Abstract
Paclitaxel, a chemotherapeutic drug, induces severe peripheral neuropathy. Gabapentin (GBT) is a first line agent used to treat neuropathic pain, and its effect is mediated by spinal noradrenergic and muscarinic cholinergic receptors. Electro-acupuncture (EA) is used for treating various types of pain via its action through spinal opioidergic and noradrenergic receptors. Here, we investigated whether combined treatment of these two agents could exert a synergistic effect on paclitaxel-induced cold and mechanical allodynia, which were assessed by the acetone drop test and von Frey filament assay, respectively. Significant signs of allodynia were observed after four paclitaxel injections (a cumulative dose of 8 mg/kg, i.p.). GBT (3, 30, and 100 mg/kg, i.p.) or EA (ST36, Zusanli) alone produced dose-dependent anti-allodynic effects. The medium and highest doses of GBT (30 and 100 mg/kg) provided a strong analgesic effect, but they induced motor dysfunction in Rota-rod tests. On the contrary, the lowest dose of GBT (3 mg/kg) did not induce motor weakness, but it provided a brief analgesic effect. The combination of the lowest dose of GBT and EA resulted in a greater and longer effect, without inducing motor dysfunction. This effect on mechanical allodynia was blocked by spinal opioidergic (naloxone, 20 μg), or noradrenergic (idazoxan, 10 μg) receptor antagonist, whereas on cold allodynia, only opioidergic receptor antagonist blocked the effect. In conclusion, the combination of the lowest dose of GBT and EA has a robust and enduring analgesic action against paclitaxel-induced neuropathic pain, and it should be considered as an alternative treatment method.
Paclitaxel is a lipophilic molecule derived from the Pacific yew tree, Taxus brevifolia , and it is a widely used chemotherapeutic agent for treating a broad range of cancers such as ovarian, breast, and lung cancers [123]. It promotes the death of tumor cells by disrupting the normal microtubule dynamics, which is important for cellular division, motility, and activation [4]. Although it has efficacy against tumors, adverse effects reported in patients treated with paclitaxel are dose-limiting factors that can even lead to discontinuation of treatment [56]. One of the major side effects reported is peripheral sensory neurotoxicity, and about 60% of patients treated with paclitaxel develop this side effect [7]. Peripheral neurotoxicity is characterized by sensory symptoms such as cold and mechanical allodynia, and paresthesia in a glove-and-stocking distribution [8]. These symptoms are symmetrical and may begin as early as 24 to 72 hours after treatment with higher doses of paclitaxel, but they usually occur only after multiple courses of paclitaxel at conventional doses [910].
Gabapentin (GBT) is an anti-epileptic drug that has structural analogy to gamma-aminobutyric acid GABA, and it is referred to as a first line agent for the treatment of neuropathic pain [11] which possesses strong analgesic activity against diverse neuropathic pain disorders in humans [121314] as well as in rodents [1516]. The most important mechanism explaining the analgesic effect of GBT is probably the blockage of calcium influx into neurons. By blocking calcium influx, GBT reduces synaptic release of excitatory neurotransmitters and postsynaptic neuronal excitation in the brain and in the spinal cord [1718]. At the spinal level, GBT has been reported to act through the α2-adrenoceptor or muscarinic cholinergic receptors [192021]. However, despite its analgesic effect, GBT also induces side effects such as somnolence, nausea, and vertigo, which prevent its wide use [22].
Acupuncture has been proven to be clinically efficacious in reducing various types of pain, and electro-acupuncture (EA) is a procedure that combines acupuncture with electric current stimulation. The analgesic effect of EA on different types of pain has been reported in humans and rodents [2324]. Studies performed in animals have shown that EA significantly alleviates behavioral signs such as hyperalgesia and allodynia in peripheral nerve injury-induced neuropathic pain models [252627]. Also, in chemotherapy-induced peripheral neuropathy (CIPN), results show that EA can relieve the symptoms induced by different chemotherapeutic agents [2829]. Over the past several years, our laboratory has demonstrated that EA can significantly attenuate nerve injury [25] and chemotherapy [3031] induced neuropathic pain. We have also clarified that EA reduces pain through the descending inhibitory system that involves spinal opioidergic [32], α2-adrenergic [25], and muscarinic cholinergic receptors [2733]. In a previously published article by other laboratory, EA also attenuated allodynia via the mediation of spinal opioid and α2-drenergic receptors in paclitaxel-induced neuropathic pain in rats [34].
Thus, in this article, we hypothesized that co-administration of GBT and EA could produce increased analgesic efficacy without causing any adverse effects. To test this hypothesis, we firstly investigated the analgesic effect of different doses of GBT or EA administered separately. Secondly, we assessed whether combined treatment with these two mechanistically distinct analgesic treatment agents could produce improved analgesic efficacy compared to the use of a single agent alone. Finally, we determined which spinal receptors were involved in mediating the effects of combined GBT and EA treatment.
Young male adult C57BL/6 mice (Daehan Biolink, Chungbuk, Korea) of 6~8 weeks of age were housed in plastic cages with standard rodent food and water available ad libitum. Mice were retained in a 12 h-light/dark cycle with 60~70% relative humidity and temperature maintained at 23±2℃. Animals were adapted for seven days prior to all procedures. The animal studies were performed after receiving approval from the Institutional Animal Care and Use Committee (IACUC) of Kyung Hee University (KHUASP(SE)-16-153).
