Abstract
Myofibrillogenesis regulator-1 (MR-1) is a novel protein involved in cellular proliferation, migration, inflammatory reaction and signal transduction. However, little information is available on the relationship between MR-1 expression and the progression of atherosclerosis. Here we report atheroprotective effects of silencing MR-1 in a model of Ang II-accelerated atherosclerosis, characterized by suppression focal adhesion kinase (FAK) and nuclear factor kappaB (NF-κB) signaling pathway, and atherosclerotic lesion macrophage content. In this model, administration of the siRNA-MR-1 substantially attenuated Ang II-accelerated atherosclerosis with stabilization of atherosclerotic plaques and inhibited FAK, Akt, mammalian target of rapamycin (mTOR) and NF-kB activation, which was associated with suppression of inflammatory factor and atherogenic gene expression in the artery. In vitro studies demonstrated similar changes in Ang II-treated vascular smooth muscle cells (VSMCs) and macrophages: siRNA-MR-1 inhibited the expression levels of proinflammatory factor. These studies uncover crucial proinflammatory mechanisms of Ang II and highlight actions of silencing MR-1 to inhibit Ang II signaling, which is atheroprotective.
There is considerable evidence implicating a role for angiotensin II (Ang II) in the atherogenic process. Ang II regulates not only proteolytic enzymes secretion within atherosclerotic plaques but also inflammatory factor secretion within the arterial wall. Furthermore, among the different client proteins modified by Ang II, focal adhesion kinase (FAK), protein kinase B (Akt), mammalian target of rapamycin (mTOR) and nuclear factor kappaB (NF-κB) signaling pathways play a critical role in mediating proliferation, migration and inflammation of cells [123]. In the same time, previous studies have been demonstrated that modulation of FAK, Akt, mTOR and NF-κB signaling pathway decreases inflammatory processes and stabilize artery atheromatous plaque in the apolipoprotein E-deficient (ApoE−/−) model of atherosclerosis [4567]. However, the precise molecular mechanisms that underlie atherosclerosis-related inflammation remain to be fully elucidated.
Myofibrillogenesis regulator-1 (MR-1) was identified from a human skeletal muscle cDNA library, which encodes a protein of 142 amino acids [8]. Our previous researches showed that silencing MR-1 protected the development of cardiac hypertrophy, myocardial inflammatory injury and inhibited mTOR signaling pathway and the expression of matrix metalloprotein (MMP) 2 induced by Ang II [91011]. Ren et al. found that MR-1 modulates proliferation and migration of cells through FAK/Akt signaling pathway [12] and Li found that overexpression of MR-1 activated NF-κB activation and the proinflammatory factor expression of monocyte chemoattractant protein-1 (MCP-1) and tumor necrosis factor (TNF)-α induced by Ang II [13]. However, whether silencing MR-1 would attenuate Ang II-induced vascular inflammation and atherosclerosis in ApoE−/−mice has not been explored. Based on important role of MR-1 in cell proliferation, migration, inflammatory reaction, and signal transduction regulation, we surmised that MR-1 plays an important role in atherosclerosis development induced by Ang II.
The present study shows significant MR-1 upregulation in the atherosclerotic lesions of ApoE−/− mice induced by Ang II. Silencing MR-1 exhibited decreased atheromatous lesion formation secondary to inactivation of FAK, Akt, mTOR, NF-κB signaling pathway and alleviation of inflammation.
Eight week-old male ApoE−/− mice were obtained from Peking University Health Science Center (purchased from Jackson Laboratory) and fed with regular rodent chow. All of the animal experiments followed the instructions of the Laboratory Animal Management Statute of china Physiological Society. After 1 week of accommodation, mice were randomly divided into the following three groups (n=18 in each group): 1) untreated group served as controls; 2) siRNA-MR-1 injected group received Ang II (Sigma, 2.5 µg/kg/min, dissolved in PBS including 0.01 M acetic acid) by implanted subcutaneous minipump (model 2004; Alza Corp., Mountain View, CA) for 4 weeks; 3) siRNA-control injected group received Ang II (Sigma, 2.5 µg/kg/min, dissolved in PBS including 0.01 M acetic acid) infusing by implanted subcutaneous minipump for 4 weeks. All operation was proceeded under anesthesia with 2.5% avertin (14 µl/g body weight, i.p., Aldrich Chemical Co.). The doses of Ang II and siRNA-MR-1, siRNAcontrol were chosen to mimic previous reports [1014]. Jugular vein injecting were performed during avertin anesthesia in the next day of operation as described previously [3]. Two weeks later, a second injection was administered in a similar fashion. Animals were sacrificed after 4 weeks.
