Abstract
Our previous studies have confirmed that morroniside has neuroprotective effects. However, the effects of morroniside on cardiac myocardium remain unknown. Rats were anaesthetized with 10% chloral hydrate (0.35~0.4 mL/kg) and an acute myocardial infarction (AMI) was induced by ligating the anterior descending coronary artery (LAD). Following AMI, morroniside was administered intragastrically for 3 consecutive days at doses of 45, 90 and 180 mg/kg, respectively. Lactate dehydrogenase (LDH) and cardiac troponin T (cTnT) activities in AMI rats in the serum were detected with commercial kits. The expression of IL-6, IL-1β and TNF-α in myocardium was detected by Western blotting analysis. We observed a significant decline in the Q(q) wave amplitude in morroniside-treated rats after 72 h. Additionally, treatment of morroniside decreased the levels of LDH and cTnT in AMI rats. We also observed that morroniside reduced the expression of IL-6, IL-1β and TNF-α in myocardium. Taken together, our findings demonstrate that morroniside had effective anti-inflammatory properties in AMI rats.
Worldwide, acute myocardial infarction (AMI) is a severe disease with high mortality. AMI is accompanied by inflammatory responses that lead to the recruitment of leukocytes and subsequent myocardial damage and scar formation [1]. Several studies have shed a new light on both the positive and negative roles of inflammation in processes of tissue repair and regeneration [2]. Although inflammation plays a critical role in cellular debris removal and the formation of new tissue after ischemic injury, exaggerated inflammation may obstruct the healing process, as shown in ApoE–/– mice with coronary artery ligation [34]. Inflammation plays an important role in the development of myocardial infarction (MI) [25].
Morroniside extracted from Cornus officinalis is one of the most abundant iridoid glycosides. It has been shown that morroniside not only ameliorates anti-oxidative and anti-apoptotic effects in vitro, but that it also improves neurological functional recovery in vivo [678910]. The effects of morroniside on cardiac myocardium have not been comprehensibly explored. In the present study, we investigated whether morroniside could reduce myocardial inflammation in AMI rats.
This study was approved by the Animal Care and Use Committee of Xuanwu Hospital in Capital Medical University. The animals were housed under a 12/12 h dark/light cycle with food and water under specific pathogen-free (SPF) conditions to ensure physical and psychological well-being. All animals were anesthetized throughout surgery and post-operative care was provided after the surgery.
Adult male Sprague-Dawley (SD) rats (250 to 280 g) were acquired from Beijing Vitalriver Experimental Animal Co. (Beijing, China).
As described previously [1112], rats were anesthetized by intraperitoneal injection of 10% chloral hydrate (0.35~0.4 mL/kg), mechanically ventilated with a small animal ventilator (Harvard 683, U.S.A) at 50 breaths/min. The inspiratory/expiratory ratio was set to 1:2 and the tidal volume was adjusted to 2~3 mL/100 g body weight. Thoracotomy was performed through the fourth intercostals space and the left anterior descending coronary artery was ligated 2~3 mm away from its origin between the pulmonary artery conus and the left atrium with 6.0 prolene suture. Successful coronary occlusion was verified by observing the development of a pale color in the distal myocardium and ST segment elevation on ECG after ligation.
SD rats that were subjected to the described AMI model were candidates for morroniside administration. Immediately following regaining of consciousness post AMI, morroniside, dissolved in ddH2O, was administered intragastrically once daily for 3 days at doses of 45, 90 and 180 mg/kg, respectively. In sham-operated animals the LAD artery was not ligating. The vehicle control groups of AMI rats and sham-operated rats received an equal volume of normal saline.
Rats were anesthetized with 10% chloral hydrate and needle electrodes were inserted into the lead II position. Electrocardiograms (ECG) of the experimental animals were recorded (MP150, BIOPAC Co., U.S.A).
Serum samples of rats were obtained from aorta abdominals after ligation for 72 h. The LDH and cTnT activities in the serum of rats were detected by respective commercial kits (JianCheng Bioengineering, Nanjing, China).
After day 3 post AMI, each rat was sacrificed under 10% chloral hydrate (0.35~0.4 mL/kg) anesthesia and its heart was harvested for western blotting procedures. Myocardial proteins were extracted using RIPA Lysis Buffer (Beyotime Inc., China) and 1% phenylmethanesulfonyl fluoride (PMSF). Following homogenization of myocardial tissues and ultrasonic grinding to no precipitation, the samples were centrifuged at 12,000×g at 4℃ for 30 min and the supernatants were collected as total protein. All the steps above were carried out on the ice, to avoid protein denaturation. Protein concentrations were determined by using a bicinchoninic acid (BCA) protein assay kit (applygen, China). Protein samples (40 µg/lane) were separated by 15% SDS-PAGE and electrically transferred onto a nitrocellulose membrane (250 mA, 1 h). The membranes were blocked with 5% nonfat milk solution for 2 h at room temperature and then incubated with primary antibody against IL-6 (1:1000 dilution, abcam, ab9324), TNF-α (1:1000 dilution, abcam, ab11564), IL-1β (1:1000 dilution, abcam, ab9722) and GAPDH (1:1000 dilution, CST, #5174) in Tris-buffered saline Tween-20 (TBST) containing 5% nonfat milk solution overnight at 4℃. Membranes were washed 3 times for 10 min each with TBST solution and incubated with secondary antibody (1:4000 dilution, ZSGB-BIO, China) at room temperature for 2 h. The transferred proteins were visualized by an enhanced chemiluminescence (ECL) system (FluorChemHD2, ProteinSimple Co., U.S.A). All bands of interest were quantified and normalized to their respective GAPDH bands.