Paclitaxel administration produced neuropathic pain characterized by cold and mechanical allodynia in mice. Paclitaxel (Sigma Chemical Co., U.S.A.) was dissolved in 50% Ethanol and 50% Cremophor EL solution to a concentration of 6 mg/ml, and then, it was diluted in phosphate buffered saline (PBS) to obtain a target concentration of 0.2 mg/ml. Paclitaxel (2 mg/kg) or vehicle was injected intraperitoneally (i.p.) every other day to a total of four injections (days 0, 2, 4, 6). The accumulated concentration of paclitaxel was 8 mg/kg [35].
To determine whether cold and mechanical allodynia were induced in animals, acetone drop tests and von Frey filament assays were used, respectively [36]. Before starting behavioral tests, every mouse was adapted to the cage and to all testing procedures for seven days. Mice were placed on a stainless steel mesh floor with an acrylic cover (12×8×6 cm) and were adapted for 30 min prior to each test. Experimenters who were blinded to the treatments evaluated the behavioral tests.
To measure cold sensitivity, 10 µl of acetone (Daejung Chemical Ltd., Gyeonggi-do, Korea) was applied three times at the center of the plantar surface of each hind paw. After acetone was sprayed, the number of responses (withdrawal or flick, licking or shaking of the paw) was counted for 30 s [37]. The average number of responses was calculated using six scores obtained from each mouse.
Mechanical sensitivity was evaluated by the number of hind paw withdrawal responses to the von Frey filament stimulation (bending force: 0.4 g, Linton Instrumentation, Norfolk, UK) [38]. Each hind paw was poked 10 times on the mid plantar surface and each application was presented for approximately 3 s [39]. Withdrawal responses in the von Frey filament assay were obtained from both hind paws and were calculated as an overall percentage response (number of responses/20×100).
Rota-rod test was performed to assess the adverse effect of GBT at different doses and when it is combined with EA. A Rotarod treadmill device was purchased from Ugo Basile (S.R.L., VA, Italy). One hour after the injection of GBT or EA stimulation, mice of all groups were placed on a rotating drum revolving at a constant speed of 20 revolutions per minute [40]. Before the test, animals were trained to adapt to the horizontal revolving rod. Only those rodents that were able to remain on the horizontal revolving rod for more than 300 s were used. The fall-off time at which each mouse fell from the rotating rod device was noted, and the cut-off time was set at 300 s, as used in other studies [41].
GBT was purchased from Sigma Chemical Co., USA, and it was dissolved in PBS. Six to eight mice were used in each GBT group (3, 30, and 100 mg/kg, i.p.) [4243]. In the control group, four mice received the same volume of PBS, intraperitoneally. Acupoint Zusanli (ST36) was used for EA or manual acupuncture (MA) [44]. For EA stimulation at acupoint ST36, two acupuncture needles were inserted: one into the right side ST36 and another one 5 mm distal to ST36 (5 mm in depth). The tip of the electrode cables from an electrical stimulator (Nihon Kohden, Japan) was connected to a pair of steel acupuncture needles, and constant rectangular current pulses (2 Hz, 0.3-ms pulse duration) were applied for 20 min. For MA at ST36, an acupuncture needle was inserted into right side ST36, the same point as that for EA, for 20 min without electrical stimulation. EA and MA procedures was performed under isoflurane anesthesia [30]. Combination treatment with GBT and EA was performed to assess the possible synergistic effect. Along with EA or MA at ST36, mice received GBT (3 mg/kg) or the same volume of PBS, intraperitoneally.
To investigate the analgesic action of GBT and EA at the spinal level, the opioid receptor antagonist naloxone (Sigma; 20 µg), the selective α2-adrenergic receptor antagonist idazoxan (Sigma; 10 µg), the non-selective muscarinic cholinergic receptor antagonist atropine (Sigma; 0.1 µg), or PBS was injected intrathecally in a volume of 5 µl with direct lumbar puncture under isoflurane anesthesia [2145]. A Hamilton syringe needle was used to inject antagonists into the subarachnoid space between L5 and L6. The antagonists were dissolved in PBS and were injected 20 min before GBT and EA administration.
Multiple injections (days 0, 2, 4, 6) of paclitaxel (2 mg/kg, i.p.) produced cold and mechanical allodynia in C57BL/6 mice (Fig. 1). Vehicle (1:1 cremophor EL and EtOH diluted in PBS) was administered intraperitoneally as control. Cold and mechanical allodynia were assessed by the acetone drop test (10 µl) and von Frey filament assay (0.4 g bending force), respectively. Significant cold and mechanical allodynia signs were observable from day 7 to 14 (Fig. 1). On day 17, cold allodynia decreased to the control level (Fig. 1a), whereas mechanical allodynia lasted longer, up to day 17 (Fig. 1b). Vehicle had no effect on cold and mechanical sensitivity.
The elapsed time of the anti-allodynic effect of different doses of GBT was assessed (Fig. 2). Mice were divided into four groups (PBS, 3, 30, and 100 mg/kg GBT), and responses to the acetone drop test and von Frey filament assay were recorded before the injection of paclitaxel (baseline) and after the injection of paclitaxel (time point zero). GBT was administered intraperitoneally at three different doses (3, 30, and 100 mg/kg), and PBS (i.p.) was used as control. All assessments for observing the elapsed time effect of different doses of GBT were conducted between days 7 and 14, when significant cold and mechanical allodynia were present in mice (Fig. 1). On the day of the evaluation, behavioral tests were conducted at time point zero (before the administration of GBT or vehicle), and at 60, 120, and 180 min after the initiation of treatment. The medium and highest doses of GBT (30 and 100 mg/kg) produced relieving effects, which lasted until 180 min after the injection, on both cold and mechanical allodynia (Fig. 2a, b). However, the lowest dose of GBT (3 mg/kg) produced an analgesic effect on cold allodynia that lasted only for 60 min after the treatment (Fig. 2a), whereas the analgesic effect was not observed on mechanical allodynia (Fig. 2b).