After 4 weeks, mice were killed and perfused with 7.5% sucrose in paraformaldehyde. Afterwards, a section of the thoracic aorta fixed with 4% paraformaldehyde for 12 h (n=6). Then embedded in paraffin and cut into 5 µm serial sections which were prepared every 100 µm. Eight sections were prepared from each animal subject for hematoxylin and eosin (H&E) staining. For the detection of collagen, the sections were stained with picrosirius red. The plaque area and collagen area were observed and calculated using Image-Pro Plus 6.0 software (Media Cybernetics Inc., Rockville, MD, USA). Images were captured with a Sony 3-CCD video camera and analyzed by ImagePro image analysis software. Aortic immunocytochemical analyses were performed with antibodies specific for CD68 and for colocalization studies in mice plaques, MR-1 expressing cells (macrophages and VSMC) were identified by immunofluorescence (CD-68 and α-actin Abs, respectively; Dako) in combination with MR-1 immunoperoxidase (MR-1 polyclonal antibody was generated in our laboratory) [10].
Systolic blood pressure was measured by tail-cuff plethysmography (BP-2000 system; Visitech Systems, Apex, NC) as described previously [13]. Mice were fasted for 12–15 h before blood was collected at the time of killing. Serum was separated by centrifugation and stored at −80℃. Total cholesterol, triglycerides, and free fatty acids were analyzed by using enzymatic methods (Wako Chemicals). Blood glucose was measured by a one-touch glucose monitoring system.
Primary macrophages were isolated from ApoE−/−mice peritoneal cavity 5 days after thioglycollate injection. Cells were seeded in RPMI medium 1640 supplemented with 10% FBS (Invitrogen) for 4 h, then rinsed and then maintained in DMEM supplemented with 2 mM L-glutamine, 100 U/ml penicillin, 100 mg/ml streptomycin (Invitrogen), and 10% FBS for 24~48 h. Primary murine VSMC cultures were obtained from ApoE−/− mice aortas and identified by examining the SMC-specific marker alphasmooth muscle (alpha-SM) actin by flow cytometry, and then cultured in DMEM supplemented with 2 mM L-glutamine, 100 U/ml penicillin, 100 mg/ml streptomycin (Invitrogen), and 10% FBS. In the experiments on mTOR signaling, the mTOR inhibitor rapamycin (Sigma) was added to the treatment cells for 24 h. Inhibitors were dissolved in DMSO (Sigma), and the final concentration of DMSO in the treatment medium was 0.1% (vol/vol). Rapamycin was used at the concentrations of 100 nM. The cells cultured without inhibitors contained an equivalent amount of DMSO. After 24 h, the cells were treated with Ang II (100 nM) for 8 h, then the protein expression levels of MR-1, TNF-α, MMP-2, MCP-1, p65 and p-p65 were determined by Western blotting.
After 4 weeks, mice were killed and the aortas were removed for Western blotting analysis. Proteins were extracted from aortic tissues or cells as previously described [14]. Proteins (50 µg) were subjected to SDS-PAGE, transferred to polyvinylidene difluoride membranes (Amersham Pharmacia Biotech, Uppsala, Sweden), and probed with monoclonal antibodies against p-FAK, p-Akt, p-p65, TNF-α, MCP-1, MMP-2, p65, GAPDH and MR-1 (polyclonal antibody was generated in our laboratory) [10] (1:5000, Santa Cruz) overnight at 4℃ followed by anti-mouse HRP-conjugated antibodies (1:2000, Santa Cruz) incubation. Protein bands were visualized using Chemiluminescence kit (Amersham). Each sample was analyzed in triplicate and normalized to values for GAPDH protein expression.
Homogenized aortic samples (50~100 mg) or confluent cells in a 100 mm dish were lysed in 1 ml TRIzol Reagent (Invitrogen).
RNA was separated from DNA by phenol-chloroform phase separation and precipitated with isopropyl alcohol and washed with 75% ethanol. The RNA pellet was dried and resuspended in RNase-free water. RNA samples then reverse-transcribed into cDNA by using a TaqMan Reverse Transcription Reagent kit (Applied Bio systems) and a TaqMan PCR Core Reagent Kit (Applied Bio systems) with gene-specific sense and antisense primers (Table 1). Each sample was analyzed in triplicate and normalized to values for GAPDH mRNA expression by using TaqMan GAPDH Control Reagent.
To explore the potential role of MR-1 in the development of atherosclerosis, we first examined the MR-1 expression levels in mouse atherosclerotic plaques. Quantitative RT-PCR and Western blot revealed a strong increase of MR-1 expression in Ang II+siRNA-control treated mice compared with Ang II+siRNA-MR-1 treated mice (Figs. 1A and B). In the same time, Western blot showed that the MR-1 expression levels is induced by Ang II in VSMCs and macrophages compared with control cells (Fig.1C). Furthermore, colocalization studies identified VSMCs and macrophages as the main cell types expressing MR-1 in atherosclerosis lesions (Fig. 1D).
In order to further investigate the effects of treatment with siRNA-MR-1 on atherosclerosis, we determined the atherosclerotic lesion areas by H&E staining. Compared with the siRNA-control group or control group, the mice which were transfected with siRNA-MR-1 exhibited a significant suppression of atheroma formation in aortas induced by Ang II (Fig. 2A), suggesting that the knockdown of MR-1 suppressed atherosclerotic development in the ApoE−/− mice induced by Ang II.
