Abstract
Exosomes are membranous vesicles of 30-150 nm in diameter that are derived from the exocytosis of the intraluminal vesicles of many cell types including immune cells, stem cells, cardiovascular cells and tumor cells. Exosomes participate in intercellular communication by delivering their contents to recipient cells, with or without direct contact between cells, and thereby influence physiological and pathological processes. They are present in various body fluids and contain proteins, nucleic acids, lipids, and microRNAs that can be transported to surrounding cells. Theragnosis is a concept in next-generation medicine that simultaneously combines accurate diagnostics with therapeutic effects. Molecular components in exosomes have been found to be related to certain diseases and treatment responses, indicating that they may have applications in diagnosis via molecular imaging and biomarker detection. In addition, recent studies have reported that exosomes have immunotherapeutic applications or can act as a drug delivery system for targeted therapies with drugs and biomolecules. In this review, we describe the formation, structure, and physiological roles of exosomes. We also discuss their roles in the pathogenesis and progression of diseases including neurodegenerative diseases, cardiovascular diseases, and cancer. The potential applications of exosomes for theragnostic purposes in various diseases are also discussed. This review summarizes the current knowledge about the physiological and pathological roles of exosomes as well as their diagnostic and therapeutic uses, including emerging exosome-based therapies that could not be applied until now.
Exosomes are cell-derived membrane vesicles of 30-150 nm in diameter that have their origin in late endosomes (Les) (Table 1) [1234]. Membrane receptors, soluble proteins, lipids, RNAs, and even organelles can be packed in exosomes as cargos that can be functional in recipient cells. As the first process of exosome biogenesis, a part of the cell membrane buds into the cell to form an early endosome. Then, early endosomes transform into LEs filled with intraluminal vesicles (ILVs), i.e., multivesicular bodies (MVBs) under the regulation of endocytosis-associated proteins, the Ras superfamily GTPase Rab and lipid raft complexes. MVBs are the precursor for exosomes. The composition of exosomes reflects that of ILVs, and their biogenesis is linked to endosome maturation. Finally, the ILVs are released from MVBs into the extracellular environment, generating exosomes [56]. Exosome generation can be either dependent on or independent of the endosomal sorting complexes required for transport (ESCRT) proteins (Fig. 1).
The ESCRT protein complexes are classified into four groups, namely ESCRT-0, -I, -II, and -III [7]. These complexes are sequentially gathered to the cytosolic surface of the endosomal membrane and drive the inward budding and fission of the membrane to form ILVs. ESCRT-0 initiates the ESCRT pathway at endosomes because it can bind phosphatidylinositol 3-phosphate and ubiquitin. The two subunits of ESCRT-0, namely Hrs and STAM1/2 bind ubiquitinated cargos. ESCRT-0 is recruited to pre-MVB endosomes because the FYVE domain of the Hrs subunit binds phosphatidylinositol 3-phosphate [8]. ESCRT-I and -II also have ubiquitin-interaction domains and sort ubiquitinated cargos at ILV with ESCRT-0. ESCRT-I and ESCRT-II recruit ESCRT-III, which drives the invagination and constriction of the membrane [9]. The ESCRT complexes accumulate ubiquitinated cargos in exosomes containing both membrane and cytoplasmic proteins. The ESCRT-1 complexes subunit TSG101 is essential for exosomal secretion. Some cargos can be targeted to MVBs without requiring interactions with ESCRT-0, -I and -II. ALIX, which is an ESCRT-interacting protein, binds to the G protein-coupled membrane receptor PAR1 and the ESCRT-III component CHMP4, and thereby functions in sorting PAR1 as the cargo to MVBs independently of ubiquitylation. ALIX also interacts with the PDZ scaffolding protein syntenin, which binds syndecans and CD63 as PDZ ligand cargos. Thus, ALIX, syntenin, syndecan and CD63 co-accumulate in MVBs, and thus exosomes independently of ubiquitination [910]. Lipid- and tetraspanin-required exosome biogenesis occur through ESCRT-independent pathways. Some proteins are targeted to exosomes via tetraspanins or protein lipidation, including the glycosylphosphatidyl inositol anchor and saturated fatty acid modifications. Exosomes are highly enriched in tetraspanins which have four transmembrane domains that contain distinct palmitoylation sites. Tetraspanins such as CD9, CD63, CD37, CD81, and CD82 are especially plentiful in the exosomal membrane and are used as exosome biomarkers [11]. CD55, CD58, and CD59, which are glycosylphosphatidyl inositol-anchored proteins, and Lyn, which is a palmitoylated protein, are targeted to exosomes during reticulocyte maturation. Proteins with these lipid modifications are selectively recruited into lipid rafts, which are composed of sphingomyelin, cholesterol and ceramide. The lipid rafts concentrate in the exosome membranes [612]. As a more immediate route of ESCRT-independent exosome biogenesis, the infection of cells by human immunodefiuciency virus (HIV)-1 increases the cellular release of exosomes. Exosomes from HIV-1 positive individuals contain the viral protein, Nef which is anchored to lipid raft micro-domains. These exosomes contain the tetraspanins-CD63 and CD81, which are classical exosome markers, and have a similar size to that of classical exosomes [113]. Exosomes derived from many cell types originate as cargo-containing ILVs within LEs or MVBs. Exosome biogenesis can be induced by the ESCRT complex, lipid rafts, and the tetraspanins. The ESCRT-0 complex clusters ubiquitinated cargoes, the ESCRT-I and -II complexes direct the membrane budding, and the ESCRT-III complex serves as the “molecular scissors” that cleave the formed vesicles from the membrane. Exosomes are highly enriched in sphingomyelin, cholesterol, and ceramide, which are concentrated in membrane lipid rafts. The highly fluid lipid raft microdomains are involved in the endocytic and exocytic processes. Tetraspanins play a role in the formation of the exosome. The proteins of the tetraspanin family, which contain four trnasmembranous domains, select cargoes for exosome secretion. Tetraspanins are plentiful in the ILVs of MVBs and in exosomes. Additionally, Nef, an accessory protein encoded by HIV-1, induces the extracellular release of exosomes.
As cell-derived extracellular vesicles (EVs), exosomes mediate intercellular communication and are thereby involved in the regulation of diverse biological processes. Exosomes may affect recipient cells by receptor-mediated interactions or by the delivery of biologically active cargo molecules, such as proteins, mRNAs and microRNAs (miRNAs) when the exosomes are taken up by the recipient cells through endocytosis, membrane combination, or phagocytosis [6]. The type and physiopathological state of the parent cells determine the constituents and structure of exosomes. Exosomes are potential transporters of several biomolecular cargos, including proteins, peptides, lipids, DNA, mRNA, and miRNA (Fig. 2).
Especially, genetic materials such as mRNA and miRNA as exosome cargo can be translated or regulate gene expression in the recipient or target cells [14]. In this way, exosomes can be involved in normal physiological processes such as immune responses, neurobiological functions and synaptic plasticity, cell differentiation, stem cell maintenance and plasticity, and tissue repair [15]. Exosomes are discharged from many cell types including red blood cells, platelets, lymphocytes, dendritic cells, and cancer cells [161718]. In vivo, exosomes are present in most body fluids including cerebrospinal fluid, breast milk, blood, urine, saliva, amniotic fluid, and cancer effusions of ascites. In blood, exosomes are present at a concentration of almost 3 million exosomes per microliter [19]. The fundamental processes by which exosomes exchange their contents with the recipient cells have not been clearly determined so far. Certain studies have demonstrated that exosomes can merge with the cell membrane of the recipient cells, which results in the insertion of the exosome contents into the recipient cell [202122]. This process of exosomes merging with the cell membrane of recipient cell is especially favored under acidic environments similar to those within cancer cells (Fig. 3).
