Abstract
The subgranular zone (SGZ) of hippocampal dentate gyrus (HDG) is a primary site of adult neurogenesis. Toll-like receptors (TLRs), are involved in neural system development of Drosophila and innate immune response of mammals. TLR2 is expressed abundantly in neurogenic niches such as adult mammalian hippocampus. It regulates adult hippocampal neurogenesis. However, the role of TLR2 in adult neurogenesis is not well studied in global or focal cerebral ischemia. Therefore, this study aimed to investigate the role of TLR2 in adult neurogenesis after photochemically induced cerebral ischemia. At 7 days after photothrombotic ischemic injury, the number of bromodeoxyuridine (BrdU)-positive cells was increased in both TLR2 knock-out (KO) mice and wild-type (WT) mice. However, the increment rate of BrdU-positive cells was lower in TLR2 KO mice compared to that in WT mice. The number of doublecortin (DCX) and neuronal nuclei (NeuN)-positive cells in HDG was decreased after photothrombotic ischemia in TLR2 KO mice compared to that in WT mice. The survival rate of cells in HDG was decreased in TLR2 KO mice compared to that in WT mice. In contrast, the number of cleaved-caspase 3 (apoptotic marker) and the number of GFAP (glia marker)/BrdU double-positive cells in TLR2 KO mice were higher than that in WT mice. These results suggest that TLR2 can promote adult neurogenesis from neural stem cell of hippocampal dentate gyrus through increasing proliferation, differentiation, and survival from neural stem cells after ischemic injury of the brain.
New neurons are generated from neural stem cells (NSC) throughout adulthood [1]. The generation of new neurons in adult brain is mostly associated with two regions: the subventricular zone (SVZ) of lateral ventricles and the subgranular zone (SGZ) of hippocampal dentate gyrus (HDG) [23]. NSCs in SVZ and SGZ are self-renewing. They are multipotent cells that give rise to neurons, astrocytes, and oligodendrocytes. They seem to have astrocyte like appearance. NSCs are known to express vimentin and glial fibrillary acid protein (GFAP) [45]. Neural progenitors and stem cells (NSCs) of the SVZ can differentiate into neuroblasts. They can migrate a long distance tangentially through rostral migratory stream and differentiate into interneurons in the olfactory bulb (OB). However, NSCs of the hippocampal SGZ can only migrate a short distance into granular cell layer where they differentiate into projection neurons [6].
Adult neurogenesis from HDG and SVZ can be divided into five stages: 1) proliferation, NSCs give rise to transiently amplifying cells and proliferating progenitor cells tightly associated with astrocytes and vascular structure; 2) differentiation and survival, transiently amplifying cells differentiate into immature neuron; 3) migration, immature neurons migrate a short distance into hippocampal granular cell layer and OB; 4) axon/dendrite targeting, immature neurons extend their projecting axon and short dendrites, and 5) synaptic integration, new granular neurons make synaptic integrations with other neurons [78]. Recent reports have shown that new neurons generated from neural stem cells might play pivotal roles in cognition and brain repair. For example, water maze exercise and enriched environment associated with improved memory function and synaptic plasticity can enhance adult neurogenesis in HDG [9]. In addition, adult neurogenesis is increased from HDG and SVZ after brain stroke, selective lesion, and seizure [1011]. However, roles of newborn neurons derived from HDG and SVG are currently unclear.
Toll-like receptors (TLRs), hallmarks of innate immunity, are increasingly implemented in central nervous system (CNS) plasticity-related processes including neurogenesis. They exhibit differential expression patterns in the brain with various functions in development. Critical roles of TLR3 [12] in NSCs and TLR4 [13] in microglia modulated proliferation and neural differentiation have been shown in adult brain [14]. TLR8 regulates neurotoxicity related to neural development [15] while TLR9 attenuates neurogenesis after seizure induced aberrant hippocampal neurogenesis [16]. Expression pattern of TLRs during brain development has also demonstrated roles of TLRs in neuronal plasticity and neurogenesis [17]. TLR2 is expressed in the central nervous system (CNS) [18] and peripheral nervous system (PNS) [19]. A recent study has shown that TLR2 is expressed abundantly in neurogenic niches of adult brain such as the hippocampus [20] of mammalian. It regulates adult hippocampal neurogenesis [21]. TLR2 can induce pro-inflammatory chemokines and pro-apoptotic proteins affected by microenvironment or damage [222324].
Transient whole brain ischemia can induce neuronal cell death in the brain [2526], notably in the hippocampal dentate gyrus. Adult neurogenesis in the dentate SGZ is affected by various physiological conditions, including CNS diseases, ischemia, epileptic, and seizure [2728]. Recent reports have shown that expression levels of TLR2 protein and mRNA in ischemic mouse brain are upregulated in TLR2 KO mice compared to those in WT mice following cerebral focal ischemia injury, while TLR4 KO mice exhibit reduced infarct size [2930]. Previously, we have shown that TLR4 plays a critical role in adult hippocampal neurogenesis of inflammatory-related neurological disorders [31]. However, the role of TLR2 in adult neurogenesis in global or focal cerebral ischemia is not well studied yet.
Thus, the objective of the present study was to elucidate the role of TLR2 in adult neurogenesis from neural stem cells of HDG in photochemical-induced cerebral ischemia.