In order to assess whether the silencing of MR-1 affects plaque stability, we analyzed fibrous cap and cap-to-core ratio by H&E staining. The fibrous cap in Ang II+siRNA-MR-1 mice (12.13±2.11 µm) showed a marked increase (p<0.05), compared with Ang II+siRNA-control mice (7.22±0.12 µm) (Fig. 2B). The cap-to-core ratio was also increased in Ang II+siRNA-MR-1 mice (0.23±0.03, p<0.05), compared to the Ang II+siRNA-control mice (0.16±0.01) (Fig. 2C).
Moreover, we analyzed the collagen content in atherosclerotic plaque using picrosirius red staining. We found that siRNA-MR-1 significantly increased the collagen area (Fig. 2D).
Moreover, Ang II strongly induced macrophage recruitment and foam cell formation in the vasculature. At 4 weeks, quantitative RT-PCR (qRT-PCR) analyses of aortas demonstrated that Ang II infusion increased expression of two macrophage marker genes, CD68 and F4/80 (4.1- and 7.6-fold, respectively) (Fig. 2E), and that siRNA-MR-1 markedly attenuated vascular CD68 (32%) and F4/80 (38%) gene expression.
To determine the effect of siRNA-MR-1 on atherosclerosis formation induced by Ang II, we treated ApoE−/− mice with Ang II (2.5 µg/kg/min) via an osmotic infusion mini-pump for 4 weeks. We found that siRNA-MR-1 administration had no effect on body weight and blood pressure vs. Ang II+siRNA-control (Table 2). The profiles of lipids and glucose either before or after Ang II+siRNA-control administration were not significantly different between Ang II+siRNA-control infused mice and control mice. Whereas administration of Ang II+siRNA-MR-1 significantly decreased serum FFA, triglycerides, total cholesterol and blood glucose concentrations by 17.8%, 19.2%, 19.6%, 22.2% respectively vs. Ang II+siRNA-control–infused mice (Table 2, *p<0.05).
We next used aortic tissues to measure the effect of silencing MR-1 on Ang II-induced expression of vascular inflammatory gene, such as the inflammatory marker TNF-α, MCP-1 and matrix metalloproteinase MMP-2 which is distributed throughout the vulnerable regions of atherosclerotic plaque and contributes to the development of atherosclerosis in ApoE−/− mice [15]. Western blotting showed increased expression of TNF-α, MCP-1 and MMP-2 in Ang II-infused+siRNA-control mice, whereas the siRNA-MR-1 could attenuate this effect of Ang II (Fig. 3A). Similarly, as shown in Fig. 3B, Ang II markedly increased the expression of VSMCs proatherogenic genes, including TNF-α and MCP-1, however, whose expression was attenuated by siRNA-MR-1 treatment and the similar result is shown in macrophages (data not shown).
To understand the potential mechanisms observed in siRNA-MR-1 treated mice, we studied the effect of siRNA-MR-1 on the activation of FAK-Akt-mTOR-NF-κB signaling pathway induced by Ang II, which is associated with VSMC proliferation and inflammation [16]. Our previous study found that siRNA-MR-1 can inhibit cell inflammation, proliferation and adhesion through FAK/Akt signaling pathway [1012], and Akt plays a central role in resistance of cells to apoptosis by regulating the activation of mTOR and NF-κB. Now, we investigated the effect of siRNA-MR-1 on these signaling proteins in artery induced by Ang II. As shown in Fig. 4A, siRNA-MR-1 decreased the levels of p-FAK, p-Akt, p-mTOR and p-p65 in artery wall induced by Ang II compared with the siRNA-control group.
In order to determine whether mTOR could interfere with NF-κB signaling pathway in our experimental conditions, we studied the effect of mTOR on the translocation of p65 to the nucleus which is important to NF-κB activation. As shown in Fig. 4B, Ang II induced the phosphorylation of p65 and nuclear translocation determined by Western blot analysis after 8 h of Ang II treatment of cells. More importantly, p65 phosphorylation and nuclear translocation were decreased in rapamycin treated cells. But, the phosphorylation of Akt was not affected by rapamycin (Fig. 4C). Lamin B1 was used as a loading control for nuclear extracts.
The current study demonstrates that systemic delivery of siRNA-MR-1 inhibits atherosclerotic lesion formation, macrophage accumulation, and maintaining atherosclerotic plaque stability through suppression of FAK-Akt-mTOR-NF-κB signalling pathway in ApoE−/− mice induced by Ang II. FAK is a member of the focal adhesions that mediates integrin-mediated signal transduction associated with a variety of cellular functions including cellular proliferation, migration, and adhesion. The downstream signaling pathways involved in FAK signaling include the Jun N-terminal kinases (JNK) survival pathway, and the phosphoinositide 3-kinase (PI3K)/Akt pathway [17]. PI3K/Akt controls cell proliferation, survival, and apoptosis. Our previous studies have found that silencing MR1 could inhibit FAK/Akt signal pathway induced by Ang II [1012]. Moreover, NF-κB signaling pathways are downstream targets of activated PI3K/Akt. Transcription factors NF-κB controls the expression of genes involved in immune and inflammatory reaction, and the mTOR plays an important role in cell survival, proliferation, and metabolism. Previous studies has also been suggested that inhibition of mTOR alleviated atherosclerosis and results in the selective elimination of macrophages in atherosclerotic plaques [1819]. We found that silencing of MR-1 in an atherosclerotic mouse model induced by Ang II resulted in a decrease of macrophages accumulation and the expression of proinflammatory factor MCP-1, TNF-α and matrix metalloproteinase MMP-2, which mediate the adhesion and recruitment of monocytes into the arterial wall, where they differentiate into macrophages [20]. Therefore, we demonstrated that siRNA-MR-1 reduced the severity of atherosclerosis by decreasing inflammation.