The components, biogenesis, and discharge of exosomes are strongly directed procedures that are affected by various activating stimuli and stress signals that reflect changes in the environmental conditions. Furthermore, the release of exosomes is an effective adjustive mechanism by which cells regulates their internal stress states and the extracellular environment [23]. Stressful conditions occur frequently in the tumor microenvironment, and need to be overwhelmed by the cancer cells to allow tumor growth against unfavorable conditions such as hypoxia, cancer chemotherapy, irradiation, starvation, or other patient's factors. Hypoxia also results in the acidification of the tumor microenvironment, which may have an intense effect on EV trafficking, since the secretion and absorption of EVs remain active under acidic conditions [21]. Exosome-medicated signaling in cancers is thus influenced by various stressful situations [24], and can promote cancer development through interaction between the cancer cells and the neighboring stroma, the stimulation of proliferative and angiogenic signaling, the progression of immune repression, and the initiation of pre-metastatic niches (Fig. 4) [2225262728].
Other studies have suggested that the exosomes bind at the cell surface of the recipient cells through specific receptors [29] or undergo internalization by endocytosis or micropinocytosis following fusion with internal sections [3031]. Exosomes are now known to play crucial roles in most physiological processes in tissues and organs. Because exosomes are secreted by many cell types including immune cells, neural and stem cells, they may be involved in diverse physiological processes such as antigen presentation [32], RNA transfer between cells [33] or tissue repair [34]. Exosomes have been most intensively to investigate their roles in the following several areas; in immunology, regulating the behaviors of lymphocytes and macrophages; in the cardiovascular system, regulating coagulation, angiogenesis, and thrombosis; in the central nervous system (CNS), regulating the incorporation of neurons and glial cells including astrocytes, oligodendrocytes, and microglia in the regulation of synaptic function, neuronal plasticity, neuro-glial communication, and myelination; and in bones, regulating the expression of specific enzymes. A common mechanism that is active in many of the above processes is the exchange of RNAs among cells through EVs trafficking [3536373839404142]. Extensive studies have been performed on the facilitator effect of exosomes in the immune response [36] and their role in antigen presentation has also been extensively reported [43]. The immune response is a complex process involving both innate and acquired immunity [44]. These responses can be controlled by various components of exosomes, including proteins, RNAs, and lipids and this process are termed immunomodulation. The roles of exosomes in coagulation, inflammation and angiogenesis have also been reported [22]. After their activation, platelet secretes exosomes as well as shedding other microvesicles [45]. The activated platelet-derived exosomes were not found to perform any role in coagulation, but their contents of miR-223, miR-339 and miR-21 might be transferred into smooth muscle cells and inhibit the expression of platelet-derived growth factor β, and these exosomal miRNAs can be used as biomarkers for predicting atherosclerosis [46]. It has been reported that exosomes are involved in Dictyostelium cells by towards chemoattractant signals [47]. Studies on the miRNA contents of exosomes in human milk have reported that miR-155 and miR-181a, which play important roles in immune regulation, were present at high concentration during the first 6 months of lactation, but were significantly reduced afterward [4849]. Some studies have reported that the exosomes are not only involved in triggering downstream signaling but also specifically target recipient cells and exchange certain proteins and nucleic acids with those cells [50]. Exosomes have unique functions in mediating intercellular communication among both nearby and distant cells in the body. Similarly, exosomes play a unique role in spreading pathogens such as viruses and prions from cells to previously uninfected ones [51].
Exosomes have been recognized to have pathophysiological roles in diseases including cancer, infectious diseases, autoimmune diseases, metabolic diseases, cardiovascular diseases and neurodegenerative disorders. Based on their integral contents, exosomes play important roles in promoting tumor progression via their abilities to stimulate cell proliferation, angiogenesis, extracellular matrix remodeling, metastasis, and promoting immune surveillance escape [6]. Exosomes affect recipient cells by transferring carcinogenic biomolecules as their cargos. Upon entering target cells, these cargos contribute to the development of a cancer phenotype in the recipient cells [52]. Several molecules that act as The inducers of the epithelial-mesenchymal transition can be transferred to tumor cells as the cargos of exosomes. Because epithelial-mesenchymal transition is the critical event that initiates cancer invasion and metastasis, exosomes can contribute to the development of a high level of malignancy in tumor cells [6]. Importantly, exosomes can spread numerous pathogens, including HIV, Epstein–Barr virus, cytomegalovirus, hepatitis C virus, herpes simplex virus, Toxoplasma gondii, Leishmania spp., Plasmodium spp., Mycobacterium spp., Salmonella Typhimurium, Mycoplasma spp., and prions via the selective delivery of pathogen-derived cargos. After experimental infections with pathogens in cells or animals, the cargos of exosomes have been identified to comprise components of the donor cell alongside numerous pathogen-derived components [53]. Exosomes can activate or suppress immune responses by spreading microbial and donor components beyond the infected cell. Additionally, the infected cell-derived exosomes could interact with non-immune cells including epithelial cells, fibroblasts, mesenchymal cells, platelets, and vascular cells and could thereby influence the outcome of an infection [53]. Exosomes can also mediate the spread of neurodegenerative diseases. As a tool for inter-neuronal communication, exosomes can not only contribute to local synaptic plasticity but also allow communication within the CNS, thus influencing distant neuronal networks. This could provide a mechanism for the local propagation of neurodegenerative disease in the brain because exosomes containing misfolded, aggregated forms of neurodegenerative disease-associated proteins exist in the cerebrospinal fluid and blood of the patients [54]. These findings suggest that neurodegenerative diseases may be transmitted in the brain via exosomes [55]. Exosomes are elevated in the metabolic syndrome and contribute to its pathophysiological manifestations such as vascular complications, inflammation, and blood coagulopathy [56]. Exosomes are further increased in the metabolic syndrome and this change is often accompanied by vascular complications including atherosclerosis. An increased abundance of exosomes is also associated with obesity [57]. In obesity and type 2 diabetes mellitus, the abundance of exosomes has been reported to be reduced significantly after caloric restriction or bariatric surgery and the resultant normalization of glycemic control reflects an attenuation of inflammation [58]. The abundance of exosomes in the circulation has been found to increase in many inflammatory conditions including cardiovascular diseases [59]. Exosomes induce cytokine and chemokine release from endothelial cells and contribute to the propagation of endothelial pro-inflammatory cascades. By contrast, exosomes isolated from apoptotic endothelial cells, platelets, endothelial progenitor cells, or ischemic muscle have shown some beneficial effects such as the stimulation of endothelial proliferation, migration, tube formation in vitro, and endothelial repair. These beneficial functions of EVs are altered by diabetes. Circulating endothelial exosomes are also correlated with cardio metabolic risk factors, particularly dyslipidemia [60]. Uremia as a cardiovascular risk factor also correlates with an increased abundance of platelet-derived exosomes in the circulation to triggering thrombosis. Hyperuricemia in chronic renal failure patients can lead to raised risk of cardiovascular events. Thus, in hyperglycemia, dyslipidemia, and hyperinsulinemia, as well as hyperuricemia and uremia, exosomes can contribute to the development of cardio metabolic disease through their pro-coagulant activity and their diminished support of endothelial function [61]. Exosomes also contribute to the progression of autoimmune diseases. Since they can contain autoantigens that are involved in autoimmune diseases. Exosomes isolated from synovial fluids have been found to contain autoantigen proteins that might be involved to the development of rheumatoid arthritis (RA). Tumor necrosis factor-α-positive exosomes isolated from synovial fibroblasts obtained from patients with RA inhibit the apoptosis of T cells, further aggravating RA [6263].
