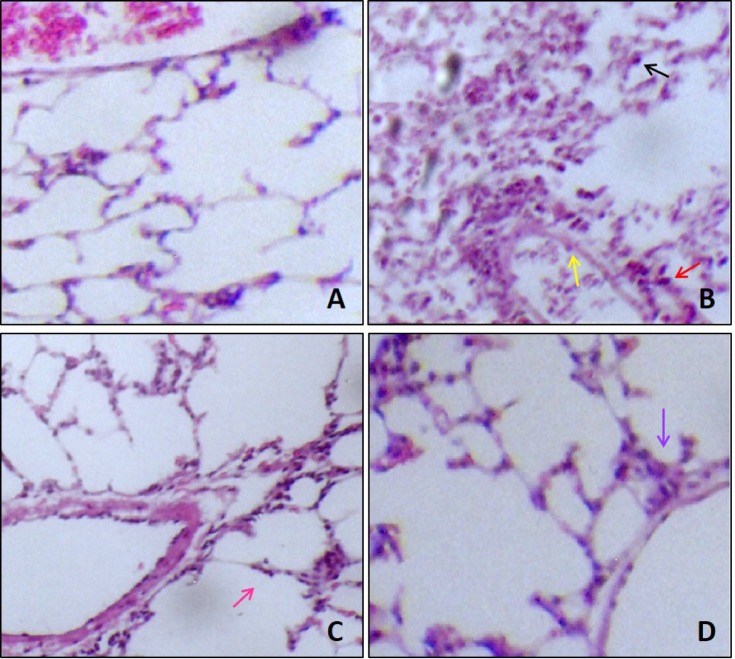