To evaluate the analgesic effect of EA and MA, mice were divided into three groups; control, EA, and MA. All three groups of mice exhibited cold and mechanical allodynia after paclitaxel injection. Mice in the control group did not undergo any further treatment. Both EA and MA were administered at ST36 acupoint for 20 min. Constant rectangular current pulses (2 Hz, 0.3-ms pulse duration) were applied for EA, whereas electrical stimulation was not applied for MA. Compared to the control, EA produced a stronger anti-allodynic effect than MA on both cold and mechanical allodynia (Fig. 3a, b). Although MA produced relieving effects, when this group was compared to the control group, the effect was not statistically significant. The analgesic effects of EA were stronger at 60 and 120 min after administration, but the effect on both cold and mechanical allodynia disappeared after 180 min.
The combined effects of GBT and EA on paclitaxel-induced cold and mechanical allodynia in mice are shown in Fig. 4. The lowest dose of GBT (3 mg/kg, i.p.) and EA (ST36) were administered simultaneously. The anti-allodynic effect of combined application of GBT and EA was stronger and it lasted longer than the effect of the combination of GBT with MA or PBS with EA (GBT3+MA and PBS+EA). In the acetone drop test, the combination of PBS with EA produced a strong analgesic effect after 60 and 120 min of treatment, but the effect did not last up to 180 min, whereas the combination of GBT with EA produced an effect that lasted up to 180 min after the administration (Fig. 4a). In the von Frey filament assay also, neither the effect of the combination of GBT with MA nor the effect of the combination of PBS with EA was observed within 120 min, whereas the combination of GBT with EA produced an analgesic effect that lasted until 180 min (Fig. 4b).
To assess whether mice treated with three different doses of GBT or GBT with EA show irregular motor function, Rota-rod tests were conducted. Mice displaying cold and mechanical allodynia were divided into five groups (PBS, GBT 3 mg/kg, GBT 30 mg/kg, GBT 100 mg/kg, and GBT 3 mg/kg+EA). A Rota-rod test was performed under the condition of 20 rpm speed with a 300 second cut-off time. Paclitaxel injections were not found to result in motor dysfunction. The medium and highest doses of GBT induced dose-dependent dysfunction (Fig. 5). However, the lowest dose of GBT or the combination of GBT with EA did not induce any motor dysfunction, thus demonstrating that the combination of GBT with EA did not induce any motor weakness.
To clarify the analgesic effect of combined GBT and EA administration at the spinal cord level, the opioidergic (naloxone), α2-adrenergic (idazoxan), or muscarinic cholinergic (atropine) receptor antagonist was injected intrathecally 20 min before the treatment. Naloxone, idazoxan, or atropine was injected in a volume of 5 µl, and the same volume of PBS was used as control.
In cold allodynia, naloxone, but not idazoxan and atropine, block the anti-allodynic effect of GBT and EA, showing that GBT and EA analgesic effect is mediated only by spinal opioidergic receptors. However, in mechanical allodynia, the effect was blocked by naloxone and idazoxan, but not atropine, stating that GBT and EA act via spinal opioidergic and/or α2-adrenergic, but not muscarinic cholinergic receptors on mechanical allodynia.
Paclitaxel-induced peripheral neuropathy is a serious side effect that requires interruption of treatment of tumors. Various agents are used to reduce this adverse effect; however, conventional analgesic drugs, such as non-steroidal anti-inflammatory drugs (NSAIDs) and antidepressants, have a poor action or induce side effects, which can lead to discontinuation of treatments [46]. Thus, an alternative treatment method is needed to reduce the side effect of paclitaxel [47].
Experimental and clinical studies have reported that GBT is effective in relieving symptoms associated with acute and chronic neuropathy [484950]. Furthermore, it was shown to be effective in peripheral neuropathy induced by various chemotherapeutic agents such as oxaliplatin [51], vincristine [52], and paclitaxel [53]. However, when GBT is used at high doses, despite its analgesic effect, side effects such as sedation and vertigo led to discontinuation of treatment [22]. To reduce these side effects and to increase the analgesic effect, an effort to combine GBT with other analgesic drugs or treatment methods has been made. Specifically, many studies have reported its use with morphine [6], as GBT was reported to act via the spinal noradrenergic and muscarinic cholinergic system, whereas morphine acts as a opioid receptor agonist. Indeed, GBT, when used with morphine, was shown to increase pain tolerance threshold of cold stimulation [54], and it also produced better analgesia in patients suffering from neuropathic cancer pain than when used alone [5556].
EA is a treatment method that has a similar spinal mechanism as morphine, and in our previous experiments conducted in oxaliplatin-induced neuropathic pain in rats, EA was shown to produce a somewhat similar analgesic effect as morphine [31]. Furthermore, the opioidergic pathway was involved in the action of EA, as the opioidergic receptor antagonist completely blocked the analgesic effect of EA on oxaliplatin-induced neuropathic pain in rats. As mentioned earlier, EA also attenuated paclitaxel-induced mechanical allodynia and thermal hyperalgesia via the mediation of spinal opioid and α2-adrenergic receptors [34].