Exosomes have been regarded as innovative targets [6465]. Diverse body fluids including breast milk, saliva, serum, plasma, amniotic fluid, malignant ascites fluid, and urine contains numerous exosomes that can be isolated [666768]. Exosome could have clinical applications both as diagnostic biomarkers and as therapeutic tools. Cells carry exosomes in both normal and pathological conditions. Exosomes may be able to carry various nucleic acids and proteins that provide information about pathological states from donor cells, and they are widely considered to have strong potential to contain valuable biomarkers for experimental and clinical diagnostics. Specific mRNAs/miRNAs have been discovered in exosomes isolated from the serum of patients with glioblastoma or ovarian carcinoma [2269]. Since it is a straightforward process to isolate exosomes from body fluids, they are attractive as diagnostic markers for cancer and other pathological conditions. Tumor cells have been described to release an increased amounts of exosomes as compared with non-tumor cells [70]. Such tumor-derived exosomes exhibit characteristic genomic and proteomic signatures; thus, they represent suitable targets for cancer diagnosis. However, these exosomes might stimulate the oncogenic potential of recipient cells [71]. Several studies have shown that exosomes released by tumor cells influence the development, differentiation and metastasis of cancer cells [22727374]. Clinical studies of exosomes in patients with lung cancer and melanoma have already been accomplished [7576]. Tetraspanins, which are a family of membrane-associated with proteins, are plentiful in exosomes. The exosomal marker CD63 belong to the tetraspanin family and the abundance of CD63 exosomes in plasma was reported to be significantly increased in patients with melanoma as compared with healthy controls [70]. More recently, a comparative analysis of exosomal protein was performed in various types of human cancer and the results showed that CD63 was higher in exosomes derived from malignant cancer cells than in those derived from non-cancer cell lines, providing further evidence that exosomal CD63 could be a biomarker of cancer [77]. Urinary exosomal proteins have also been studied as potential biomarkers for bladder cancer and prostate cancer. The potential exosomal biomarkers for bladder cancer comprise eight urinary exosomal proteins, namely, five proteins associated with the epidermal growth factor receptor pathway, the α-subunit of Gs protein, resistin, and retinoic acid-induced protein 3, which were identified by comparing the proteomic profiles of urinary exosomes between patients with bladder cancer and healthy controls [78]. Futhermore, the abundance of 24 urinary exosomal proteins significantly differed between bladder cancer and hernia (control) patients [79]. The early detection and diagnosis of prostate cancer may be achieved using the prostate-specific antigen test; however, it has the problems of a low specificity and a high rate of false-positives, which may result in the overtreatment of indolent prostate cancers. Therefore, new biomarkers with a higher diagnostic accuracy are much needed for prostate cancer. The circulating concentration of miRNA-141 is a robust diagnostic biomarker for prostate cancer [80]. Furthermore, the concentration of miRNA-141 and miRNA-375 in serum were reported to be correlated with the progression of prostate cancer [81]. Exosomal miRNAs are a major component of RNase-resistant miRNAs in serum or plasma specimens [8082], so it is plausible to speculate that the circulating concentrations of exosomal miRNA-141 and miRNA-375 may be valuable biomarkers for the diagnosis of prostate cancer [83]. The presence of two known prostate cancer biomarkers, namely PCA-3 and TMRPRSS2:ERG, was demonstrated in urinary exosomes collected from prostate cancer patients [84]. The concentration of exosomal miRNA-21 in serum was found to be increased in patients with esophageal squamous cell cancer(ESCC) as compared against serum from patients with benign tumors without systemic inflammation, exosomal miRNA-21 also show potential as biomarkers for the diagnosis of esophageal squamous cell cancer [85]. The concentration of miRNA-1246 was also reported to be elevated in exosomes isolated from the serum samples of patients with ESCC, it was not upregulated in ESCC tissue samples [86]. This finding suggests that circulating exosomal miRNA-1246, but not biopsy-derived miRNA-1246, has a significant potential as a new diagnostic and prognostic biomarker in ESCC. Otherwise, exosomal miRNAs have also demonstrated a high potential as diagnostic biomarkers for cardiovascular diseases and renal fibrosis [8788]. Serum proteins in urinary exosomes, which can be easily by noninvasive methods, have also been exploited for their potential utilities in diagnostics, especially for urinary tract diseases. Urinary exosomal fetuin-A was reported to be increased in intensive care unit patients with acute kidney injury (AKI) as compared with patients without AKI [89]. Additionally, activating transcription factor 3 was found in exosomes collected from patients with AKI but not in those collected from patients with chronic kidney disease or controls [90]. Aquaporin 1 and 2 have also been considered as biomarkers of renal ischemia/reperfusion injury and the action of antidiuretic hormone [91]. Exosomes also exist in human saliva and could be a source of disease biomarkers since they contain nucleic acids and proteins [92]. Research on exosomes over the past decade has highlighted their promising potential application as sources of biomarkers in clinical diagnostics. Scientific and technical advances in exosome isolation and function have facilitated exosome research and made exosomal diagnostics more cost-efficient. In general, exosomal biomarkers are still in the early discovery/development phase, but it is clear that they have a considerable potential for widespread use in next generation of clinical diagnostics. The developing field of exosomal diagnostics is anticipated to yield innovative biofluid-based molecular diagnostic methods that can significantly contribute to efficient personalized medicine.
EVs that are naturally released from certain cell types exhibit a potential for use as therapeutic tools. Mesenchymal stem cell (MSC)-derived EVs recapitulate the immunomodulatory and cytoprotective activities of their parent cells [9394]. Human dendritic cell-derived exosomes bearing H-Y peptide triggered naïve Marilyn CD4+ transgenic T cells in vivo. However, those exosomes were only immunogenic when they were incubated with mature dendritic cells that had not previously encountered antigens [16]. Human dendritic cell-derived exosomes (DEX) loaded with major histocompatibility complex (MHC) class I restricted peptides activate the peptide-specific CD8+ T cell clone only in the presence of dendritic cell lacking the proper MHC class I component [9596]. Human dendritic cells generated a competent exosome-associated MHC class I complex that was proficient in priming cytotoxic T lymphocytes in vivo, but the priming process required mature dendritic cells [9597]. Bone marrow MSC-derived exosomes were protective in models of myocardial ischemia/reperfusion injury [34], hypoxia-induced pulmonary hypertension [98], and brain injury [99100]. These exosomes likewise stimulated neurite outgrowth in cultured astrocytes by transferring miR-133b between cells [101]. Human umbilical cord MSC-derived EVs were protective in models of acute renal injury [102] and liver fibrosis [103]. MSC-derived exosomes inhibited breast cancer growth via vascular endothelial growth factor down-regulation and miR-16 transfer in mice [104]. However, the altered functionalities of cancer cell lines [105], attenuated immune rejection of tumor [106], and increased tumor growth as a result of exosome treatments have also been reported. Additionally, islet MSC-derived exosomes induced autoimmunity in non-obese diabetic mice [107]. Exosomes are easy to handle and can easily be applied to transfer proteins and genes among cells. These characteristics of exosomes indicate their potential utilities for the inhibition of pathological angiogenesis and cancer metastasis, which are two main targets of cancer therapy [108109]. Moreover, exosomes have been discussed as potential cancer vaccines [110]. The objective of cancer immunotherapy is to promote tumor-targeting immunity or support a continuing antitumor host immune response that is otherwise ineffective for reasons such as immunosuppression in the tumor microenvironment. Strategies include the activation of dendritic cells or their functions by inducing tumor-associated-antigen-specific T cell responses. Indeed, the adoptive transfers of tumor-associated-antigen-loaded mature autologous dendritic cells was reported to extend overall survival in patients with metastatic castration-resistant prostate cancer [111]. The unique characteristics of exosomes, including their known molecular composition and critical immunogenicity, facilitate their production using ‘good manufacturing practice (GMP)’ processes, which allows clinical trials using DEX to be performed in metastatic-tumor-bearing patients. Fifteen advanced (stage III/IV) melanoma patients with tumors bearing the MAGE-3 antigen participated and accomplished a first phase I study on the