All experiments were approved by the Animal Care and Use Committee of Chonnam National University. TLR2 knock-out (TLR2 KO) mice with C57BL/6 background mice were provided by Dr. Akira (University of Tokyo, Japan). Seven-week-old male C57BL/6 mice were purchased from Daehan Biolink (Chungbuk, Korea). Male C57BL/6 mice (body weight, 15–20 g) were caged in an air-conditioned room maintained at 22±2℃ with relative humidity of 50±10% and a 12/12 h light/dark cycle. Animals were provided free access to tap water and conventional mouse chow diet. They were acclimated for one week prior to the beginning of the study. Average age of mice used in this study was 7 weeks. Mice were divided into the following four groups: 1) Wild type, 2) TLR2 KO, 3) Wild type with photochemical-induced cerebral ischemia, and 4) TLR2 KO with photochemical-induced cerebral ischemia mice (n=5 mice/group).
Photothrombosis was performed using published procedure [32] with slight modifications. Briefly, Rose Bengal (Sigma, St. Louis, MO, USA) was dissolved in sterile saline at a concentration of 10 mg/ml. Then 0.1 ml of solution containing 1 mg of Rose Bengal was injected intravenously to each mouse five minutes before illumination. Mice were placed in a stereotactic frame. Their rectal temperature was monitored and controlled at 36.5–37.5℃ using a heating pad. At the dorsal aspect of the head, the skull was exposed by a median incision of the skin. The periosteum was gently removed to identify bregma and lambda points. Then 532 nm green laser light (1.5 mm aperture) was centered at 2 mm posterior and 2 mm laterally from the bregma. The duration of laser exposure was 10 minutes. Cerebral infarction was confirmed by 2,3,5-triphenyltetrazolium chloride staining on the next day.
Bromodeoxyuridine (BrdU; Sigma, St. Louis, MO, USA) was dissolved in physiologic saline containing 0.9% NaCl and injected intraperitoneally into mice (50 mg/kg). To examine proliferation of neural stem cells in hippocampal DG, BrdU was injected once daily into TLR2 KO mice and WT mice. Mice were then sacrificed at three hours after BrdU injection. To study the survival of newborn cells from neural stem cells and differentiation rate of neural stem cells, BrdU was injected once daily for five consecutive days. Mice were then sacrificed at 28 days after the final BrdU injection.
Animals were perfused transcardially with 0.1 M phosphate-buffered saline (PBS, pH 7.4) and fixed with fresh and cold 4% paraformaldehyde (PFA) in 0.1 M PBS. Brains were dissected and post-fixed overnight with 4% PFA in 0.1 M PBS at 4℃, washed for 6 h in PBS at 4℃, and cryoprotected overnight with 30% sucrose in 0.1 M PBS at 4℃. Brains were then embedded in freezing media (optimum cutting temperature), frozen in chilled isopentane (−25℃), and stored at −80℃ until sectioning. Brains were cryocut sagittally or coronally to sections of 40 µm in thickness using a cryostat (model CM3050 S; Leica Microsystems, Bannockburn). Sections were stored in cryoprotectant solution (25% ethylene glycol, 25% glycerol, and 0.05 M sodium phosphate buffer, Na-PB) at −20℃.
Sections were washed with Na-PB and mounted onto charged slides. Sections stained for BrdU were pretreated with 2 N HCl at 37℃ for 30 min and neutralized with PBS before incubation with primary antibody. Sections were blocked with PBS containing 5% horse serum and 0.4% Triton X-100 in PBS (PBST) for 60 min followed by incubation with primary antibody in the same buffer solution at 10℃ overnight. Primary antibodies were used at the following concentrations: rat anti-BrdU (1:200; Abcam, Cambridge, UK), mouse anti-GFAP (1:200; Chemicon, Temecula, CA, USA), mice anti-neuronal nuclear antigen (NeuN, 1:100; Chemicon), rabbit anti-ionized calcium-binding adapter molecule 1 (Iba-1, 1:100; Wako Chemicals USA, Inc., Richmond, VA, USA), and goat anti-Doublecortin (DCX, 1:100; Cell signaling, Danvers, MA, USA). Sections were washed three times with PBST for 10 min at room temperature and blocked in PBST containing 5% horse serum for 30 min. Samples were then incubated with secondary antibodies conjugated to FITC (green, Jackson Immuno-Research, West Grove, PA, USA) or CY3 (red, Jackson Immuno-Research) at room temperature for two h, washed three times with PBST, stained with 10 mg/ml 4′, 6′-diamidino-2-phenylindole (DAPI) (Sigma) for 10 min, and coverslipped.
To examine apoptosis of NSCs in the hippocampal SGZ, immunofluorescent staining was performed using activated caspase-3 antibody (1:200, Cell Signaling, Danvers, MA, USA) according to the immunofluorescent staining method described above. Activated caspase-3 positive cells were counted throughout the hippocampal SGZ of six serial sections.
Staining was analyzed with a confocal microscope (Zeiss, Thornwood, NY, USA) equipped with an argon/krypton laser (488 nm), two helium/neon lasers (543 and 633 nm), a Coherent (Santa Clara, CA, USA), and a Chameleon two-photon laser at 725 nm to image DAPI staining. Pictures were obtained with a Spot RT Slider camera and associated software (version 3.4; Diagnostic Instruments, Sterling Heights, MI, USA) or LSM software (Zeiss, Thornwood, NY, USA). Co-localizations were investigated by performing z-stack acquisitions and three-dimensional reconstructions. Images were analyzed with Image J (Ver.1.4, NIH, USA). Adobe Photoshop version 7.0.1 (Adobe Systems, San Jose, CA, USA) was used to adjust contrast and brightness.
A complete series of one in ten sections of the DG was analyzed. For each experiment, nine sections per mouse were selected for analysis. Immunolabeled cells were counted and multiplied by 10 to obtain the total number of labeled cells throughout the DG. Data are expressed as mean±SEM. Statistical analyses were performed using one-way analysis of variance (ANOVA) followed by Student-Newman-Keuls test for multiple comparisons. Results were considered statistically significant when p value was less than 0.05 [33].