Thus, in this article, we hypothesized that simultaneous injection of GBT, which acts via spinal α2-adrenergic and muscarinic receptors, and EA, which acts through opioidergic and α2-adrenergic receptors, may produce a synergistic effect without causing significant side effects.
To determine whether this hypothesis is true, we firstly, observed the elapsed time effect of three different doses of GBT (3, 30, and 100 mg/kg) on paclitaxel-induced cold and mechanical allodynia. Our results demonstrated that the medium and highest doses (30 and 100 mg/kg) of GBT provided a significant analgesic effect compare to the control. The lowest dose (3 mg/kg) of GBT had no effect on cold allodynia and it had only a small effect that lasted for 60 min on mechanical allodynia (Fig. 2). However, the medium and highest doses of GBT induced motor dysfunction in Rota-rod tests, whereas the lowest dose-treated mice did not show any motor weakness (Fig. 5).
Following the experience with GBT, we checked whether EA at ST36 acupoint can alleviate cold and mechanical allodynia induced by paclitaxel injection, and we found that it exerted a greater analgesic effect than MA performed at the same site (Fig. 3). The analgesic effect of EA lasted until 120 min, whereas mice treated with MA showed no significant analgesic effect. ST36 is an acupoint generally used in pain conditions including neuropathic pain, and our laboratory has proved that acupuncture at ST36 has a stronger analgesic effect than that at any other acupoints based on studies conducted using different animal models [253057]. In addition, EA at ST36 also produced a strong analgesic effect on paclitaxel-induced neuropathic pain [34].
In our subsequent behavioral tests, we found that combined treatment with the lowest dose of GBT and EA had an analgesic effect that lasted longer (up to 180 min) and it was stronger than that of GBT combined with MA or EA combined with PBS (Fig. 4). Furthermore, using Rota-rod test, we found that combination treatment with GBT and EA did not induce any motor weakness, as no significant difference was found between the GBT and EA combination group and the control group.
Finally, to determine the spinal mechanism of the analgesic action of the combination of GBT and EA, we injected opioidergic, adrenergic, and muscarinic cholinergic receptor antagonists before treatment with GBT and EA. The results reported in Fig. 6 show that only the opioidergic receptor antagonist blocked the analgesic effect of the combination of GBT and EA on cold allodynia, whereas, both opioidergic and adrenergic receptor antagonists blocked the effect of the combination of GBT and EA on mechanical allodynia. Although we cannot clearly explain all the details of the process, this discrepancy may have resulted from the difference in the mechanism of cold and mechanical allodynia. In several experimental studies, cold allodynia is shown to be mediated mostly by C fibers [585960], and at the spinal cord level, the majority of opioid receptors are reported to be located on the presynaptic terminals of small unmyelinated C fibers than on those of large myelinated A primary afferent fibers [61]. This may explain why only naloxone blocked the analgesic effect of the combination of GBT and EA on cold allodynia, as mechanical allodynia is mediated by both A and C fibers [62], and intrathecally administered α2-adrenergic agonist was reported to decrease only mechanical allodynia but not cold allodynia in a rat model of neuropathic pain [63]. However, in both cold and mechanical allodynia, the interaction of opioidergic and noradrenergic systems should not be ignored as their co-administration often leads to a synergistic effect, which may originate from the brain as GBT and EA are both known to act on different part of the brain involved in the descending pain inhibitory system. GBT was reported to decrease pain by activating locus coeruleus (LC), which is the largest group of noradrenergic neurons in the central nervous system (CNS) [64], and EA by activating both periaqueductal grey (PAG) [65], an abundant endogenous opioid peptide synthetization site, and LC [66]. In our experiments, the simultaneous activation of PAG and LC induced by both GBT and EA may enhance the opioidergic and noradrenergic descending inhibitory pathway, as activation of both brain part resulted in better analgesia than the stimulation of each site alone [67]. Moreover, although atropine did not block the effect of GBT and EA, the cholinergic system may also have played a role in this synergistic process by potentiating the effect of the opioidergic pathway in the brain, as the effect of microinjection of morphine and carbachol (the opioid receptor agonist and cholinergic receptor agonist, respectively) in the central nucleus of amygdala was blocked by naloxone pretreatment [68]. Moreover, the effect of co-administration of GBT with the opioid receptor agonist was also blocked by intrathecal naloxone pretreatment in a rat model of pain [69]. All these results let us speculate that the interaction of opioidergic, noradrenergic, and cholinergic system in the CNS could have led to a synergistic action of GBT and EA.
As mentioned above, in our experiments, atropine did not block the pain attenuating effect of GBT and EA, whereas, atropine blocked the effect of separately administered GBT [70] and EA [30] in other experiments conducted by others and our own laboratory, suggesting that different mechanism than that of the monotherapy may be involved in the analgesic mechanism of GBT and EA.
In conclusion, simultaneous treatment with the combination of the lowest dose of GBT (3 mg/kg) and EA at ST36 significantly attenuated cold and mechanical allodynia induced by four intraperitoneal injections of paclitaxel in mice. This combination of GBT and EA produced a greater and longer effect than GBT or EA alone, and this analgesic effect was mediated by spinal opioidergic and/or α2-adrenergic receptors. These results suggest that combination treatment with GBT and EA could be used as an alternative method for reducing paclitaxel-induced neuropathic pain.
Figures and Tables
Fig. 1
Elapsed time of cold and mechanical allodynia induced by paclitaxel injections.