feasibility and safety of DEX administration in France [75]. In that trial, DEX was administrated by subcutaneous/intradermal injections every week for first 4 weeks then every 3 weeks thereafter in patients who attained a stable disease or showed an objective tumor response. Patients who were injected with DEX 6-120 times showed a dramatic increase in concentration of exosome in their blood circulation. The therapy schedule was controlled well and there were no toxic reactions exceeding NCI-CTC grade 2. Five patients obtained some positive clinical effects (one partial, one minor, one mixed response and two stable diseases) on target lesions in the skin and lymph nodes. Two patients who were treated with the standard MAGE-3 vaccination exhibited a clinical response to DEX treatment [75]. After the exosome therapy, T cell immunomonitoring revealed that the CD122 molecule (interleukin-2 receptor β chain) was upregulated in CD4+ T cells, but no major changes in other T cell markers were found. While the lymphocyte pool remained stable throughout the exosome therapy, after three rounds of vaccinations with the exosomes, the abundance of circulating CD3−/CD56+ natural killer (NK) cells dramatically increased. A phase II clinical trial was performed for evaluating the clinical benefit of IFN-ν-DEX, containing MHC class I- and class II-restricted cancer antigens as a preservation immunotherapy strategy after induction chemotherapy in patients bearing inoperable non-small cell lung cancer (NSCLC) without tumor progression. The results showed an increase in NKp30-dependent NK cell functions in a fraction of the NSCLC patients who presented with imperfect NKp30 expression. Significantly, the MHC class II expression levels of the final IFN-ν-DEX products were correlated with the expression levels of the NKp30 ligand BAG6 on DEX and with NKp30-dependent NK cell functions. The latter parameter was also correlated with a longer progression-free survival. Therefore, this phase II trial defined the capability of DEX administration to augment the NK cell response of antitumor immunity in patients with advanced NSCLC [112]. The fundamental treatment of patients with advanced NSCLC is based on four to six cycles of platinum-based doublet chemotherapy. Although the quality of life and survival can be improved by that treatment compared to the best supportive care, toxicity remains a serious challenge and different treatment strategies have been intensively explored [113]. In particular, various research efforts have focused on extending the benefit of first-line chemotherapy, which is defined as the ‘maintenance’ strategy. To date, the approved therapies have included the prolongation of one or more of the drugs used in the induction regimen (continuation maintenance) or the application of a different regimen with no cross-resistance (switching maintenance or early second-line therapy) [114]. After the phase I trials of DEX, innovative methods to develop DEX as an immunotherapy have been investigated with the aim of further improving the partial DEX-induced T cell responses. The use of exosomes derived from TLR4L- or IFN-ν-maturated dendritic cells induce greater T cell stimulation than immature dendritic cell-induced exosome and this method has been an important innovation in therapeutic application of exosome [115116117]. Exosomes from tumor cells stimulate immunosuppression through the promotion of T cell apoptosis, the suppression of dendritic cell differentiation and NK cytotoxicity, and the stimulation of immunosuppressive myeloid suppressor cells and regulatory T cells [118]. Certain situations, such as thermal and oxidative stresses, can enhance the release of immunosuppressive exosomes from leukemia and lymphoma T and B cells [119] and promote changes in the proteomic and genetic content of exosomes that influence the responses of recipient cells to stress signals (Fig. 4) [120121].
Exosomes can be used as targeted drug delivery systems. Exosomes derived from immature dendritic cell from the bone marrow of mouse were loaded with stimulatory molecules such as MHCII and CD80 [76]. Exosomes purified by ultracentrifugation have been used as carrier for short interfering RNA (siRNA) delivery in both in vitro and in vivo studies. Those studies selected brain as a target tissue, because it is believed that the blood-brain barrier represents an impediment to drug delivery to the CNS. The blood-brain barrier represents an impediment to drug delivery because it is composed of capillary endothelial cells that are tightly sealed by intercellular junctions and regulate the barrier functions [43]. Exosomes carry naturally occurring RNAs across the blood-brain barrier and might provide effective delivery methods. The concept of using exosomes for nucleic acid or drug delivery systems has been proposed in some studies that have demonstrated the transport of intracellular mRNAs and miRNAs from the exosome-producing cells to other cultured cells [2233]. In addition, JS1124, which is an inhibitor of signal transducer and activator of transcription proteins, and curcumin were successfully transported into microglia cells through the intranasal route in mice [122]. Drug-loaded exosomes were selectively taken up by the microglia cells and later induced their apoptosis after the intranasal delivery of the exosomes in mice [122]. To assure the targeted delivery of the exosomes, the innovative strategy of employing LAMP2B, which is an exosomal membrane surface protein, to display the targeted peptide on the exosome surfaces was used. The feasibility of using exosomes for delivery of siRNA was proved by the strong mRNA (60%) and protein (62%) knockdown of BACE1, which is a therapeutic target in Alzheimer's disease, in wild-type mice [123]. For the clinical application of this method, a source of ‘self’ exosomes will be required, along with the technical capabilities to load them with therapeutic agents such as chemically modified siRNAs and engineer them with appropriate targeting moieties [123]. Mammalian cells actively secrete exosomes that have diameters of 40-150 nm and contain various proteins and RNAs with many regulatory functions that may modify the phenotypes of recipient cells [124125]. Since nanosized exosomes can carry cell surface molecules, they have not only a high ability to penetrate the interstitial tissue of organs but also a natural targeting ability [123126127]. Despite those advantages, most mammalian cells secrete relatively low amounts of exosomes and it is a challenge to purify exosomes at sufficiently high yield [128]. Therefore, the production of nanosized artificial exosome-mimicking vesicles at a substantially greater yield is an attractive methodology for the development of exosome-based drug delivery systems [129]. New developed bioinspired cell-derived nanocarriers, which have been termed exosome-mimetic nanovesicles combine the characteristics of cells and nanocarriers, and have the potential to be applied for the targeted delivery of anticancer drugs. By subjecting cells of various origins to serial extrusion through filters with diminishing pore sizes after loading the cells with chemotherapeutic agents, high quantities of exosome-mimetic nanovesicles carrying sheltered drugs with 100-fold higher production yield were generated. Furthermore, the efficient and dose-dependent delivery of chemotherapeutic agents to cells and tissues using these exosomes has been demonstrated. Therefore, these bioengineered drug-loaded nanovesicles can serve as novel exosome-mimetics to effectively deliver chemotherapeutic agents to treat malignant tumors [129].
In summary, it is well known that most cell types, especially cancer cell secrete exosomes into extracellular spaces and detect it in various body fluids. We described the formation, structure, and physiological roles of exosomes. We also discuss their roles in the pathogenesis and progression of diseases including neurodegenerative diseases, cardiovascular diseases, and cancer and also the potential applications of exosomes for theragnostic purposes in various diseases. This review summarizes the current knowledge about the physiological and pathological roles of exosomes as well as their diagnostic and therapeutic uses, including emerging exosome-based therapies and exosome-mimicking drug delivery system that could not be applied until now.
Figures and Tables
Fig. 3
Transportation of exosomes from 1) donor cells to 2) recipient cells.
Exosomes are released from donor cells and uptake by recipient cells. The exosomes can be (a) fused with the plasma membrane or be (b) internalized by recipient cells. MVE, multivesicular endosome.
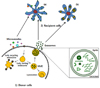
ACKNOWLEDGEMENTS
This work was supported by the Biomedical Technology Development Project, National Research Foundation, KOREA (NRF-2015M3A9B5029741).
Notes
References
1. van der Pol E, Böing AN, Harrison P, Sturk A, Nieuwland R. Classification, functions, and clinical relevance of extracellular vesicles. Pharmacol Rev. 2012; 64:676–705.