Fig. 2 illustrates that normal rats did not show any changes in ECG pattern. AMI rats showed a significant elevation in ST segments on ECG compared to sham controls, suggesting that modeling was successful. Our data demonstrate that at 72 h following AMI, morroniside treatment resulted in a significant decline in Q(q) wave amplitude. Early post-AMI, total Q-wave amplitude (QWAmp) independently correlated with infarct size [13]. Large infarct size increased immediate morbidity and mortality and, in rats that survived this acute event, larger non-functional scars adversely affect long-term prognosis [14].
It was found that the activities of LDH and cTnT were increased in the serum in AMI rats, compared with the sham group. Treatment with morroniside (45, 90 and 180 mg/kg) for 3 days reduced the levels of LDH and cTnT, compared with the vehicle group (Fig. 3). This finding indicated that morroniside was effective for the treatment of AMI in rats.
As shown in Fig. 4, Western blot analysis 3 days post AMI showed that the expression of IL-6, IL-1β and TNF-α was remarkably reduced by morroniside treatment (180 mg/kg), 73%, 57% and 67%, respectively (1.86±0.67 vs. 0.51±0.19, 1.13±0.15 vs. 0.49±0.13, 1.52±0.39 vs. 0.50±0.18; p<0.05, n=40) compared to the vehicle-treated AMI rats. The expressions of IL-6, IL-1β and TNF-α were increased by 60%, 65% and 86%, respectively (0.74±0.36 vs. 1.86±0.67, 0.39±0.11 vs. 1.13±0.15, 0.21±0.14 vs. 1.52±0.39; p<0.05, n=40) in the vehicle group compared to the sham-treated rats. Taken together, this data provides evidence that morroniside might promote anti-inflammatory effects via the suppression of inflammatory cytokines IL-6, IL-1β and TNF-α.
It has been shown that C. officinalis plays important roles in the regulation of the anti-oxidative, anti-diabetic and anti-inflammatory immune response [15]. Previous studies have characterized the potent acute neuroprotective effects of morroniside, a primary active ingredient extracted from the Chinese herb C. officinalis (Chinese name, Shan-zhu-yu), following both an oxidative stress-induced neurotoxic cell death model and a focal cerebral ischemia rat model [678910]. Here, we focused on the role of morroniside treatment in a preclinical rat model of AMI, contributing novel findings on the anti-inflammatory activities of morroniside.
After AMI, inflammatory cytokines such as IL-6 [16], IL-1β and TNF-α were reported to be elevated and their increasing levels was closely correlated to disease severity and prognosis of the patients. IL-6, with its extensive bioactivities, causes various biological effects in the process of inflammatory reaction, anti-infection and injury [17]. In the fast revascularization during instability in coronary artery disease (FRISC-II) trial, IL-6 was related to increased risk of mortality among patients presenting with AMI. Among patients with elevated IL-6 levels, early invasive strategy prompted a significant 65% decrease in mortality at 1 year after treatment [18]. The secondary prevention cholesterol and recurrent events (CARE) trial studies reported that TNF-α has been associated with an elevated risk of recurrent MI and cardiovascular death after a first MI [18]. TNF-α production is increased in unstable atherosclerotic plaques [1819] and is detectable in increased concentrations in patients with AMI [1820]. Furthermore, plasma TNF-α concentrations are predictive of death and recurrent ischemic events following AMI [1821]. Clinical studies demonstrated that the level of IL-6, IL-1β and TNF-α increased dramatically in the circulatory and myocardial tissue of patients with heart failure, which were positively correlated with the severity of the condition. The previous experiment verified that TNF-α and IL-1β impair the myocardial systolic functions in rats and exert toxic effects on cultured cardiocytes [22]. Inflammatory cytokines may reduce matrix synthesis and increase its degradation, favoring plaque rupture [23]. Canakinumab is a human monoclonal antibody that selectively neutralizes IL-1β. The Canakinumab Anti-inflammatory Thrombosis Outcomes Study (CANTOS) examines how blocking IL-1β with canakinumab lowers recurrence rates of myocardial infarction, stroke and cardiovascular disease [2425].
In summary, our findings suggest that morroniside could reduce IL-6, IL-1β and TNF-α levels. As the results of our Western blot shown, anti-inflammation mechanisms might be involved in the cardioprotection of morroniside treatment post AMI.
ACKNOWLEDGEMENTS
This study was supported by the National Science and Technology Major Project (2012ZX09102201-106); National Natural Science Foundation of China (81373994, 81573633, 81503049, 81173575).
References
1. Morimoto H, Takahashi M, Izawa A, Ise H, Hongo M, Kolattukudy PE, Ikeda U. Cardiac overexpression of monocyte chemoattractant protein-1 in transgenic mice prevents cardiac dysfunction and remodeling after myocardial infarction. Circ Res. 2006; 99:891–899. PMID: 16990567.