Behavioral tests for cold and mechanical allodynia were performed before (time point zero) and after four injections of 2 mg/kg paclitaxel (days 0, 2, 4, and 6, i.p., n=10). Also, 1:1 cremophor EL and EtOH diluted in PBS was used as the vehicle (i.p., n=9). Cold (a) and mechanical (b) allodynia assessments were made by the acetone drop test and von Frey filament assay, respectively. Data is presented as mean±S.E.M.; ***p<0.001 vs. vehicle, by Two-way ANOVA.
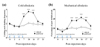
Fig. 2
Elapsed time of the dose-dependent effect of GBT on paclitaxel-induced cold and mechanical allodynia in mice.
Mice with paclitaxel-induced cold and mechanical allodynia were divided into four groups: PBS (PBS, n=6), GBT 3 mg/kg (GBT3, n=7), GBT 30 mg/kg (GBT30, n=7), and GBT 100 mg/kg (GBT100, n=6). PBS was used as control and both PBS and GBT were administered intraperitoneally. (a) Elapsed time and dose-dependent effect of GBT on cold allodynia. (b) Elapsed time and the dose-dependent effect of GBT on mechanical allodynia. Bl refers to baseline and represent data for behavioral assessments before the injection of paclitaxel. Data is presented as mean±S.E.M.; **p<0.01, ***p<0.001 vs. PBS; by Two-way ANOVA.
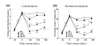
Fig. 3
Elapsed time of the effect of EA and MA on paclitaxel-induced cold and mechanical allodynia in mice.
Mice with paclitaxel-induced cold and mechanical allodynia were divided into three groups: CONT (control, n=4), MA (manual acupuncture, ST 36, n=6), EA (electro-acupuncture, ST 36, n=6). (a) Elapsed time of the analgesic effect of EA and MA in cold allodynia-induced mice. (b) Elapsed time of the analgesic effect of EA and MA in mechanical allodynia-induced mice. Bl refers to baseline. Data is presented as mean±S.E.M.; **p<0.01, ***p<0.001 vs. CONT; by Two-way ANOVA.

Fig. 4
Elapsed time of the synergistic effect of the lowest dose of GBT and EA at ST36.
Mice with paclitaxel-induced cold and mechanical allodynia were divided into four groups: CONT (control, n=6), GBT3+MA (GBT 3 mg/kg+MA, n=6), PBS+EA (n=6), GBT3+EA (GBT 3 mg/kg+EA, n=6). Elapsed time of the effect of combination treatment with GBT and EA was observed in both (a) cold and (b) mechanical allodynia induced by paclitaxel. Bl refers to baseline. Data is presented as mean±S.E.M.; *p<0.05, **p<0.01, ***p<0.001 vs. CONT; by Two-way ANOVA.
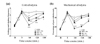
Fig. 5
Rota-rod test performed in GBT and EA treated mice.
Paclitaxel-induced cold and mechanical allodynic mice were divided into five groups for performing Rota-rod test: PBS (i.p., n=6), GBT3 (GBT 3 mg/kg, i.p., n=8), GBT30 (GBT 30 mg/kg, i.p., n=8), GBT100 (GBT 100 mg/kg, i.p., n=7), and GBT3+EA (GBT 3 mg/kg (i.p.)+EA (ST36), n=6). Rota-rod tests were performed under the condition of 20 rpm speed with a 300 second cut-off time. PBS was used as control. Data is presented as mean±S.E.M.; **p<0.01, ***p<0.001 vs. PBS; by paired t-test.
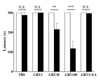
Fig. 6
Analgesic mechanism at the spinal level due to the synergistic effect of GBT and EA on paclitaxel-induced allodynia in mice.
(a) Effect of naloxone, idazoxan, atropine, and PBS pretreatment on the analgesic effect of GBT and EA on paclitaxel-induced cold allodynia. (b) Effect of naloxone, idazoxan, atropine, and PBS pretreatment on the analgesic effect of GBT and EA on paclitaxel-induced mechanical allodynia. Mice with paclitaxel-induced cold and mechanical allodynia were divided into four groups: PBS (i.t., n=6), Naloxone (20 µg, i.t., n=6), Idazoxan (10 µg, i.t., n=6), and Atropine (0.1 µg , i.t., n=5). Antagonists were injected 20 min prior to GBT and EA treatment, and all behavioral assessments were made 60 min after GBT and EA treatment. Data is presented as mean±S.E.M.; **p<0.01, ***p<0.001 vs. PBS; by paired t-test.
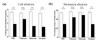
ACKNOWLEDGEMENTS
This work was supported by a grant from Kyung Hee University in 2016 (KHU-20160548) and by a Basic Science Research Program through the National Research Foundation of Korea (NRF) funded by the Ministry of Education (NRF-2016R1D1A1A02937335).
References
1. Armstrong DK, Bundy B, Wenzel L, Huang HQ, Baergen R, Lele S, Copeland LJ, Walker JL, Burger RA. Gynecologic Oncology Group. Intraperitoneal cisplatin and paclitaxel in ovarian cancer. N Engl J Med. 2006; 354:34–43.
2. Miller K, Wang M, Gralow J, Dickler M, Cobleigh M, Perez EA, Shenkier T, Cella D, Davidson NE. Paclitaxel plus bevacizumab versus paclitaxel alone for metastatic breast cancer. N Engl J Med. 2007; 357:2666–2676.
3. Sandler A, Gray R, Perry MC, Brahmer J, Schiller JH, Dowlati A, Lilenbaum R, Johnson DH. Paclitaxel-carboplatin alone or with bevacizumab for non-small-cell lung cancer. N Engl J Med. 2006; 355:2542–2550.