2. Kalra H, Drummen GP, Mathivanan S. Focus on extracellular vesicles: introducing the next small big thing. Int J Mol Sci. 2016; 17:170.


3. Zaborowski MP, Balaj L, Breakefield XO, Lai CP. Extracellular vesicles: composition, biological relevance, and methods of study. Bioscience. 2015; 65:783–797.


4. Hurley JH. ESCRTs are everywhere. EMBO J. 2015; 34:2398–2407.
6. Xu W, Zeng S, Li M, Fan Z, Zhou B. Aggf1 attenuates hepatic inflammation and activation of hepatic stellate cells by repressing Ccl2 transcription. J Biomed Res. 2016; 31:1–9.


7. Kowal J, Tkach M, Théry C. Biogenesis and secretion of exosomes. Curr Opin Cell Biol. 2014; 29:116–125.


10. Baietti MF, Zhang Z, Mortier E, Melchior A, Degeest G, Geeraerts A, Ivarsson Y, Depoortere F, Coomans C, Vermeiren E, Zimmermann P, David G. Syndecan-syntenin-ALIX regulates the biogenesis of exosomes. Nat Cell Biol. 2012; 14:677–685.


11. Andreu Z, Yáñez-Mó M. Tetraspanins in extracellular vesicle formation and function. Front Immunol. 2014; 5:442.


12. Yuyama K, Igarashi Y. Physiological and pathological roles of exosomes in the nervous system. Biomol Concepts. 2016; 7:53–68.


13. Madison MN, Okeoma CM. Exosomes: implications in HIV-1 pathogenesis. Viruses. 2015; 7:4093–4118.


14. Peterson MF, Otoc N, Sethi JK, Gupta A, Antes TJ. Integrated systems for exosome investigation. Methods. 2015; 87:31–45.


15. EL Andaloussi S, Mäger I, Breakefield XO, Wood MJ. Extracellular vesicles: biology and emerging therapeutic opportunities. Nat Rev Drug Discov. 2013; 12:347–357.


16. Théry C, Zitvogel L, Amigorena S. Exosomes: composition, biogenesis and function. Nat Rev Immunol. 2002; 2:569–579.


17. Andre F, Schartz NE, Movassagh M, Flament C, Pautier P, Morice P, Pomel C, Lhomme C, Escudier B, Le Chevalier T, Tursz T, Amigorena S, Raposo G, Angevin E, Zitvogel L. Malignant effusions and immunogenic tumour-derived exosomes. Lancet. 2002; 360:295–305.


18. Hendrix A, Westbroek W, Bracke M, De Wever O. An ex(o)citing machinery for invasive tumor growth. Cancer Res. 2010; 70:9533–9537.


19. Zwicker JI, Liebman HA, Neuberg D, Lacroix R, Bauer KA, Furie BC, Furie B. Tumor-derived tissue factor-bearing microparticles are associated with venous thromboembolic events in malignancy. Clin Cancer Res. 2009; 15:6830–6840.


20. Barry OP, Praticò D, Savani RC, FitzGerald GA. Modulation of monocyte-endothelial cell interactions by platelet microparticles. J Clin Invest. 1998; 102:136–144.


21. Parolini I, Federici C, Raggi C, Lugini L, Palleschi S, De Milito A, Coscia C, Iessi E, Logozzi M, Molinari A, Colone M, Tatti M, Sargiacomo M, Fais S. Microenvironmental pH is a key factor for exosome traffic in tumor cells. J Biol Chem. 2009; 284:34211–34222.


22. Skog J, Würdinger T, van Rijn S, Meijer DH, Gainche L, Sena-Esteves M, Curry WT Jr, Carter BS, Krichevsky AM, Breakefield XO. Glioblastoma microvesicles transport RNA and proteins that promote tumour growth and provide diagnostic biomarkers. Nat Cell Biol. 2008; 10:1470–1476.


23. Villarroya-Beltri C, Baixauli F, Gutiérrez-Vázquez C, Sánchez-Madrid F, Mittelbrunn M. Sorting it out: regulation of exosome loading. Semin Cancer Biol. 2014; 28:3–13.


24. de Jong OG, Verhaar MC, Chen Y, Vader P, Gremmels H, Posthuma G, Schiffelers RM, Gucek M, van Balkom BW. Cellular stress conditions are reflected in the protein and RNA content of endothelial cell-derived exosomes. J Extracell Vesicles. 2012; 1:18396. DOI: 10.3402/jev.v1i0.18396.


25. Grange C, Tapparo M, Collino F, Vitillo L, Damasco C, Deregibus MC, Tetta C, Bussolati B, Camussi G. Microvesicles released from human renal cancer stem cells stimulate angiogenesis and formation of lung premetastatic niche. Cancer Res. 2011; 71:5346–5356.


26. Peinado H, Alečkovič M, Lavotshkin S, Matei I, Costa-Silva B, Moreno-Bueno G, Hergueta-Redondo M, Williams C, García-Santos G, Ghajar C, Nitadori-Hoshino A, Hoffman C, Badal K, Garcia BA, Callahan MK, Yuan J, Martins VR, Skog J, Kaplan RN, Brady MS, Wolchok JD, Chapman PB, Kang Y, Bromberg J, Lyden D. Melanoma exosomes educate bone marrow progenitor cells toward a pro-metastatic phenotype through MET. Nat Med. 2012; 18:883–891.


27. Wieckowski EU, Visus C, Szajnik M, Szczepanski MJ, Storkus WJ, Whiteside TL. Tumor-derived microvesicles promote regulatory T cell expansion and induce apoptosis in tumor-reactive activated CD8+ T lymphocytes. J Immunol. 2009; 183:3720–3730.


28. Szajnik M, Czystowska M, Szczepanski MJ, Mandapathil M, Whiteside TL. Tumor-derived microvesicles induce, expand and upregulate biological activities of human regulatory T cells (Treg). PLoS One. 2010; 5:e11469.


29. Segura E, Guérin C, Hogg N, Amigorena S, Théry C. CD8+ dendritic cells use LFA-1 to capture MHC-peptide complexes from exosomes in vivo. J Immunol. 2007; 179:1489–1496.
30. Morelli AE, Larregina AT, Shufesky WJ, Sullivan ML, Stolz DB, Papworth GD, Zahorchak AF, Logar AJ, Wang Z, Watkins SC, Falo LD Jr, Thomson AW. Endocytosis, intracellular sorting, and processing of exosomes by dendritic cells. Blood. 2004; 104:3257–3266.


31. Fitzner D, Schnaars M, van Rossum D, Krishnamoorthy G, Dibaj P, Bakhti M, Regen T, Hanisch UK, Simons M. Selective transfer of exosomes from oligodendrocytes to microglia by macropinocytosis. J Cell Sci. 2011; 124:447–458.


32. Théry C, Boussac M, Véron P, Ricciardi-Castagnoli P, Raposo G, Garin J, Amigorena S. Proteomic analysis of dendritic cell-derived exosomes: a secreted subcellular compartment distinct from apoptotic vesicles. J Immunol. 2001; 166:7309–7318.


33. Valadi H, Ekström K, Bossios A, Sjöstrand M, Lee JJ, Lötvall JO. Exosome-mediated transfer of mRNAs and microRNAs is a novel mechanism of genetic exchange between cells. Nat Cell Biol. 2007; 9:654–659.


34. Lai RC, Arslan F, Lee MM, Sze NS, Choo A, Chen TS, Salto-Tellez M, Timmers L, Lee CN, El Oakley RM, Pasterkamp G, de Kleijn DP, Lim SK. Exosome secreted by MSC reduces myocardial ischemia/reperfusion injury. Stem Cell Res. 2010; 4:214–222.


35. Bobrie A, Théry C. Unraveling the physiological functions of exosome secretion by tumors. Oncoimmunology. 2013; 2:e22565.


36. Cocucci E, Racchetti G, Meldolesi J. Shedding microvesicles: artefacts no more. Trends Cell Biol. 2009; 19:43–51.


37. Crescitelli R, Lässer C, Szabó TG, Kittel A, Eldh M, Dianzani I, Buzás EI, Lötvall J. Distinct RNA profiles in subpopulations of extracellular vesicles: apoptotic bodies, microvesicles and exosomes. J Extracell Vesicles. 2013; 2:DOI: 10.3402/jev.v2i0.20677.


38. Montecalvo A, Larregina AT, Shufesky WJ, Stolz DB, Sullivan ML, Karlsson JM, Baty CJ, Gibson GA, Erdos G, Wang Z, Milosevic J, Tkacheva OA, Divito SJ, Jordan R, Lyons-Weiler J, Watkins SC, Morelli AE. Mechanism of transfer of functional microRNAs between mouse dendritic cells via exosomes. Blood. 2012; 119:756–766.