Macrophages are implicated in the lipoprotein uptake, vascular inflammation and the formation of atherosclerosis but also contributed to plaque destabilization [21]. Hence, our results suggest that targeting MR-1 to inhibit macrophages accumulation is a promising way for the treatment of the formation of atherosclerosis and atherosclerotic plaque stability. In additon, enhanced inflammation activity was also a hallmark of the formation of atherosclerosis and plaque rupture. Studies have suggested that MMP-2 was markedly increased in unstable plaques, demonstating that MMP-2 is an important risk factor for unstable plaques [22]. MCP-1 is highly expressed in macrophage-enriched plaques, suggesting pivotal roles in the processes of atherosclerosis formation and plaque instability [23]. In the same time, TNF-α also could contribute to the atherosclerotic lesion and plaques instability [24].
The present study shows that the knockdown of MR-1 suppressed atherosclerosis and promoted plaque stability induced by Ang II by decreasing the expression levels of MCP-1, TNF-α and MMP-2, atherosclerotic lesion macrophage content, plaque area and increasing the fibrous cap, which was beneficial for plaque stability. Moreover, it has been shown that mTOR signalling regulates lipid homoeostasis by activating sterol regulatory elementbinding proteins [25]. Accordingly, suppression of mTOR alleviated lipid metabolism and prevented macrophage accumulation. In our experiments, we demonstrated that silencing MR-1 can inhibite the levels of total cholesterol, triglycerides, free fatty acids and blood glucose. These data indicate that siRNA-MR-1 regulation of lipid homoeostasis is regulated at least in part by mTOR.
The method of RNAi which efficiently and selectively silences target gene expression has been implicated in experimental studies for the treatment of various diseases. In the present study, we successfully inhibited MR-1 expression in vivo in an atherosclerotic mouse model. In addition, MR-1 promoted the formation of atherosclerosis and plaque instability, including MMP-2, MCP-1 and TNF-α upregulated induced by Ang II. These results indicate that MR-1 is a promising target for the treatment of atherosclerosis.
Figures and Tables
Fig. 1
Upregulation of MR-1 expression in atherosclerotic aortas of ApoE−/− mice and VSMCs and macrophages.
(A) Aortic MR-1 mRNA expression after 4 weeks of Ang II+siRNA or Ang II+siRNA-control treatment. Data are mean±SEM (n=6 per group). (B) Aortic MR-1 protein expression after 4 weeks of Ang II+siRNA or Ang II+siRNA-control treatment. (C) Analysis of MR-1 protein levels of VSMCs and macrophages. (D) MR-1 colocalization with VSMC and macrophages in atherosclerotic plaques (double immunohistochemistry/ Immunofluorescence).
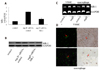
Fig. 2
siRNA-MR-1 exhibited a significant suppression of atheroma formation in the vascular induced by Ang II.
H&E staining quantitative analysis of (A) plaque area, (B) fibrous cap thickness, (C) cap-to-core ratio and (D) picrosirius red staining analysis of collagen area in three groups of mice. At least three independent experiments were performed and the data are presented as the mean±SEM. p<0.05 vs. siRNA-control denotes statistically significant difference. (E) The effect of siRNA-MR-1 on the expression of two macrophage marker genes, CD68 and F4/80 induced by Ang II in the vascular tissues.
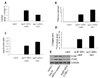
Fig. 3
siRNA-MR-1 inhibited vascular and cellular inflammatory gene expression and matrix metalloproteinase MMP-2 induced by Ang II.
(A) Western blot analysis of TNF-α, MCP-1 and MMP-2 protein expression in aortas (n=6). (B) Western blot analysis of TNF-α, MCP-1 and MMP-2 protein expression in VSMCs (n=6). Top, representative blots; bottom, quantitative results. Values are means±SEM. *p<0.05 vs. siRNA-control injected groups and untreated groups induced by Ang II. Experiments were repeated at least three times.
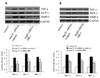
Fig. 4
Silencing MR-1 inhibites inflammatory signaling pathways in lesions of ApoE−/− mice induced by Ang II.
(A) siRNA-MR-1 markedly reduced the levels of p-FAK, p-Akt, p-mTOR and p-p65 in artery wall induced by Ang II compared with the siRNA-control group. (B) Rapamycin inhibited p65 phosphorylation and nuclear translocation in VSMCs. Western blot analysis of p65 translocation and phosphorylation in cytoplasmic extracts (CE) and nuclear extracts (NE) of 3 groups of VSMCs. (C) The phosphorylation of Akt was not affected by rapamycin. Each assay was done in triplicate.