To investigate whether TLR2 could regulate neurogenesis in the dentate gyrus after photothrombotic ischemia, WT and TLR2 KO mice were sacrificed at 10 days after ischemia and their brain sections were stained with DCX, an immature new born neural marker. The number of DCX positive cells was not significantly different between WT and TLR2 KO mice under normal condition. After ischemic injury, DCX positive cells were increased in WT (32.4±5.9% increase) and TLR2 (22.4±4.9% increase) groups compared to those under sham condition. However, DCX positive cells were significantly reduced in TLR2 KO mice compared to those in WT mice after ischemic injury (14±5.7% decrease, p=0.0387) (Figs. 1A and B), indicating that DCX-positive cells in SGZ were significantly increased by photothrombotic ischemia in both WT mice and TLR2 KO mice. However, the number of DCX-positive cells in TLR2 KO mice was decreased compared to that in WT mice after ischemia.
BrdU-labeled nuclei in SGZ of both control and ischemic brains appeared as irregularly shaped clusters located exclusively in the SGZ. This pattern is a characteristic of dentate gyrus progenitor cells. The number of BrdU-positive cells was not changed by sham operation or at 1 or 3 days after photothrombotic ischemia (Fig. 2A), demonstrating that the stress of surgery did not increase cell proliferation. The number of BrdU-positive cells was gradually increased in both TLR2 KO mice (113.7±6.9% compared to WT at 0 day) and WT (167.7±6.4% compared to TLR2 KO at 0 day) mice at 7 days after photothrombotic ischemia, with 2.5-fold increase in BrdU immunoreactive nuclei per square millimeter dentate gyrus compared to sham operating group (Fig. 2B). The increasing rate of BrdU-positive cells in TLR2 KO mice (58.5±7.3%) was decreased compared to that in WT mice. These results demonstrated that TLR2 stimulated the proliferation of adult NSCs after photothrombotic ischemia in the hippocampal DG in vivo.
To examine the survival and matured neuronal differentiation of BrdU-positive cells after ischemic injury, numbers of BrdU-positive cells and NeuN/BrdU or GFAP/BrdU double-positive cells were determined at 28 days after BrdU injection once daily for five consecutive days. NeuN was used as a marker of mature neuron while GFAP was used as a marker of glial cell. BrdU positive cells at 28 days after five days of BrdU injection were diminished in the hippocampal DG of TLR2 KO mice (8.8±3.0% decrease, p=0.0182) compared to those in WT mice (Figs. 3A and B). To investigate apoptosis of neural stem cells or newborn neuron in the hippocampal DG, activated caspase-3 (an apoptotic executing caspase) immunostaining was performed. Caspase 3-positive cells in SGZ were increased in TLR2 KO mice (9.6±2.9% increase, p=0.0165) compared to those in WT mice (Figs. 3C and D), indicating that new born neurons underwent apoptosis. The number of NeuN/BrdU double-positive cells in the hippocampal DG of TLR2 KO mice were less than those in WT mice (7.6±3.0% less compared to WT, p=0.0371, Fig. 4B). In addition, the percentage (%) of NeuN/BrdU double-positive cells over the number of BrdU positive cells in the hippocampal DG of TLR2 KO mice were less than those in WT mice (25.5±6.3% less compared to WT, p=0.0067, Fig. 4C). However, the number and percentage of GFAP/BrdU positive cells in TLR2 KO mice were higher (7.6±3.0% higher) than those in WT mice (Figs. 5A and B). These results indicated that TLR2 enhanced neuronal differentiation but inhibited astrocyte differentiation from stem cells of hippocampal dentate gyrus after ischemic injury, suggesting that TLR2 could modulate the fate of neural or glial differentiation from neural stem cells after ischemic injury.
Recent brain studies have shown that microglia express TLR2 and modulate hippocampal neurogenesis through secreting immune related cytokines and neurotrophic factors [34]. To determine the effect of TLR2 on ischemia-induced inflammation, the activity of microglia following ischemia was quantified by measuring immunoreactivity of Iba-1, a specific marker of microglia (n=5 each). The number of Iba-1-positive cells in DG was increased in TLR2 KO mice compared to that in WT mice at 7 days after photothrombotic ischemia (76.23±18.8% increase, p=0.0066, Fig. 6A and 6B). This result demonstrated that TLR2 could suppress the activity of microglia in the hippocampus after ischemia.
Toll was first discovered in Drosophila melanogaster. It regulates dorso-ventral patterning during embryonic development and controls immune responses [3536]. After the discovery of TLR4, a mammalian homolog of Toll, many mammalian homologs have been identified across diverse species. In mammalian, TLRs are involved in innate immune activity and activation of the adaptive immune system. They also have physiological and metabolic roles in adults. There was a report that Knock-out of TLR2 affects immune response through modulating proinflammatory cytokines in human coronary artery endothelial cells [37]. Human adipose-derived stem cells (hASCs) expressing all TLRs including TLR2 are activated by TLR ligands such as LPS, leading to significant upregulation of IL6 and IL8 that can stimulate osteoblast differentiation [38]. Mesenchymal stem cells derived from human turbinate tissue also express TLR2 and TLR4 while TLR agonist affects proliferation, differentiation, and immune responses [39]. TLR2 regulates multiple metabolism along with TLR4 to control autonomic nervous system [40].
Adult neurogenesis continues in the hippocampal DG and SVZ throughout adulthood for cognition or repair of lost neurons following injury or disease [4142]. Endogenous neurogenesis can be regulated by various stimuli, including physical exercise, enriched environment, stroke, and growth factors [4344]. Recent reports have shown that ischemic brain injury upregulates vascular endothelial growth factor (VEGF), fibroblast growth factor (FGF)-2, and glial derived neurotrophic factor (GDNF) expression, resulting in increased neurogenesis from adult dentate gyrus [454647]. In the present study, photochemical-induced cerebral ischemia also enhanced neurogenesis in hippocampal dentate gyrus in both WT mice and TLR2 KO mice. This suggests that cerebral ischemia is one of the major stimuli for neurogenesis from neural stem cells. TLR2 is known as a candidate receptor that may regulate adult neurogenesis from neural stem cells in SVZ and hippocampal SGZ [4849]. However, whether TLR2 regulates neurogenesis under cerebral ischemia condition in hippocampus has not been reported yet.