39. Li J, Zhang Y, Liu Y, Dai X, Li W, Cai X, Yin Y, Wang Q, Xue Y, Wang C, Li D, Hou D, Jiang X, Zhang J, Zen K, Chen X, Zhang CY. Microvesicle-mediated transfer of microRNA-150 from monocytes to endothelial cells promotes angiogenesis. J Biol Chem. 2013; 288:23586–23596.


40. Skinner AM, O'Neill SL, Kurre P. Cellular microvesicle pathways can be targeted to transfer genetic information between non-immune cells. PLoS One. 2009; 4:e6219.


41. Hannafon BN, Ding WQ. Intercellular communication by exosome-derived microRNAs in cancer. Int J Mol Sci. 2013; 14:14240–14269.


42. Raposo G, Stoorvogel W. Extracellular vesicles: exosomes, microvesicles, and friends. J Cell Biol. 2013; 200:373–383.


43. Ahmad M, Iqbal M, Akhtar N, Murtaza G, Madni MA, Rasool F. Comparison of bioavailability and pharmacokinetics of diclofenac sodium and diclofenac potassium in healthy and Escherichia coli induced febrile rabbits. Pakistan J Zool. 2010; 42:395–400.
44. Hoebe K, Janssen E, Beutler B. The interface between innate and adaptive immunity. Nat Immunol. 2004; 5:971–974.


45. Tanaka M, Oikawa K, Takanashi M, Kudo M, Ohyashiki J, Ohyashiki K, Kuroda M. Down-regulation of miR-92 in human plasma is a novel marker for acute leukemia patients. PLoS One. 2009; 4:e5532.


46. Tan M, Yan HB, Li JN, Li WK, Fu YY, Chen W, Zhou Z. Thrombin stimulated platelet-derived exosomes inhibit platelet-derived growth factor receptor-beta expression in vascular smooth muscle cells. Cell Physiol Biochem. 2016; 38:2348–2365.


47. Khiljee S, Ahmad M, Murtaza G, Madni A, Akhtar N, Akhtar M. Bioequivalence evaluation of norfloxacin tablets based on in vitro - in vivo correlation. Pak J Pharm Sci. 2011; 24:421–426.
48. Raposo G, Nijman HW, Stoorvogel W, Liejendekker R, Harding CV, Melief CJ, Geuze HJ. B lymphocytes secrete antigen-presenting vesicles. J Exp Med. 1996; 183:1161–1172.


49. Mittelbrunn M, Gutiérrez-Vázquez C, Villarroya-Beltri C, González S, Sánchez-Cabo F, González MÁ, Bernad A, Sánchez-Madrid F. Unidirectional transfer of microRNA-loaded exosomes from T cells to antigen-presenting cells. Nat Commun. 2011; 2:282.


50. Yang C, Robbins PD. The roles of tumor-derived exosomes in cancer pathogenesis. Clin Dev Immunol. 2011; 2011:842849.


51. Ahmad M, Ahmad R, Murtaza G. Comparative bioavailability and pharmacokinetics of flurbiprofen 200 mg SR pellets from India and France. Adv Clin Exp Med. 2011; 20:599–604.
52. Zhang HG, Grizzle WE. Exosomes: a novel pathway of local and distant intercellular communication that facilitates the growth and metastasis of neoplastic lesions. Am J Pathol. 2014; 184:28–41.
53. Schorey JS, Cheng Y, Singh PP, Smith VL. Exosomes and other extracellular vesicles in host-pathogen interactions. EMBO Rep. 2015; 16:24–43.


54. El Andaloussi S, Lakhal S, Mäger I, Wood MJ. Exosomes for targeted siRNA delivery across biological barriers. Adv Drug Deliv Rev. 2013; 65:391–397.


55. Howitt J, Hill AF. Exosomes in the pathology of neurodegenerative diseases. J Biol Chem. 2016; 291:26589–26597.


56. Lakhter AJ, Sims EK. Minireview: emerging roles for extracellular vesicles in diabetes and related metabolic disorders. Mol Endocrinol. 2015; 29:1535–1548.


57. Stepanian A, Bourguignat L, Hennou S, Coupaye M, Hajage D, Salomon L, Alessi MC, Msika S, de Prost D. Microparticle increase in severe obesity: not related to metabolic syndrome and unchanged after massive weight loss. Obesity (Silver Spring). 2013; 21:2236–2243.


58. Cheng V, Kashyap SR, Schauer PR, Kirwan JP, McCrae KR. Restoration of glycemic control in patients with type 2 diabetes mellitus after bariatric surgery is associated with reduction in microparticles. Surg Obes Relat Dis. 2013; 9:207–212.


59. Suades R, Padró T, Badimon L. The role of blood-borne microparticles in inflammation and hemostasis. Semin Thromb Hemost. 2015; 41:590–606.


60. Amabile N, Cheng S, Renard JM, Larson MG, Ghorbani A, Mc-Cabe E, Griffin G, Guerin C, Ho JE, Shaw SY, Cohen KS, Vasan RS, Tedgui A, Boulanger CM, Wang TJ. Association of circulating endothelial microparticles with cardiometabolic risk factors in the Framingham Heart Study. Eur Heart J. 2014; 35:2972–2979.


61. Lawson C, Vicencio JM, Yellon DM, Davidson SM. Microvesicles and exosomes: new players in metabolic and cardiovascular disease. J Endocrinol. 2016; 228:R57–R71.


62. Yang C, Robbins PD. Immunosuppressive exosomes: a new approach for treating arthritis. Int J Rheumatol. 2012; 2012:573528.


63. Tran TH, Nguyen HT, Pham TT, Choi JY, Choi HG, Yong CS, Kim JO. Development of a graphene oxide nanocarrier for dual-drug chemo-phototherapy to overcome drug resistance in cancer. ACS Appl Mater Interface. 2015; 7:28647–28655.


64. Pisitkun T, Johnstone R, Knepper MA. Discovery of urinary biomarkers. Mol Cell Proteomics. 2006; 5:1760–1771.


65. Simpson RJ, Lim JW, Moritz RL, Mathivanan S. Exosomes: proteomic insights and diagnostic potential. Expert Rev Proteomics. 2009; 6:267–283.


66. Runz S, Keller S, Rupp C, Stoeck A, Issa Y, Koensgen D, Mustea A, Sehouli J, Kristiansen G, Altevogt P. Malignant ascites-derived exosomes of ovarian carcinoma patients contain CD24 and EpCAM. Gynecol Oncol. 2007; 107:563–571.


67. Lässer C, Alikhani VS, Ekström K, Eldh M, Paredes PT, Bossios A, Sjöstrand M, Gabrielsson S, Lötvall J, Valadi H. Human saliva, plasma and breast milk exosomes contain RNA: uptake by macrophages. J Transl Med. 2011; 9:9.


68. Keller S, Rupp C, Stoeck A, Runz S, Fogel M, Lugert S, Hager HD, Abdel-Bakky MS, Gutwein P, Altevogt P. CD24 is a marker of exosomes secreted into urine and amniotic fluid. Kidney Int. 2007; 72:1095–1102.


69. Taylor DD, Homesley HD, Doellgast GJ. “Membrane-associated” immunoglobulins in cyst and ascites fluids of ovarian cancer patients. Am J Reprod Immunol. 1983; 3:7–11.


70. Logozzi M, De Milito A, Lugini L, Borghi M, Calabrò L, Spada M, Perdicchio M, Marino ML, Federici C, Iessi E, Brambilla D, Venturi G, Lozupone F, Santinami M, Huber V, Maio M, Rivoltini L, Fais S. High levels of exosomes expressing CD63 and caveolin-1 in plasma of melanoma patients. PLoS One. 2009; 4:e5219.


71. Mathivanan S, Ji H, Simpson RJ. Exosomes: extracellular organelles important in intercellular communication. J Proteomics. 2010; 73:1907–1920.


72. Qu JL, Qu XJ, Zhao MF, Teng YE, Zhang Y, Hou KZ, Jiang YH, Yang XH, Liu YP. Gastric cancer exosomes promote tumour cell proliferation through PI3K/Akt and MAPK/ERK activation. Dig Liver Dis. 2009; 41:875–880.