ACKNOWLEDGEMENTS
This work was supported by grants from Science and technology project of hunan province department of education (13C251) and the first echelon pillar engineering fund of hunan Environment-Biologic Polytechnic.
Notes
Author contributions W.J.D. designed the entire project and write the articles. Y.G.W. directed the entire project. H.Y.L., Q.H.Z. and Y.X.C. managed literature searches and analyses. Y.X.C., J.P.C. and Q.H.Z. took care of animals and assisted most of animal experiments. W.J.D., Y.X.C., and Q.H.Z. undertook statistical analysis. W.J.D., Y.X.C. and Q.H.Z. performed DNA and protein expression analysis. All authors contributed to and approved the final version of the manuscript.
References
1. Dong X, Yu LG, Sun R, Cheng YN, Cao H, Yang KM, Dong YN, Wu Y, Guo XL. Inhibition of PTEN expression and activity by angiotensin II induces proliferation and migration of vascular smooth muscle cells. J Cell Biochem. 2013; 114:174–182.


2. Cho JR, Lee CY, Lee J, Seo HH, Choi E, Chung N, Kim SM, Hwang KC, Lee S. MicroRNA-761 inhibits Angiotensin II-induced vascular smooth muscle cell proliferation and migration by targeting mammalian target of rapamycin. Clin Hemorheol Microcirc. 2015; 63:45–56.