In general, adult neurogenesis can be divided into five separated stages: stem cells proliferation, survival, migration, differentiation, and synaptic integration. In the present study, the number of DCX (newborn neuronal marker)-positive cells was decreased in hippocampal DG TLR2 KO mice compared to that in WT mice after cerebral ischemia, indicating that TLR2 could promote neurogenesis under cerebral ischemic injury. To elucidate the mechanism underlying the stimulatory effect of TLR2 on adult neurogenesis from neural stem cells after cerebral ischemic injury, stem cells proliferation, cell survival, and differentiation were examined in the present study. The number of BrdU-positive cells was lower in hippocampal DG of TLR2 KO mice than that in WT mice after cerebral ischemic injury, demonstrating that TLR2 could promote proliferation of neural stem cell in hippocampal DG, resulting in increased neurogenesis in hippocampal DG after cerebral ischemia. Our analysis using TLR2 KO mice after brain specific damage demonstrated that TLR2 played an important role in brain development and self-renewal property of hippocampal neural stem cells. It has been recently reported that TLR2 activation upregulates cell cycle related molecular targets through modulating inflammatory mediators in stem cells [50]. However, such data are not consistent with a previous study showing that TLR2 is not involved in the regulation of neural stem cell proliferation in hippocampal DG without cerebral ischemia [48]. In addition, the rate of neural differentiation from neural stem cells was compared between hippocampal DG of TLR2 KO mice and WT mice after cerebral ischemia in the present study. The percentage of NeuN (mature neural marker)/BrdU-positive cells were found to be lower in hippocampal DG of TLR2 KO mice than that in WT mice after cerebral ischemia, whereas the percentage of GFAP (astrocyte marker)/BrdU-positive cell was higher in hippocampal DG of TLR2 KO mice compared to that in WT mice after cerebral ischemia. These results suggest that TLR2 can enhance neural differentiation from neural stem cells in DG after cerebral ischemia, resulting in increased neurogenesis after cerebral ischemia. This is consistent with results of a previous report showing that TLR2 increases neuronal differentiation in mouse embryonic neural stem cells [48].
To determine whether TLR2 receptor was associated with apoptosis or survival of newborn neuron, the number of activated caspase-3-positive cells was examined. In the present study, the number of BrdU-positive cells at 28 days after consecutive BrdU injection for five days was lower in the hippocampal DG of TLR2 KO mice compared to that in WT mice after cerebral ischemia. Besides, the number of caspase 3-positive cells was higher in the hippocampal DG of TLR2 KO mice compared to that in WT mice after cerebral ischemia. This result supports that TLR2 has a protective effect on the survival of adult NSCs at a late step which is reduced by ischemia. It is consistent with a previous report demonstrating that TLR-mediated NF-κB activation seems to have anti-apoptotic role in neuron under ischemic injury or oxidative stress condition [515253]. However, TLR2 can regulate anti-apoptotic or cytotoxic function in various cell types through NF-κB signaling pathway [5455]. In the present study, the recruitment of activated microglia was evaluated under ischemic condition. Activated microglia was found to be significantly increased in TLR2 KO mice compared to that in WT mice, meaning that TLR2 decreased microglia activation. Taken together, our findings suggest that TLR2 can augment cell survival of newborn neural cells in the hippocampal DG through modulating microglia activity after cerebral ischemia.
TLR2 is known to be expressed in all tissues, including adult neural stem cells, microglia, and neurons [565758]. However, the present study did not study tissue specific effect of TLR2 because whole body knock-out mice were used in this study. It has been reported that Cre recombinase under control of seleted tissue promoter such as nestin or Sox2 promoter can allow knock-out or overexpression of a floxed target gene [59]. Therefore, further study is needed to determine tissue specific effects of TLR2 through knockout or overexpression of TLR2 using Cre system in neural stem cells of tissue specific transgenic mice. Specific system such as inactivation of TLR2 in specific tissues by Cre recombinase might be used to regulate proliferation, differentiation, and survival of neural stem cells in hippocampal neurogenesis.
In summary, TLR2 receptor can enhance adult neurogenesis from neural stem cells in the hippocampal DG after cerebral ischemia through promoting proliferation and neural differentiation of neural stem cells and survival of newborn neurons. Therefore, TLR2 pathway that regulates hippocampal neurogenesis after ischemia might be targeted as a prospective therapeutic strategy to treat brain nerve injury and various neurodegenerative disorders.
ACKNOWLEDGEMENTS
This work was supported by grants (2011-0030121, 2015R1A2A 2A01006595) of the National Research Foundation (NRF) funded by the Ministry of Science, ICT and Future Planning (MSIP), Republic of Korea. It was also supported by a grant (CRI 17033-22) from Chonnam National University Hospital Biomedical Research Institute, Republic of Korea.
Notes
References
1. Kempermann G, Song H, Gage FH. Neurogenesis in the adult hippocampus. Cold Spring Harb Perspect Biol. 2015; 7:a018812. PMID: 26330519.