73. McCready J, Sims JD, Chan D, Jay DG. Secretion of extracellular hsp90alpha via exosomes increases cancer cell motility: a role for plasminogen activation. BMC Cancer. 2010; 10:294.


75. Lamparski HG, Metha-Damani A, Yao JY, Patel S, Hsu DH, Ruegg C, Le Pecq JB. Production and characterization of clinical grade exosomes derived from dendritic cells. J Immunol Methods. 2002; 270:211–226.


76. Morse MA, Garst J, Osada T, Khan S, Hobeika A, Clay TM, Valente N, Shreeniwas R, Sutton MA, Delcayre A, Hsu DH, Le Pecq JB, Lyerly HK. A phase I study of dexosome immunotherapy in patients with advanced non-small cell lung cancer. J Transl Med. 2005; 3:9.
77. Yoshioka Y, Konishi Y, Kosaka N, Katsuda T, Kato T, Ochiya T. Comparative marker analysis of extracellular vesicles in different human cancer types. J Extracell Vesicles. 2013; 2:DOI: 10.3402/jev.v2i0.20424.


78. Smalley DM, Sheman NE, Nelson K, Theodorescu D. Isolation and identification of potential urinary microparticle biomarkers of bladder cancer. J Proteome Res. 2008; 7:2088–2096.


79. Chen CL, Lai YF, Tang P, Chien KY, Yu JS, Tsai CH, Chen HW, Wu CC, Chung T, Hsu CW, Chen CD, Chang YS, Chang PL, Chen YT. Comparative and targeted proteomic analyses of urinary microparticles from bladder cancer and hernia patients. J Proteome Res. 2012; 11:5611–5629.


80. Mitchell PS, Parkin RK, Kroh EM, Fritz BR, Wyman SK, Pogosova-Agadjanyan EL, Peterson A, Noteboom J, O'Briant KC, Allen A, Lin DW, Urban N, Drescher CW, Knudsen BS, Stirewalt DL, Gentleman R, Vessella RL, Nelson PS, Martin DB, Tewari M. Circulating microRNAs as stable blood-based markers for cancer detection. Proc Natl Acad Sci U S A. 2008; 105:10513–10518.


81. Brase JC, Johannes M, Schlomm T, Fälth M, Haese A, Steuber T, Beissbarth T, Kuner R, Sültmann H. Circulating miRNAs are correlated with tumor progression in prostate cancer. Int J Cancer. 2011; 128:608–616.


82. Hunter MP, Ismail N, Zhang X, Aguda BD, Lee EJ, Yu L, Xiao T, Schafer J, Lee ML, Schmittgen TD, Nana-Sinkam SP, Jarjoura D, Marsh CB. Detection of microRNA expression in human peripheral blood microvesicles. PLoS One. 2008; 3:e3694.


83. Lin J, Li J, Huang B, Liu J, Chen X, Chen XM, Xu YM, Huang LF, Wang XZ. Exosomes: novel biomarkers for clinical diagnosis. ScientificWorldJournal. 2015; 2015.


84. Nilsson J, Skog J, Nordstrand A, Baranov V, Mincheva-Nilsson L, Breakefield XO, Widmark A. Prostate cancer-derived urine exosomes: a novel approach to biomarkers for prostate cancer. Br J Cancer. 2009; 100:1603–1607.


85. Tanaka Y, Kamohara H, Kinoshita K, Kurashige J, Ishimoto T, Iwatsuki M, Watanabe M, Baba H. Clinical impact of serum exosomal microRNA-21 as a clinical biomarker in human esophageal squamous cell carcinoma. Cancer. 2013; 119:1159–1167.


86. Takeshita N, Hoshino I, Mori M, Akutsu Y, Hanari N, Yoneyama Y, Ikeda N, Isozaki Y, Maruyama T, Akanuma N, Komatsu A, Jitsukawa M, Matsubara H. Serum microRNA expression profile: miR-1246 as a novel diagnostic and prognostic biomarker for oesophageal squamous cell carcinoma. Br J Cancer. 2013; 108:644–652.


87. Kuwabara Y, Ono K, Horie T, Nishi H, Nagao K, Kinoshita M, Watanabe S, Baba O, Kojima Y, Shizuta S, Imai M, Tamura T, Kita T, Kimura T. Increased microRNA-1 and microRNA-133a levels in serum of patients with cardiovascular disease indicate myocardial damage. Circ Cardiovasc Genet. 2011; 4:446–454.


88. Lv LL, Cao YH, Ni HF, Xu M, Liu D, Liu H, Chen PS, Liu BC. MicroRNA-29c in urinary exosome/microvesicle as a biomarker of renal fibrosis. Am J Physiol Renal Physiol. 2013; 305:F1220–F1227.


89. Zhou H, Yuen PS, Pisitkun T, Gonzales PA, Yasuda H, Dear JW, Gross P, Knepper MA, Star RA. Collection, storage, preservation, and normalization of human urinary exosomes for biomarker discovery. Kidney Int. 2006; 69:1471–1476.


90. Zhou H, Xu M, Huang Q, Gates AT, Zhang XD, Castle JC, Stec E, Ferrer M, Strulovici B, Hazuda DJ, Espeseth AS. Genome-scale RNAi screen for host factors required for HIV replication. Cell Host Microbe. 2008; 4:495–504.


91. Takata K, Matsuzaki T, Tajika Y, Ablimit A, Hasegawa T. Localization and trafficking of aquaporin 2 in the kidney. Histochem Cell Biol. 2008; 130:197–209.


92. Palanisamy V, Sharma S, Deshpande A, Zhou H, Gimzewski J, Wong DT. Nanostructural and transcriptomic analyses of human saliva derived exosomes. PLoS One. 2010; 5:e8577.


93. Baglio SR, Pegtel DM, Baldini N. Mesenchymal stem cell secreted vesicles provide novel opportunities in (stem) cell-free therapy. Front Physiol. 2012; 3:359.


94. Chen TS, Yeo RWY, Arslan F, Yin Y, Tan SS, Lai RC, Choo A, Padmanabhan J, Lee CN, de Kleijn DPV, Tan KH, Lim SK. Efficiency of exosome production correlates inversely with the developmental maturity of MSC donor. J Stem Cell Res Ther. 2013; 3:145.


95. André F, Chaput N, Schartz NE, Flament C, Aubert N, Bernard J, Lemonnier F, Raposo G, Escudier B, Hsu DH, Tursz T, Amigorena S, Angevin E, Zitvogel L. Exosomes as potent cell-free peptide-based vaccine. I. Dendritic cell-derived exosomes transfer functional MHC class I/peptide complexes to dendritic cells. J Immunol. 2004; 172:2126–2136.


96. Hsu DH, Paz P, Villaflor G, Rivas A, Mehta-Damani A, Angevin E, Zitvogel L, Le Pecq JB. Exosomes as a tumor vaccine: enhancing potency through direct loading of antigenic peptides. J Immunother. 2003; 26:440–450.


97. Chaput N, Schartz NE, André F, Taïeb J, Novault S, Bonnaventure P, Aubert N, Bernard J, Adema G, Adams M, Ferrantini M, Carpentier AF, Escudier B, Tursz T, Angevin E, Zitvogel L. Exosomes as potent cell-free peptide-based vaccine. II. Exosomes in CpG adjuvants efficiently prime naive Tc1 lymphocytes leading to tumor rejection. J Immunol. 2004; 172:2137–2146.


98. Lee C, Mitsialis SA, Aslam M, Vitali SH, Vergadi E, Konstantinou G, Sdrimas K, Fernandez-Gonzalez A, Kourembanas S. Exosomes mediate the cytoprotective action of mesenchymal stromal cells on hypoxia-induced pulmonary hypertension. Circulation. 2012; 126:2601–2611.


99. Doeppner TR, Herz J, Görgens A, Schlechter J, Ludwig AK, Radtke S, de Miroschedji K, Horn PA, Giebel B, Hermann DM. Extracellular vesicles improve post-stroke neuroregeneration and prevent postischemic immunosuppression. Stem Cells Transl Med. 2015; 4:1131–1143.