3. Bihl JC, Zhang C, Zhao Y, Xiao X, Ma X, Chen Y, Chen S, Zhao B, Chen Y. Angiotensin-(1-7) counteracts the effects of Ang II on vascular smooth muscle cells, vascular remodeling and hemorrhagic stroke: Role of the NFκB inflammatory pathway. Vascul Pharmacol. 2015; 73:115–123.


4. Moraes JA, Frony AC, Dias AM, Renovato-Martins M, Rodrigues G, Marcinkiewicz C, Assreuy J, Barja-Fidalgo C. Alpha1beta1 and integrin-linked kinase interact and modulate angiotensin II effects in vascular smooth muscle cells. Atherosclerosis. 2015; 243:477–485.


5. Zhang F, Ren X, Zhao M, Zhou B, Han Y. Angiotensin-(1-7) abrogates angiotensin II-induced proliferation, migration and inflammation in VSMCs through inactivation of ROS-mediated PI3K/Akt and MAPK/ERK signaling pathways. Sci Rep. 2016; 6:34621.


6. Fitzgibbons TP, Czech MP. Emerging evidence for beneficial macrophage functions in atherosclerosis and obesity-induced insulin resistance. J Mol Med (Berl). 2016; 94:267–275.


7. Pantan R, Tocharus J, Suksamrarn A, Tocharus C. Synergistic effect of atorvastatin and Cyanidin-3-glucoside on angiotensin II-induced inflammation in vascular smooth muscle cells. Exp Cell Res. 2016; 342:104–112.


8. Li TB, Liu XH, Feng S, Hu Y, Yang WX, Han Y, Wang YG, Gong LM. Characterization of MR-1, a novel myofibrillogenesis regulator in human muscle. Acta Biochim Biophys Sin (Shanghai). 2004; 36:412–418.


9. Dai W, He W, Shang G, Jiang J, Wang Y, Kong W. Gene silencing of myofibrillogenesis regulator-1 by adenovirus-delivered small interfering RNA suppresses cardiac hypertrophy induced by angiotensin II in mice. Am J Physiol Heart Circ Physiol. 2010; 299:H1468–H1475.


10. Dai W, Chen H, Jiang J, Kong W, Wang Y. Silencing MR-1 attenuates inflammatory damage in mice heart induced by AngII. Biochem Biophys Res Commun. 2010; 391:1573–1578.


11. Dai WJ, Zhang M, Chen JJ. Gene expression profiling study of angiotensin II-induced cardiac hypertrophy in response to silencing MR-1. Progress Biochem Biophys. 2011; 38:633–641.
12. Ren K, Jin H, Bian C, He H, Liu X, Zhang S, Wang Y, Shao RG. MR-1 modulates proliferation and migration of human hepatoma HepG2 cells through myosin light chains-2 (MLC2)/focal adhesion kinase (FAK)/Akt signaling pathway. J Biol Chem. 2008; 283:35598–35605.