2. Xin M, Olson EN, Bassel-Duby R. Mending broken hearts: cardiac development as a basis for adult heart regeneration and repair. Nat Rev Mol Cell Biol. 2013; 14:529–541. PMID: 23839576.


3. Panizzi P, Swirski FK, Figueiredo JL, Waterman P, Sosnovik DE, Aikawa E, Libby P, Pittet M, Weissleder R, Nahrendorf M. Impaired infarct healing in atherosclerotic mice with Ly-6C(hi) monocytosis. J Am Coll Cardiol. 2010; 55:1629–1638. PMID: 20378083.
4. Dutta P, Nahrendorf M. Monocytes in myocardial infarction. Arterioscler Thromb Vasc Biol. 2015; 35:1066–1070. PMID: 25792449.


5. Lindahl B, Toss H, Siegbahn A, Venge P, Wallentin L. Markers of myocardial damage and inflammation in relation to long-term mortality in unstable coronary artery disease. FRISC Study Group. Fragmin during Instability in Coronary Artery Disease. N Engl J Med. 2000; 343:1139–1147. PMID: 11036119.
6. Wang W, Huang W, Li L, Ai H, Sun F, Liu C, An Y. Morroniside prevents peroxide-induced apoptosis by induction of endogenous glutathione in human neuroblastoma cells. Cell Mol Neurobiol. 2008; 28:293–305. PMID: 17647102.


7. Wang W, Sun F, An Y, Ai H, Zhang L, Huang W, Li L. Morroniside protects human neuroblastoma SH-SY5Y cells against hydrogen peroxide-induced cytotoxicity. Eur J Pharmacol. 2009; 613:19–23. PMID: 19379729.


8. Wang W, Xu J, Li L, Wang P, Ji X, Ai H, Zhang L, Li L. Neuroprotective effect of morroniside on focal cerebral ischemia in rats. Brain Res Bull. 2010; 83:196–201. PMID: 20637265.


9. Sun FL, Wang W, Zuo W, Xue JL, Xu JD, Ai HX, Zhang L, Wang XM, Ji XM. Promoting neurogenesis via Wnt/β-catenin signaling pathway accounts for the neurorestorative effects of morroniside against cerebral ischemia injury. Eur J Pharmacol. 2014; 738:214–221. PMID: 24876057.


10. Sun FL, Wang W, Cheng H, Wang Y, Li L, Xue JL, Wang XF, Ai HX, Zhang L, Xu JD, Wang XM, Ji XM. Morroniside improves microvascular functional integrity of the neurovascular unit after cerebral ischemia. PLoS One. 2014; 9:e101194. PMID: 24979385.


11. D'Uva G, Aharonov A, Lauriola M, Kain D, Yahalom-Ronen Y, Carvalho S, Weisinger K, Bassat E, Rajchman D, Yifa O, Lysenko M, Konfino T, Hegesh J, Brenner O, Neeman M, Yarden Y, Leor J, Sarig R, Harvey RP, Tzahor E. ERBB2 triggers mammalian heart regeneration by promoting cardiomyocyte dedifferentiation and proliferation. Nat Cell Biol. 2015; 17:627–638. PMID: 25848746.
12. Selye H, Bajusz E, Grasso S, Mendell P. Simple techniques for the surgical occlusion of coronary vessels in the rat. Angiology. 1960; 11:398–407. PMID: 13749954.


13. Kochav JD, Okin PM, Wilson S, Afroz A, Renilla A, Weinsaft JW. Usefulness of Q-wave area for threshold-based stratification of global left ventricular myocardial infarct size. Am J Cardiol. 2013; 112:174–180. PMID: 23611753.