100. Zhang Y, Chopp M, Meng Y, Katakowski M, Xin H, Mahmood A, Xiong Y. Effect of exosomes derived from multipluripotent mesenchymal stromal cells on functional recovery and neurovascular plasticity in rats after traumatic brain injury. J Neurosurg. 2015; 122:856–867.


101. Xin H, Li Y, Buller B, Katakowski M, Zhang Y, Wang X, Shang X, Zhang ZG, Chopp M. Exosome-mediated transfer of miR-133b from multipotent mesenchymal stromal cells to neural cells contributes to neurite outgrowth. Stem Cells. 2012; 30:1556–1564.


102. Bruno S, Grange C, Deregibus MC, Calogero RA, Saviozzi S, Collino F, Morando L, Busca A, Falda M, Bussolati B, Tetta C, Camussi G. Mesenchymal stem cell-derived microvesicles protect against acute tubular injury. J Am Soc Nephrol. 2009; 20:1053–1067.


103. Li T, Zhu J, Ma K, Liu N, Feng K, Li X, Wang S, Bie P. Autologous bone marrow-derived mesenchymal stem cell transplantation promotes liver regeneration after portal vein embolization in cirrhotic rats. J Surg Res. 2013; 184:1161–1173.


104. Lee JK, Park SR, Jung BK, Jeon YK, Lee YS, Kim MK, Kim YG, Jang JY, Kim CW. Exosomes derived from mesenchymal stem cells suppress angiogenesis by down-regulating VEGF expression in breast cancer cells. PLoS One. 2013; 8:e84256.


105. Yang Y, Bucan V, Baehre H, von der, Otte A, Hass R. Acquisition of new tumor cell properties by MSC-derived exosomes. Int J Oncol. 2015; 47:244–252.


106. Kordelas L, Rebmann V, Ludwig AK, Radtke S, Ruesing J, Doeppner TR, Epple M, Horn PA, Beelen DW, Giebel B. MSC-derived exosomes: a novel tool to treat therapy-refractory graft-versus-host disease. Leukemia. 2014; 28:970–973.


107. Rahman MJ, Regn D, Bashratyan R, Dai YD. Exosomes released by islet-derived mesenchymal stem cells trigger autoimmune responses in NOD mice. Diabetes. 2014; 63:1008–1020.


108. Zöller M. Tetraspanins: push and pull in suppressing and promoting metastasis. Nat Rev Cancer. 2009; 9:40–55.


109. Pap E, Pállinger E, Pásztói M, Falus A. Highlights of a new type of intercellular communication: microvesicle-based information transfer. Inflamm Res. 2009; 58:1–8.


110. Taylor DD, Gercel-Taylor C. Exosomes/microvesicles: mediators of cancer-associated immunosuppressive microenvironments. Semin Immunopathol. 2011; 33:441–454.


111. Kantoff PW, Higano CS, Shore ND, Berger ER, Small EJ, Penson DF, Redfern CH, Ferrari AC, Dreicer R, Sims RB, Xu Y, Frohlich MW, Schellhammer PF. IMPACT Study Investigators. Sipuleucel-T immunotherapy for castration-resistant prostate cancer. N Engl J Med. 2010; 363:411–422.


112. Besse B, Charrier M, Lapierre V, Dansin E, Lantz O, Planchard D, Le Chevalier T, Livartoski A, Barlesi F, Laplanche A, Ploix S, Vimond N, Peguillet I, Théry C, Lacroix L, Zoernig I, Dhodapkar K, Dhodapkar M, Viaud S, Soria JC, Reiners KS, Pogge von, Vély F, Rusakiewicz S, Eggermont A, Pitt JM, Zitvogel L, Chaput N. Dendritic cell-derived exosomes as maintenance immunotherapy after first line chemotherapy in NSCLC. Oncoimmunology. 2015; 5:e1071008.


113. Pignon JP, Tribodet H, Scagliotti GV, Douillard JY, Shepherd FA, Stephens RJ, Dunant A, Torri V, Rosell R, Seymour L, Spiro SG, Rolland E, Fossati R, Aubert D, Ding K, Waller D, Le Chevalier T. LACE Collaborative Group. Lung adjuvant cisplatin evaluation: a pooled analysis by the LACE Collaborative Group. J Clin Oncol. 2008; 26:3552–3559.


114. Polo V, Garcia-Martin E, Bambo MP, Pinilla J, Larrosa JM, Satue M, Otin S, Pablo LE. Reliability and validity of Cirrus and Spectralis optical coherence tomography for detecting retinal atrophy in Alzheimer's disease. Eye (Lond). 2014; 28:680–690.


115. Segura E, Nicco C, Lombard B, Véron P, Raposo G, Batteux F, Amigorena S, Théry C. ICAM-1 on exosomes from mature dendritic cells is critical for efficient naive T-cell priming. Blood. 2005; 106:216–223.


116. Utsugi-Kobukai S, Fujimaki H, Hotta C, Nakazawa M, Minami M. MHC class I-mediated exogenous antigen presentation by exosomes secreted from immature and mature bone marrow derived dendritic cells. Immunol Lett. 2003; 89:125–131.


117. Viaud S, Ploix S, Lapierre V, Théry C, Commere PH, Tramalloni D, Gorrichon K, Virault-Rocroy P, Tursz T, Lantz O, Zitvogel L, Chaput N. Updated technology to produce highly immunogenic dendritic cell-derived exosomes of clinical grade: a critical role of interferon-γ. J Immunother. 2011; 34:65–75.
118. Gutiérrez-Vázquez C, Villarroya-Beltri C, Mittelbrunn M, Sánchez-Madrid F. Transfer of extracellular vesicles during immune cell-cell interactions. Immunol Rev. 2013; 251:125–142.


119. Hedlund M, Nagaeva O, Kargl D, Baranov V, Mincheva-Nilsson L. Thermal- and oxidative stress causes enhanced release of NKG2D ligand-bearing immunosuppressive exosomes in leukemia/lymphoma T and B cells. PLoS One. 2011; 6:e16899.


120. Biasutto L, Chiechi A, Couch R, Liotta LA, Espina V. Retinal pigment epithelium (RPE) exosomes contain signaling phosphoproteins affected by oxidative stress. Exp Cell Res. 2013; 319:2113–2123.


121. Eldh M, Ekström K, Valadi H, Sjöstrand M, Olsson B, Jernås M, Lötvall J. Exosomes communicate protective messages during oxidative stress; possible role of exosomal shuttle RNA. PLoS One. 2010; 5:e15353.


122. Zhang Y, Luo CL, He BC, Zhang JM, Cheng G, Wu XH. Exosomes derived from IL-12-anchored renal cancer cells increase induction of specific antitumor response in vitro: a novel vaccine for renal cell carcinoma. Int J Oncol. 2010; 36:133–140.


123. Alvarez-Erviti L, Seow Y, Yin H, Betts C, Lakhal S, Wood MJ. Delivery of siRNA to the mouse brain by systemic injection of targeted exosomes. Nat Biotechnol. 2011; 29:341–345.


124. Théry C, Ostrowski M, Segura E. Membrane vesicles as conveyors of immune responses. Nat Rev Immunol. 2009; 9:581–593.


126. Zhuang X, Xiang X, Grizzle W, Sun D, Zhang S, Axtell RC, Ju S, Mu J, Zhang L, Steinman L, Miller D, Zhang HG. Treatment of brain inflammatory diseases by delivering exosome encapsulated anti-inflammatory drugs from the nasal region to the brain. Mol Ther. 2011; 19:1769–1779.


127. Sun D, Zhuang X, Xiang X, Liu Y, Zhang S, Liu C, Barnes S, Grizzle W, Miller D, Zhang HG. A novel nanoparticle drug delivery system: the anti-inflammatory activity of curcumin is enhanced when encapsulated in exosomes. Mol Ther. 2010; 18:1606–1614.