4. Wang TH, Wang HS, Soong YK. Paclitaxel-induced cell death: where the cell cycle and apoptosis come together. Cancer. 2000; 88:2619–2628.
5. Verweij J, Clavel M, Chevalier B. Paclitaxel (Taxol™) and docetaxel (Taxotere™): not simply two of a kind. Ann Oncol. 1994; 5:495–505.
6. Gilron I, Bailey JM, Tu D, Holden RR, Weaver DF, Houlden RL. Morphine, gabapentin, or their combination for neuropathic pain. N Engl J Med. 2005; 352:1324–1334.
7. Maestri A, De Pasquale Ceratti A, Cundari S, Zanna C, Cortesi E, Crinò L. A pilot study on the effect of acetyl-L-carnitine in paclitaxel-and cisplatin-induced peripheral neuropathy. Tumori. 2005; 91:135–138.
8. Dougherty PM, Cata JP, Cordella JV, Burton A, Weng HR. Taxolinduced sensory disturbance is characterized by preferential impairment of myelinated fiber function in cancer patients. Pain. 2004; 109:132–142.
9. Golden JP, Johnson EM. Models of chemotherapy drug-induced peripheral neuropathy. Drug Discov Today: Dis Model. 2004; 1:186–191.
10. Ward SJ, Ramirez MD, Neelakantan H, Walker EA. Cannabidiol prevents the development of cold and mechanical allodynia in paclitaxel-treated female C57Bl6 mice. Anesth Analg. 2011; 113:947–950.
11. Dworkin RH, O'Connor AB, Audette J, Baron R, Gourlay GK, Haanpää ML, Kent JL, Krane EJ, Lebel AA, Levy RM, Mackey SC, Mayer J, Miaskowski C, Raja SN, Rice AS, Schmader KE, Stacey B, Stanos S, Treede RD, Turk DC, Walco GA, Wells CD. Recommendations for the pharmacological management of neuropathic pain: an overview and literature update. Mayo Clin Proc. 2010; 85:3 Suppl. S3–S14.
12. Rowbotham M, Harden N, Stacey B, Bernstein P, Magnus-Miller L. Gabapentin for the treatment of postherpetic neuralgia: a randomized controlled trial. JAMA. 1998; 280:1837–1842.
13. Rice AS, Maton S. Postherpetic Neuralgia Study Group. Gabapentin in postherpetic neuralgia: a randomised, double blind, placebo controlled study. Pain. 2001; 94:215–224.
14. Backonja M, Beydoun A, Edwards KR, Schwartz SL, Fonseca V, Hes M, LaMoreaux L, Garofalo E. Gabapentin for the symptomatic treatment of painful neuropathy in patients with diabetes mellitus: a randomized controlled trial. JAMA. 1998; 280:1831–1836.
15. Tsavaris N, Kopterides P, Kosmas C, Efthymiou A, Skopelitis H, Dimitrakopoulos A, Pagouni E, Pikazis D, Zis PV, Koufos C. Gabapentin monotherapy for the treatment of chemotherapy-induced neuropathic pain: a pilot study. Pain Med. 2008; 9:1209–1216.
16. Partridge BJ, Chaplan SR, Sakamoto E, Yaksh TL. Characterization of the effects of gabapentin and 3-isobutyl-gamma-aminobutyric acid on substance P-induced thermal hyperalgesia. Anesthesiology. 1998; 88:196–205.
17. Gee NS, Brown JP, Dissanayake VU, Offord J, Thurlow R, Woodruff GN. The novel anticonvulsant drug, gabapentin (Neurontin), binds to the alpha2delta subunit of a calcium channel. J Biol Chem. 1996; 271:5768–5776.
18. Luo ZD, Chaplan SR, Higuera ES, Sorkin LS, Stauderman KA, Williams ME, Yaksh TL. Upregulation of dorsal root ganglion (alpha)2(delta) calcium channel subunit and its correlation with allodynia in spinal nerve-injured rats. J Neurosci. 2001; 21:1868–1875.
19. Tanabe M, Takasu K, Kasuya N, Shimizu S, Honda M, Ono H. Role of descending noradrenergic system and spinal alpha2-adrenergic receptors in the effects of gabapentin on thermal and mechanical nociception after partial nerve injury in the mouse. Br J Pharmacol. 2005; 144:703–714.
20. Hayashida K, Eisenach JC. Multiplicative interactions to enhance gabapentin to treat neuropathic pain. Eur J Pharmacol. 2008; 598:21–26.
21. Takasu K, Honda M, Ono H, Tanabe M. Spinal alpha(2)-adrenergic and muscarinic receptors and the NO release cascade mediate supraspinally produced effectiveness of gabapentin at decreasing mechanical hypersensitivity in mice after partial nerve injury. Br J Pharmacol. 2006; 148:233–244.
22. Caraceni A, Zecca E, Bonezzi C, Arcuri E, Yaya Tur R, Maltoni M, Visentin M, Gorni G, Martini C, Tirelli W, Barbieri M, De Conno F. Gabapentin for neuropathic cancer pain: a randomized controlled trial from the Gabapentin Cancer Pain Study Group. J Clin Oncol. 2004; 22:2909–2917.
23. Murotani T, Ishizuka T, Nakazawa H, Wang X, Mori K, Sasaki K, Ishida T, Yamatodani A. Possible involvement of histamine, dopamine, and noradrenalin in the periaqueductal gray in electroacupuncture pain relief. Brain Res. 2010; 1306:62–68.