14. Pepys MB, Hirschfield GM, Tennent GA, Gallimore JR, Kahan MC, Bellotti V, Hawkins PN, Myers RM, Smith MD, Polara A, Cobb AJ, Ley SV, Aquilina JA, Robinson CV, Sharif I, Gray GA, Sabin CA, Jenvey MC, Kolstoe SE, Thompson D, Wood SP. Targeting C-reactive protein for the treatment of cardiovascular disease. Nature. 2006; 440:1217–1221. PMID: 16642000.


15. Ya BL, Li CY, Zhang L, Wang W, Li L. Cornel iridoid glycoside inhibits inflammation and apoptosis in brains of rats with focal cerebral ischemia. Neurochem Res. 2010; 35:773–781. PMID: 20155318.


16. Andrie RP, Becher UM, Frommold R, Tiyerili V, Schrickel JW, Nickenig G, Schwab JO. Interleukin-6 is the strongest predictor of 30-day mortality in patients with cardiogenic shock due to myocardial infarction. Crit Care. 2012; 16:R152. PMID: 22889197.
17. Ding C, Cicuttini F, Li J, Jones G. Targeting IL-6 in the treatment of inflammatory and autoimmune diseases. Expert Opin Investig Drugs. 2009; 18:1457–1466.


18. Tedgui A, Mallat Z. Cytokines in atherosclerosis: pathogenic and regulatory pathways. Physiol Rev. 2006; 86:515–581. PMID: 16601268.


19. Satoh M, Ishikawa Y, Itoh T, Minami Y, Takahashi Y, Nakamura M. The expression of TNF-alpha converting enzyme at the site of ruptured plaques in patients with acute myocardial infarction. Eur J Clin Invest. 2008; 38:97–105. PMID: 18226043.
20. Valgimigli M, Ceconi C, Malagutti P, Merli E, Soukhomovskaia O, Francolini G, Cicchitelli G, Olivares A, Parrinello G, Percoco G, Guardigli G, Mele D, Pirani R, Ferrari R. Tumor necrosis factor-alpha receptor 1 is a major predictor of mortality and new-onset heart failure in patients with acute myocardial infarction: the Cytokine-Activation and Long-Term Prognosis in Myocardial Infarction (C-ALPHA) study. Circulation. 2005; 111:863–870. PMID: 15699251.
21. Padfield GJ, Din JN, Koushiappi E, Mills NL, Robinson SD, Cruden Nle M, Lucking AJ, Chia S, Harding SA, Newby DE. Cardiovascular effects of tumour necrosis factor α antagonism in patients with acute myocardial infarction: a first in human study. Heart. 2013; 99:1330–1335. PMID: 23574969.


22. Wu W, Huang L, Zhang J, Gao Y, Yang Y. High-frequency ultrasound evaluation of effects of early treatment with metoprolol on myocardial inflammatory cytokine expression in rats with acute myocardial infarction. J Huazhong Univ Sci Technolog Med Sci. 2012; 32:774–778. PMID: 23073812.


23. Mulvihill NT, Foley JB. Inflammation in acute coronary syndromes. Heart. 2002; 87:201–204. PMID: 11847151.


24. Ridker PM, Thuren T, Zalewski A, Libby P. Interleukin-1β inhibition and the prevention of recurrent cardiovascular events: rationale and design of the Canakinumab Anti-inflammatory Thrombosis Outcomes Study (CANTOS). Am Heart J. 2011; 162:597–605. PMID: 21982649.


25. De Caterina R, D'Ugo E, Libby P. Inflammation and thrombosis -testing the hypothesis with anti-inflammatory drug trials. Thromb Haemost. 2016; 116:1012–1021. PMID: 27535617.
Fig. 2
Electrocardiogram (lead II).
(A) Normal (sham) ECG, (B) 5 min post AMI, (C) Model, 72 h post AMI, (D) Morroniside-treated, 72 h post AMI, (E) Amplitude of Q(q) wave (ECG) post AMI 72 h (model and morroniside-treated AMI rats). n=8 per group, *p<0.05 vs. model.
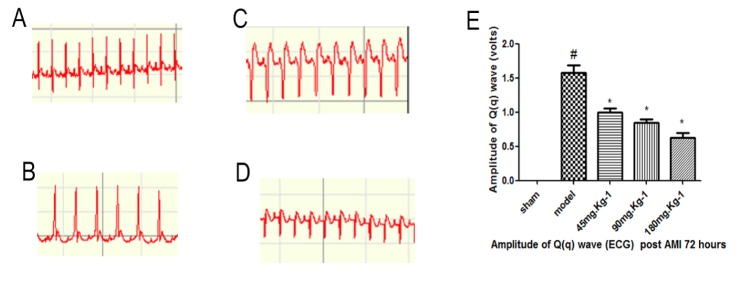