2. Bond AM, Ming GL, Song H. Adult mammalian neural stem cells and neurogenesis: five decades later. Cell Stem Cell. 2015; 17:385–395. PMID: 26431181.


3. Amrein I. Adult hippocampal neurogenesis in natural populations of mammals. Cold Spring Harb Perspect Biol. 2015; 7.


4. Morrens J, Van Den Broeck W, Kempermann G. Glial cells in adult neurogenesis. Glia. 2012; 60:159–174. PMID: 22076934.


5. Drew LJ, Fusi S, Hen R. Adult neurogenesis in the mammalian hippocampus: why the dentate gyrus? Learn Mem. 2013; 20:710–729. PMID: 24255101.


6. Ming GL, Song H. Adult neurogenesis in the mammalian brain: significant answers and significant questions. Neuron. 2011; 70:687–702. PMID: 21609825.


7. Suh H, Deng W, Gage FH. Signaling in adult neurogenesis. Annu Rev Cell Dev Biol. 2009; 25:253–275. PMID: 19575663.


8. Abrous DN, Koehl M, Le Moal M. Adult neurogenesis: from precursors to network and physiology. Physiol Rev. 2005; 85:523–569. PMID: 15788705.


9. Garthe A, Kempermann G. An old test for new neurons: refining the Morris water maze to study the functional relevance of adult hippocampal neurogenesis. Front Neurosci. 2013; 7:63. PMID: 23653589.


10. Rao MS, Hattiangady B, Shetty AK. Status epilepticus during old age is not associated with enhanced hippocampal neurogenesis. Hippocampus. 2008; 18:931–944. PMID: 18493929.


11. Liu J, Solway K, Messing RO, Sharp FR. Increased neurogenesis in the dentate gyrus after transient global ischemia in gerbils. J Neurosci. 1998; 18:7768–7778. PMID: 9742147.


12. Lathia JD, Okun E, Tang SC, Griffioen K, Cheng A, Mughal MR, Laryea G, Selvaraj PK, ffrench-Constant C, Magnus T, Arumugam TV, Mattson MP. Toll-like receptor 3 is a negative regulator of embryonic neural progenitor cell proliferation. J Neurosci. 2008; 28:13978–13984. PMID: 19091986.


13. Mouihate A. TLR4-mediated brain inflammation halts neurogenesis: impact of hormonal replacement therapy. Front Cell Neurosci. 2014; 8:146. PMID: 24904290.


14. Barak B, Feldman N, Okun E. Toll-like receptors as developmental tools that regulate neurogenesis during development: an update. Front Neurosci. 2014; 8:272. PMID: 25221470.


15. Ma Y, Haynes RL, Sidman RL, Vartanian T. TLR8: an innate immune receptor in brain, neurons and axons. Cell Cycle. 2007; 6:2859–2868. PMID: 18000403.


16. Matsuda T, Murao N, Katano Y, Juliandi B, Kohyama J, Akira S, Kawai T, Nakashima K. TLR9 signalling in microglia attenuates seizure-induced aberrant neurogenesis in the adult hippocampus. Nat Commun. 2015; 6:6514. PMID: 25751136.


17. Kaul D, Habbel P, Derkow K, Krüger C, Franzoni E, Wulczyn FG, Bereswill S, Nitsch R, Schott E, Veh R, Naumann T, Lehnardt S. Expression of Toll-like receptors in the developing brain. PLoS One. 2012; 7:e37767. PMID: 22666391.


18. Hemmati F, Ghasemi R, Mohamed Ibrahim N, Dargahi L, Mohamed Z, Raymond AA, Ahmadiani A. Crosstalk between insulin and Toll-like receptor signaling pathways in the central nervous system. Mol Neurobiol. 2014; 50:797–810. PMID: 24464263.


19. Brunn A, Mihelcic M, Carstov M, Feind L, Wieser EC, Schmidt J, Utermöhlen O, Deckert M. Toll-like receptor 2, Toll-like receptor 4, myeloid differentiation response gene 88, and Toll-IL-1 receptor domain-containing adaptor-inducing interferon-γ (TRIF) selectively regulate susceptibility of P0106-125-induced murine experimental autoimmune neuritis. Am J Pathol. 2017; 187:42–54. PMID: 27842213.


20. Drouin-Ouellet J, St-Amour I, Saint-Pierre M, Lamontagne-Proulx J, Kriz J, Barker RA, Cicchetti F. Toll-like receptor expression in the blood and brain of patients and a mouse model of Parkinson's disease. Int J Neuropsychopharmacol. 2014; 18.


21. Humann J, Mann B, Gao G, Moresco P, Ramahi J, Loh LN, Farr A, Hu Y, Durick-Eder K, Fillon SA, Smeyne RJ, Tuomanen EI. Bacterial peptidoglycan traverses the placenta to induce fetal neuroproliferation and aberrant postnatal behavior. Cell Host Microbe. 2016; 19:901.


22. Dzamko N, Gysbers A, Perera G, Bahar A, Shankar A, Gao J, Fu Y, Halliday GM. Toll-like receptor 2 is increased in neurons in Parkinson's disease brain and may contribute to alpha-synuclein pathology. Acta Neuropathol. 2017; 133:303–319. PMID: 27888296.


23. Derkow K, Krüger C, Dembny P, Lehnardt S. Microglia induce neurotoxic IL-17+ γδ T cells dependent on TLR2, TLR4, and TLR9 activation. PLoS One. 2015; 10:e0135898. PMID: 26288016.


24. Liu Y, Yin H, Zhao M, Lu Q. TLR2 and TLR4 in autoimmune diseases: a comprehensive review. Clin Rev Allergy Immunol. 2014; 47:136–147. PMID: 24352680.


25. Yonekura I, Kawahara N, Nakatomi H, Furuya K, Kirino T. A model of global cerebral ischemia in C57 BL/6 mice. J Cereb Blood Flow Metab. 2004; 24:151–158. PMID: 14747741.


26. Ahn JH, Shin BN, Park JH, Kim IH, Cho JH, Chen B, Lee TK, Tae HJ, Lee JC, Cho JH, Kang IJ, Kim YM, Lee YL, Won MH, Seo JY. Long-term observation of neuronal degeneration and microgliosis in the gerbil dentate gyrus after transient cerebral ischemia. J Neurol Sci. 2016; 363:21–26. PMID: 27000214.


27. Uemori T, Toda K, Seki T. Seizure severity-dependent selective vulnerability of the granule cell layer and aberrant neurogenesis in the rat hippocampus. Hippocampus. 2017; 27:1054–1068. PMID: 28608989.