24. Deluze C, Bosia L, Zirbs A, Chantraine A, Vischer TL. Electroacupuncture in fibromyalgia: results of a controlled trial. BMJ. 1992; 305:1249–1252.
25. Kim SK, Park JH, Bae SJ, Kim JH, Hwang BG, Min BI, Park DS, Na HS. Effects of electroacupuncture on cold allodynia in a rat model of neuropathic pain: mediation by spinal adrenergic and serotonergic receptors. Exp Neurol. 2005; 195:430–436.
26. Kim SK, Moon HJ, Park JH, Lee G, Shin MK, Hong MC, Bae H, Jin YH, Min BI. The maintenance of individual differences in the sensitivity of acute and neuropathic pain behaviors to electroacupuncture in rats. Brain Res Bull. 2007; 74:357–360.
27. Kim W, Kim SK, Min BI. Mechanisms of electroacupuncture-induced analgesia on neuropathic pain in animal model. Evid Based Complement Alternat Med. 2013; 2013:436913.
28. Bao T, Zhang R, Badros A, Lao L. Acupuncture treatment for bortezomib-induced peripheral neuropathy: a case report. Pain Res Treat. 2011; 2011:920807.
29. Donald GK, Tobin I, Stringer J. Evaluation of acupuncture in the management of chemotherapy-induced peripheral neuropathy. Acupunct Med. 2011; 29:230–233.
30. Lee JH, Go D, Kim W, Lee G, Bae H, Quan FS, Kim SK. Involvement of spinal muscarinic and serotonergic receptors in the anti-allodynic effect of electroacupuncture in rats with oxaliplatin-induced neuropathic pain. Korean J Physiol Pharmacol. 2016; 20:407–414.
31. Moon HJ, Lim BS, Lee DI, Ye MS, Lee G, Min BI, Bae H, Na HS, Kim SK. Effects of electroacupuncture on oxaliplatin-induced neuropathic cold hypersensitivity in rats. J Physiol Sci. 2014; 64:151–156.
32. Kim JH, Min BI, Na HS, Park DS. Relieving effects of electroacupuncture on mechanical allodynia in neuropathic pain model of inferior caudal trunk injury in rat: mediation by spinal opioid receptors. Brain Res. 2004; 998:230–236.
33. Park JH, Kim SK, Kim HN, Sun B, Koo S, Choi SM, Bae H, Min BI. Spinal cholinergic mechanism of the relieving effects of electroacupuncture on cold and warm allodynia in a rat model of neuropathic pain. J Physiol Sci. 2009; 59:291–298.
34. Choi JW, Kang SY, Choi JG, Kang DW, Kim SJ, Lee SD, Park JB, Ryu YH, Kim HW. Analgesic effect of electroacupuncture on paclitaxel-induced neuropathic pain via spinal opioidergic and adrenergic mechanisms in mice. Am J Chin Med. 2015; 43:57–70.
35. Smith SB, Crager SE, Mogil JS. Paclitaxel-induced neuropathic hypersensitivity in mice: responses in 10 inbred mouse strains. Life Sci. 2004; 74:2593–2604.
36. Li D, Lee Y, Kim W, Lee K, Bae H, Kim SK. Analgesic effects of bee venom derived phospholipase A(2) in a mouse model of oxaliplatin-induced neuropathic pain. Toxins (Basel). 2015; 7:2422–2434.
37. Flatters SJ, Bennett GJ. Ethosuximide reverses paclitaxel- and vincristine-induced painful peripheral neuropathy. Pain. 2004; 109:150–161.
38. Joseph EK, Levine JD. Comparison of oxaliplatin- and cisplatin-induced painful peripheral neuropathy in the rat. J Pain. 2009; 10:534–541.
39. Shibata K, Sugawara T, Fujishita K, Shinozaki Y, Matsukawa T, Suzuki T, Koizumi S. The astrocyte-targeted therapy by Bushi for the neuropathic pain in mice. PLoS One. 2011; 6:e23510.
40. Lee BH, Kim J, Lee RM, Choi SH, Kim HJ, Hwang SH, Lee MK, Bae CS, Kim HC, Rhim H, Lim K, Nah SY. Gintonin enhances performance of mice in rotarod test: involvement of lysophosphatidic acid receptors and catecholamine release. Neurosci Lett. 2016; 612:256–260.
41. Hirata H, Takahashi A, Shimoda Y, Koide T. Caspr3-deficient mice exhibit low motor learning during the early phase of the accelerated rotarod task. PLoS One. 2016; 11:e0147887.
42. Laughlin TM, Tram KV, Wilcox GL, Birnbaum AK. Comparison of antiepileptic drugs tiagabine, lamotrigine, and gabapentin in mouse models of acute, prolonged, and chronic nociception. J Pharmacol Exp Ther. 2002; 302:1168–1175.
43. Miranda HF, Noriega V, Zepeda R, Zanetta P, Prieto-Rayo J, Prieto JC, Sierralta F. Antinociceptive synergism of gabapentin and nortriptyline in mice with partial sciatic nerve ligation. Pharmacology. 2015; 95:59–64.
44. Yin CS, Jeong HS, Park HJ, Baik Y, Yoon MH, Choi CB, Koh HG. A proposed transpositional acupoint system in a mouse and rat model. Res Vet Sci. 2008; 84:159–165.
45. Kim W, Kim MJ, Go D, Min BI, Na HS, Kim SK. Combined effects of bee venom acupuncture and morphine on oxaliplatin-induced neuropathic pain in mice. Toxins (Basel). 2016; 8:33.