13. Li HL, She ZG, Li TB, Wang AB, Yang Q, Wei YS, Wang YG, Liu DP. Overexpression of myofibrillogenesis regulator-1 aggravates cardiac hypertrophy induced by angiotensin II in mice. Hypertension. 2007; 49:1399–1408.


14. Chen YX, Zhang M, Cai Y, Zhao Q, Dai W. The Sirt1 activator SRT1720 attenuates angiotensin II-induced atherosclerosis in apoE–/– mice through inhibiting vascular inflammatory response. Biochem Biophys Res Commun. 2015; 465:732–738.
15. Kuzuya M, Nakamura K, Sasaki T, Cheng XW, Itohara S, Iguchi A. Effect of MMP-2 deficiency on atherosclerotic lesion formation in apoE-deficient mice. Arterioscler Thromb Vasc Biol. 2006; 26:1120–1125.


16. Cho JR, Lee CY, Lee J, Seo HH, Choi E, Chung N, Kim SM, Hwang KC, Lee S. MicroRNA-761 inhibits Angiotensin II-induced vascular smooth muscle cell proliferation and migration by targeting mammalian target of rapamycin. Clin Hemorheol Microcirc. 2015; 63:45–56.


17. Saranya J, Shilpa G, Raghu KG, Priya S. Morus alba leaf lectin (MLL) sensitizes MCF-7 cells to anoikis by inhibiting fibronectin mediated integrin-FAK signaling through ras and activation of P38 MAPK. Front Pharmacol. 2017; 8:34–46.


18. Zheng H, Cui D, Quan X, Yang W, Li Y, Zhang L, Liu E. Lp-PLA2 silencing protects against ox-LDL-induced oxidative stress and cell apoptosis via Akt/mTOR signaling pathway in human THP1 macrophages. Biochem Biophys Res Commun. 2016; 477:1017–1023.


19. Martinet W, Verheye S, De Meyer I, Timmermans JP, Schrijvers DM, Van Brussel I, Bult H, De Meyer GR. Everolimus triggers cytokine release by macrophages: rationale for stents eluting everolimus and a glucocorticoid. Arterioscler Thromb Vasc Biol. 2012; 32:1228–1235.
20. Choudhury RP, Fuster V, Fayad ZA. Molecular, cellular and functional imaging of atherothrombosis. Nat Rev Drug Discov. 2004; 3:913–925.


21. Harmon EY, Fronhofer V 3rd, Keller RS, Feustel PJ, Zhu X, Xu H, Avram D, Jones DM, Nagarajan S, Lennartz MR. Anti-inflammatory immune skewing is atheroprotective: Apoe−/− FcγRIIb−/− mice develop fibrous carotid plaques. J Am Heart Assoc. 2014; 3:e001232.


22. Van der Donckt C, Van Herck JL, Schrijvers DM, Vanhoutte G, Verhoye M, Blockx I, Van Der Linden A, Bauters D, Lijnen HR, Sluimer JC, Roth L, Van Hove CE, Fransen P, Knaapen MW, Hervent AS, De Keulenaer GW, Bult H, Martinet W, Herman AG, De Meyer GR. Elastin fragmentation in atherosclerotic mice leads to intraplaque neovascularization, plaque rupture, myocardial infarction, stroke, and sudden death. Eur Heart J. 2015; 36:1049–1058.


23. Rull A, Beltrán-Debón R, Aragonès G, Rodríguez-Sanabria F, Alonso-Villaverde C, Camps J, Joven J. Expression of cytokine genes in the aorta is altered by the deficiency in MCP-1: effect of a high-fat, high-cholesterol diet. Cytokine. 2010; 50:121–128.


24. Schneiderman J, Schaefer K, Kolodgie FD, Savion N, Kotev-Emeth S, Dardik R, Simon AJ, Halak M, Pariente C, Engelberg I, Konstantinides S, Virmani R. Leptin locally synthesized in carotid atherosclerotic plaques could be associated with lesion instability and cerebral emboli. J Am Heart Assoc. 2012; 1:e001727.