28. Yang J, Zhang X, Chen X, Wang L, Yang G. Exosome mediated delivery of miR-124 promotes neurogenesis after ischemia. Mol Ther Nucleic Acids. 2017; 7:278–287. PMID: 28624203.


29. Cao CX, Yang QW, Lv FL, Cui J, Fu HB, Wang JZ. Reduced cerebral ischemia-reperfusion injury in Toll-like receptor 4 deficient mice. Biochem Biophys Res Commun. 2007; 353:509–514. PMID: 17188246.


30. Ziegler G, Harhausen D, Schepers C, Hoffmann O, Röhr C, Prinz V, König J, Lehrach H, Nietfeld W, Trendelenburg G. TLR2 has a detrimental role in mouse transient focal cerebral ischemia. Biochem Biophys Res Commun. 2007; 359:574–579. PMID: 17548055.


31. Seong KJ, Lee HG, Kook MS, Ko HM, Jung JY, Kim WJ. Epigallocatechin-3-gallate rescues LPS-impaired adult hippocampal neurogenesis through suppressing the TLR4-NF-κB signaling pathway in mice. Korean J Physiol Pharmacol. 2016; 20:41–51. PMID: 26807022.


32. Watson BD, Dietrich WD, Busto R, Wachtel MS, Ginsberg MD. Induction of reproducible brain infarction by photochemically initiated thrombosis. Ann Neurol. 1985; 17:497–504. PMID: 4004172.


33. Seong KJ, Lee HG, Kook MS, Ko HM, Jung JY, Kim WJ. Epigallocatechin-3-gallate rescues LPS-impaired adult hippocampal neurogenesis through suppressing the TLR4-NF-κB signaling pathway in mice. Korean J Physiol Pharmacol. 2016; 20:41–51. PMID: 26807022.


34. Gemma C, Bachstetter AD. The role of microglia in adult hippocampal neurogenesis. Front Cell Neurosci. 2013; 7:229. PMID: 24319411.


35. Valanne S, Wang JH, Rämet M. The drosophila toll signaling pathway. J Immunol. 2011; 186:649–656. PMID: 21209287.
36. Quintin J, Asmar J, Matskevich AA, Lafarge MC, Ferrandon D. The Drosophila Toll pathway controls but does not clear Candida glabrata infections. J Immunol. 2013; 190:2818–2827. PMID: 23401590.
37. Wang Y, Chen L, Tian Z, Shen X, Wang X, Wu H, Wang Y, Zou J, Liang J. CRISPR-Cas9 mediated gene knockout in human coronary artery endothelial cells reveals a pro-inflammatory role of TLR2. Cell Biol Int. 2018; 42:187–193. PMID: 28986953.


38. Lombardo E, DelaRosa O, Mancheño-Corvo P, Menta R, Ramírez C, Büscher D. Toll-like receptor-mediated signaling in human adiposederived stem cells: implications for immunogenicity and immunosuppressive potential. Tissue Eng Part A. 2009; 15:1579–1589. PMID: 19061425.


39. Hwang SH, Cho HK, Park SH, Lee W, Lee HJ, Lee DC, Oh JH, Park SH, Kim TG, Sohn HJ, Kang JM, Kim SW. Toll like receptor 3 & 4 responses of human turbinate derived mesenchymal stem cells: stimulation by double stranded RNA and lipopolysaccharide. PLoS One. 2014; 9:e101558. PMID: 25004159.
40. Okun E, Griffioen KJ, Son TG, Lee JH, Roberts NJ, Mughal MR, Hutchison E, Cheng A, Arumugam TV, Lathia JD, van Praag H, Mattson MP. TLR2 activation inhibits embryonic neural progenitor cell proliferation. J Neurochem. 2010; 114:462–474. PMID: 20456021.


41. Parent JM, Yu TW, Leibowitz RT, Geschwind DH, Sloviter RS, Lowenstein DH. Dentate granule cell neurogenesis is increased by seizures and contributes to aberrant network reorganization in the adult rat hippocampus. J Neurosci. 1997; 17:3727–3738. PMID: 9133393.


42. Cameron HA, McKay R. Stem cells and neurogenesis in the adult brain. Curr Opin Neurobiol. 1998; 8:677–680. PMID: 9811628.
43. Emsley JG, Mitchell BD, Kempermann G, Macklis JD. Adult neurogenesis and repair of the adult CNS with neural progenitors, precursors, and stem cells. Prog Neurobiol. 2005; 75:321–341. PMID: 15913880.


44. Taupin P. Neurogenesis in the adult central nervous system. C R Biol. 2006; 329:465–475. PMID: 16797452.


45. Nakatomi H, Kuriu T, Okabe S, Yamamoto S, Hatano O, Kawahara N, Tamura A, Kirino T, Nakafuku M. Regeneration of hippocampal pyramidal neurons after ischemic brain injury by recruitment of endogenous neural progenitors. Cell. 2002; 110:429–441. PMID: 12202033.


46. Schneider A, Krüger C, Steigleder T, Weber D, Pitzer C, Laage R, Aronowski J, Maurer MH, Gassler N, Mier W, Hasselblatt M, Kollmar R, Schwab S, Sommer C, Bach A, Kuhn HG, Schäbitz WR. The hematopoietic factor G-CSF is a neuronal ligand that counteracts programmed cell death and drives neurogenesis. J Clin Invest. 2005; 115:2083–2098. PMID: 16007267.


47. Kobayashi T, Ahlenius H, Thored P, Kobayashi R, Kokaia Z, Lindvall O. Intracerebral infusion of glial cell line-derived neurotrophic factor promotes striatal neurogenesis after stroke in adult rats. Stroke. 2006; 37:2361–2367. PMID: 16873711.


48. Rolls A, Shechter R, London A, Ziv Y, Ronen A, Levy R, Schwartz M. Toll-like receptors modulate adult hippocampal neurogenesis. Nat Cell Biol. 2007; 9:1081–1088. PMID: 17704767.