46. Karlsten R, Gordh T. How do drugs relieve neurogenic pain? Drugs Aging. 1997; 11:398–412.
47. Serpell MG. Neuropathic pain study group. Gabapentin in neuropathic pain syndromes: a randomised, double-blind, placebocontrolled trial. Pain. 2002; 99:557–566.
48. Gillin S, Sorkin LS. Gabapentin reverses the allodynia produced by the administration of anti-GD2 ganglioside, an immunotherapeutic drug. Anesth Analg. 1998; 86:111–116.
49. Xiao WH, Bennett G. Gabapentin has an antinociceptive effect mediated via a spinal site of action in a rat model of painful peripheral neuropathy. Analgesia. 1996; 2:267–273.
50. Rosner H, Rubin L, Kestenbaum A. Gabapentin adjunctive therapy in neuropathic pain states. Clin J Pain. 1996; 12:56–58.
51. Ling B, Authier N, Balayssac D, Eschalier A, Coudore F. Behavioral and pharmacological description of oxaliplatin-induced painful neuropathy in rat. Pain. 2007; 128:225–234.
52. Zbârcea CE, Negreş S, Cristea AN, Chiriţă C. The effect of dextromethorphan, gabapentin, amitriptyline and tramadol on a mouse model of vincristine-induced peripheral neuropathy. Farmacia. 2011; 59:809–819.
53. Gauchan P, Andoh T, Ikeda K, Fujita M, Sasaki A, Kato A, Kuraishi Y. Mechanical allodynia induced by paclitaxel, oxaliplatin and vincristine: different effectiveness of gabapentin and different expression of voltage-dependent calcium channel alpha(2)delta-1 subunit. Biol Pharm Bull. 2009; 32:732–734.
54. Eckhardt K, Ammon S, Hofmann U, Riebe A, Gugeler N, Mikus G. Gabapentin enhances the analgesic effect of morphine in healthy volunteers. Anesth Analg. 2000; 91:185–191.
55. Conno D. Gabapentin as an adjuvant to opioid analgesia for neuropathic cancer pain-report of two cases. J Pain Symptom Manage. 1999; 17:441–445.
56. Caraceni A, Zecca E, Martini C, De Conno F. Gabapentin as an adjuvant to opioid analgesia for neuropathic cancer pain. J Pain Symptom Manage. 1999; 17:441–445.
57. Gim GT, Lee JH, Park E, Sung YH, Kim CJ, Hwang WW, Chu JP, Min BI. Electroacupuncture attenuates mechanical and warm allodynia through suppression of spinal glial activation in a rat model of neuropathic pain. Brain Res Bull. 2011; 86:403–411.
58. Campero M, Serra J, Ochoa JL. C-polymodal nociceptors activated by noxious low temperature in human skin. J Physiol. 1996; 497:565–572.
59. Hao JX, Yu W, Xu XJ, Wiesenfeld-Hallin Z. Capsaicin-sensitive afferents mediate chronic cold, but not mechanical, allodynia-like behavior in spinally injured rats. Brain Res. 1996; 722:177–180.
60. Serra J, Solà R, Quiles C, Casanova-Molla J, Pascual V, Bostock H, Valls-Solé J. C-nociceptors sensitized to cold in a patient with smallfiber neuropathy and cold allodynia. Pain. 2009; 147:46–53.
61. Matthews EA, Dickenson AH. A combination of gabapentin and morphine mediates enhanced inhibitory effects on dorsal horn neuronal responses in a rat model of neuropathy. Anesthesiology. 2002; 96:633–640.
62. Woolf CJ, Mannion RJ. Neuropathic pain: aetiology, symptoms, mechanisms, and management. Lancet. 1999; 353:1959–1964.
63. Song EB, Yang JH, Lee SH, Kang YK, Na HS, Kim SJ. Effects of clonidine and epinephrine on neuropathic pain in an experimental animal model for peripheral neuropathy. J Korean Acad Rehabil Med. 1999; 23:101–108.
64. Hayashida K, Obata H, Nakajima K, Eisenach JC. Gabapentin acts within the locus coeruleus to alleviate neuropathic pain. Anesthesiology. 2008; 109:1077–1084.
65. Han JS, Xie GX, Zhou ZF, Folkesson R, Terenius L. Acupuncture mechanisms in rabbits studied with microinjection of antibodies against beta-endorphin, enkephalin and substance P. Neuropharmacology. 1984; 23:1–5.
66. Kwon YB, Kang MS, Ahn CJ, Han HJ, Ahn BC, Lee JH. Effect of high or low frequency electroacupuncture on the cellular activity of catecholaminergic neurons in the brain stem. Acupunct Electrother Res. 2000; 25:27–36.
67. Bodnar R, Paul D, Pasternak GW. Synergistic analgesic interactions between the periaqueductal gray and the locus coeruleus. Brain Res. 1991; 558:224–230.
68. Leite-Panissi CR, Brentegani MR, Menescal-de-Oliveira L. Cholinergic-opioidergic interaction in the central amygdala induces antinociception in the guinea pig. Braz J Med Biol Res. 2004; 37:1571–1579.
69. Shimoyama M, Shimoyama N, Inturrisi CE, Elliott KJ. Gabapentin enhances the antinociceptive effects of spinal morphine in the rat tail-flick test. Pain. 1997; 72:375–382.
70. Hayashida K, Parker R, Eisenach JC. Oral gabapentin activates spinal cholinergic circuits to reduce hypersensitivity after peripheral nerve injury and interacts synergistically with oral donepezil. Anesthesiology. 2007; 106:1213–1219.