49. Okun E, Griffioen KJ, Lathia JD, Tang SC, Mattson MP, Arumugam TV. Toll-like receptors in neurodegeneration. Brain Res Rev. 2009; 59:278–292. PMID: 18822314.


50. Nagai Y, Garrett KP, Ohta S, Bahrun U, Kouro T, Akira S, Takatsu K, Kincade PW. Toll-like receptors on hematopoietic progenitor cells stimulate innate immune system replenishment. Immunity. 2006; 24:801–812. PMID: 16782035.


51. Sarnico I, Lanzillotta A, Benarese M, Alghisi M, Baiguera C, Battistin L, Spano P, Pizzi M. NF-kappaB dimers in the regulation of neuronal survival. Int Rev Neurobiol. 2009; 85:351–362. PMID: 19607980.
52. Chen C, Edelstein LC, Gélinas C. The Rel/NF-kappaB family directly activates expression of the apoptosis inhibitor Bcl-x(L). Mol Cell Biol. 2000; 20:2687–2695. PMID: 10733571.
53. Aliprantis AO, Yang RB, Mark MR, Suggett S, Devaux B, Radolf JD, Klimpel GR, Godowski P, Zychlinsky A. Cell activation and apoptosis by bacterial lipoproteins through toll-like receptor-2. Science. 1999; 285:736–739. PMID: 10426996.


54. Frantz S, Kelly RA, Bourcier T. Role of TLR-2 in the activation of nuclear factor kappaB by oxidative stress in cardiac myocytes. J Biol Chem. 2001; 276:5197–5203. PMID: 11083876.
55. Conti L, Lanzardo S, Arigoni M, Antonazzo R, Radaelli E, Cantarella D, Calogero RA, Cavallo F. The noninflammatory role of high mobility group box 1/Toll-like receptor 2 axis in the self-renewal of mammary cancer stem cells. FASEB J. 2013; 27:4731–4744. PMID: 23970797.


56. Song H, Stevens CF, Gage FH. Astroglia induce neurogenesis from adult neural stem cells. Nature. 2002; 417:39–44. PMID: 11986659.


57. Ziv Y, Ron N, Butovsky O, Landa G, Sudai E, Greenberg N, Cohen H, Kipnis J, Schwartz M. Immune cells contribute to the maintenance of neurogenesis and spatial learning abilities in adulthood. Nat Neurosci. 2006; 9:268–275. PMID: 16415867.


58. Monje ML, Toda H, Palmer TD. Inflammatory blockade restores adult hippocampal neurogenesis. Science. 2003; 302:1760–1765. PMID: 14615545.


59. Lewandoski M. Conditional control of gene expression in the mouse. Nat Rev Genet. 2001; 2:743–755. PMID: 11584291.


Fig. 1
Role of TLR2 in differentiation of neural stem cells in hippocampal dentate gyrus (HDG) after photothrombotic ischemia.
(A) Representative confocal image of Doublecortin (DCX) positive cells in hippocampal dentate gyrus (DG) of wild type and TLR2 KO mice at 0 and 10 days after ischemic injury. DCX (Green) stained cells show newly generated immature neurons. (B) The number of DCX positive cell in hippocampal DG was lower in TLR2 KO mice than that in WT mice after photothrombosis induced cerebral ischemia. Quantitative analysis of the number DCX-positive cells (n=5 each). Data are expressed as mean±SEM. *p<0.05; ***p<0.001. Scale bar, 100 µm.
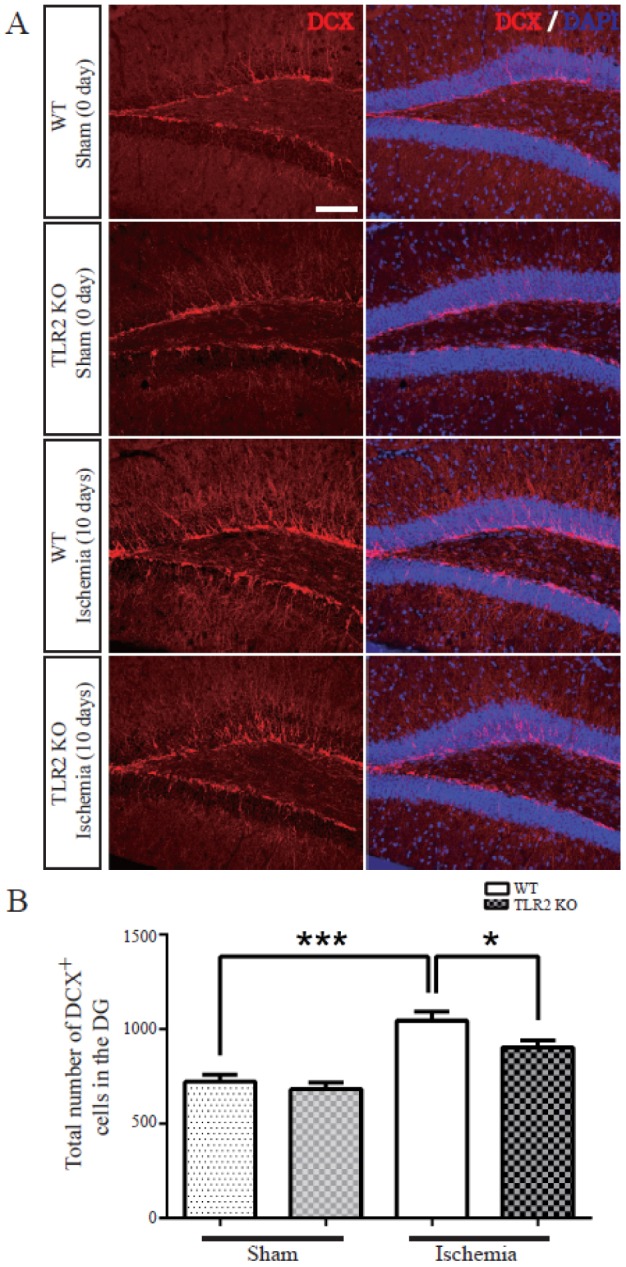
Fig. 2
Role of TLR2 in proliferation of neural stem cells in HDG after photothrombotic ischemia.
WT and TLR2 KO mice received a single dose of BrdU (50 mg/kg) at three hours before sacrifice. The number of BrdU-positive cells (green) in the SGZ of WT mice and TLR2 KO mice from 1 day to 10 days after ischemia was determined. (A) Newly proliferated cells in the subgranular zone (SGZ) of the DG were immunostained with anti-BrdU. (B) Quantitative analysis of the number of BrdU-positive cells in the DG of the hippocampus. The number of BrdU-positive cells was gradually increased in TLR2 KO mice and WT mice after photothrombotic ischemia. However, the increasing rate of BrdU-positive cells was lower in TLR2 KO mice than that in WT mice. Data are expressed as mean±SEM. **p<0.01; ***p<0.001. Scale bar, 100 µm.

Fig. 3
Role of TLR2 in the survival of new generated cells in HDG after photothrombotic ischemia.
(A) BrdU-positive cells (green) in the hippocampal dentate gyrus (DG) were determined at three hours and 28 days after BrdU injection for five consecutive days after ischemia. (B) Survival rate of BrdU-positive cells was lower in TLR2 KO mice than that in WT mice. (C) Representative confocal image of activated caspase-3 positive cells (red) in the subgranular zone (SGZ) from six sections after ischemia. (D) The number of caspase-3 positive cells was increased in TLR2 KO mice than that in WT mice after photothrombosis induced cerebral ischemia. Data are expressed as mean±SEM. *p<0.05. Scale bar, 200 µm.
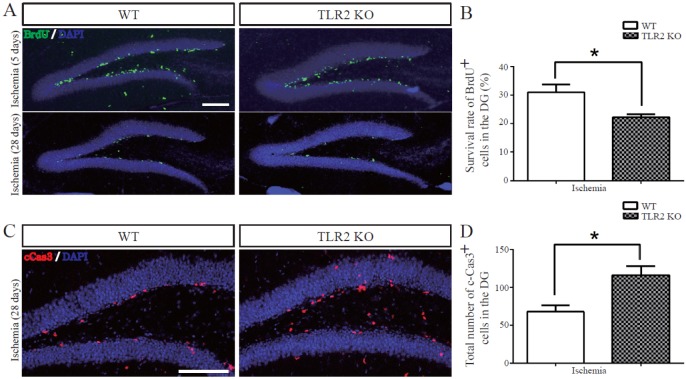
Fig. 4
Role of TLR2 in mature neuronal differentiation of newly generated cells in the HDG after photothrombotic ischemia.
(A) Representative microphotograph of BrdU/NeuN double positive cells in the hippocampal dentate gyrus at 28 days after BrdU injection for five consecutive days. BrdU (green) and NeuN (red) double stained cells (yellow, arrow) represent newly generated mature neurons. (B) Quantitative analysis of the number of BrdU- and/or NeuN-positive cells. (C) The percentage of NeuN/BrdU double positive cells was lower in TLR2 KO mice than that in WT mice. Data are presented as mean±SEM. *p<0.05; **p<0.01. Scale bar, 100 µm.

Fig. 5
Role of TLR2 in glia differentiation from newly generated cells in HDG after photothrombotic ischemia.
(A) Representative confocal image of BrdU/GFAP double-positive cells in subgranular zone (SGZ) of 28 days after BrdU injection. BrdU (green) and GFAP (red) double stained cells (yellow) represent newly generated astrocytes. (B) The percentage of BrdU/GFAP positive cells ratio was higher in TLR2 KO mice than that in WT mice after photothrombosis-induced cerebral ischemia. Data are presented as mean±SEM. *p<0.05. Scale bar, 100 µm.

Fig. 6
Role of TLR2 in microglia activation in the hippocampus after photothrombotic ischemia.
(A) Representative confocal image of Iba-1 (red) positive cells in the DG at 7 days after photothrombotic ischemia. (B) Quantification of the number of Iba-1-positive cells. It was significantly increased in TLR2 KO mice compared to that in WT mice. Data are presented as mean±SEM. **p<0.01. Scale bar, 100 µm.
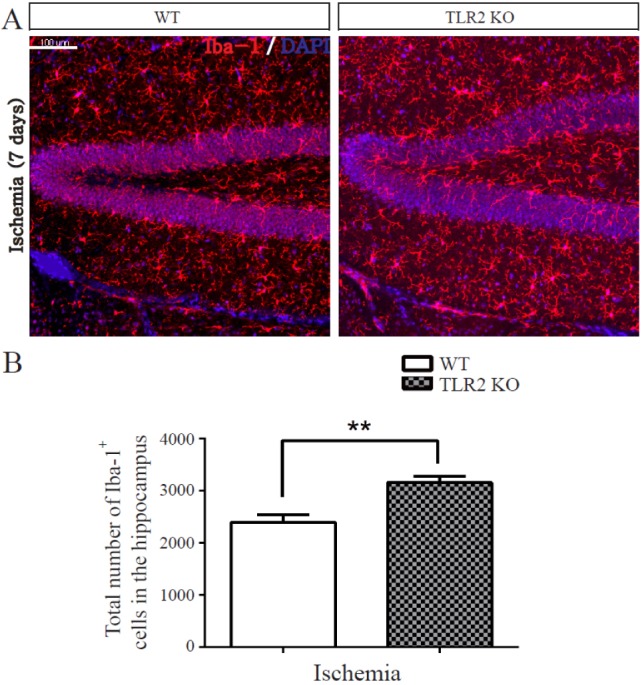