Abstract
Ardipusilloside-I is a natural triterpenoid saponin, which was isolated from Ardisia pusilla A. DC. The aim of the study was to evaluate the stimulation of ardipusilloside-I on gastrointestinal motility in vitro and in vivo. The experiment of smooth muscle contraction directly monitored the contractions of the isolated jejunal segment (IJS) in different contractile states, and the effects of ardipusilloside-I on myosin were measured in the presence of Ca2+-calmodulin using the activities of 20 kDa myosin light chain (MLC20) phosphorylation and myosin Mg2+-ATPase. The effects of ardipusilloside-I on gastro emptying and intestinal transit in constipation-predominant rats were observed, and the MLCK expression in jejuna of constipated rats was determined by western blot. The results showed that, ardipusilloside-I increased the contractility of IJS in a dose-dependent manner and reversed the low contractile state (LCS) of IJS induced by low Ca2+, adrenaline, and atropine respectively. There were synergistic effects on contractivity of IJS between ardipusilloside-I and ACh, high Ca2+, and histamine, respectively. Ardipusilloside-I could stimulate the phosphorylation of MLC20 and Mg2+-ATPase activities of Ca2+- dependent phosphorylated myosin. Ardipusilloside-I also stimulated the gastric emptying and intestinal transit in normal and constipated rats in vivo, respectively, and increased the MLCK expression in the jejuna of constipation-predominant rats. Briefly, the findings demonstrated that ardipusilloside-I could effectively excite gastrointestinal motility in vitro and in vivo.
Ardipusilloside-I (Fig. 1A), a natural triterpenoid saponin extracted from Ardisia pusilla A. DC. (family Myrsinaceae, Jiujielong in Chinese) [1], was reported to have diverse pharmaceutical effects. Ardipusilloside-I could significantly inhibit the growth of many types of tumor, such as the liver, stomach, ovarian and lung tumors [2], and it could suppress the cancer cell proliferation, invasion and metastasis in vitro and in vivo, and prolong the survival time of liver cancer mice [3]. Ardipusilloside-I could also exert improvement effects on the immunological function of tumor bearing mice [4].
However, whether ardipusilloside-I could be used to relieve intestinal motility-related disorders remains unknown. Our pre-experiment showed that ardipusilloside-I exerted obviously stimulatory effects on gastrointestinal smooth muscle movement in both normal rats and gastrointestinal dysfunction rats, which were interesting and inspired us to characterize ardipusilloside-I-induced stimulation on gastrointestinal motility in rats and investigate its potential mechanism.
It is well known that the smooth muscle contraction and relaxation of smooth muscle mainly relates to the reversible Ca2+-calmodulin dependent phosphorylation of myosin light chains by myosin light chain kinase (MLCK) [5]. So the modulation of myosin provides an important index to evaluate the effects of modulators. In vertebrate tissues, MLCK is regulated by Ca2+-calmodulin, which is rate-limiting for contraction of smooth muscle [6]. For further clarifying the function of ardipusilloside-I in modulating the movement of smooth muscle, the present study was designed to inspect the contraction of intestinal smooth muscle, myosin phosphorylation levels and myosin Mg2+-ATPase activity. Additionally, in order to shed light on the mechanism of the ardipusilloside-I for alleviating constipation characterized by abnormal hypo-gut motility in a rat model, the gastric emptying, intestinal transit (a measurement of bowel movement) and the expression of MLCK in vivo were examined respectively.
These studies were approved by the Animal Care Committee of Liaoning University of Traditional Chinese Medicine with the certification No. SYXK (Liao) 2013-0009. The animal housing facility was a barrier housing facility, conforming to the national standard of China (Laboratory Animal-Requirements of Environment and Housing Facilities) (GB 14925-2001). Sprague-Dawley (SD) rats, half male and half female, weighing 180~220 g, were obtained from Laboratory Animal Research Center of Dalian Medical University with the certification No. SCXK (Liao) 2013-0003. Animals were housed five per cage in a temperature-controlled room (12 h/12 h light/dark, ad libitum access to food and water) for a week prior to experimentation.
Ardipusilloside-I (>98% pure, free of endotoxin) was provided by the Department of Pharmacy, the Fourth Military Medical University (Xi'an, China). Compound diphenoxylate (H32022716) was manufactured by Changzhou Kangpu Medicine Industry Co., Ltd. Phenylmethylsulfonyl fluoride (PMSF), dithiothreitol (DTT) were purchased from Sigma-Aldrich (St. Louis, MO, USA). All other used chemical reagents were of commercially available analytical grade. The proportions of constituents in Krebs's solution was as follows (in mM): sodium dehydrogenate phosphate 1.8, sodium chloride 114.0, potassium chloride 4.7, magnesium chloride 1.2, calcium chloride 2.5, glucose 11.5, sodium bicarbonate 18.0; pH 7.4.
The black semisolid paste was made according to the method described previously [78]. Carboxymethylcellulose (5 g in total) was added in two 2.5-g portions to 125 ml of distilled water. After the addition of each portion, the mixture was agitated in a blender for 3 min. Cane sugar (4 g in total) was added to this mixture, followed by 8 g of desiccated milk and 4 g of cornstarch. Following the addition of each portion of these ingredients, the mixture was agitated for 3 min. And then 1.5 g of anthracotic marker was added to the well-distributed mixture in three 0.5-g portions. The resulting mixture was a black semisolid paste (about 150 ml containing 150 g nutrient paste), which was placed in a refrigerator overnight to allow any air trapped in the mixture to escape. The mixture was removed from the refrigerator and returned to reach room temperature before 2 h for using.
The methods for determining gastric emptying and intestinal transit were described previously with some modifications [789]. Briefly, each rat was given by gavage 3 ml of test meal 60 min after the last administration with ardipusilloside-I. And then the rat was killed by cervical dislocation and laparotomized 20 min later. The stomach was ligated at the esophageal cardia and pylorus to prevent leakage, removed and weighed. The stomach was then opened, any remaining test meal was rinsed out, and any excess water was cleared from the stomach with filter paper. The empty stomach was then weighed. The amount of the remained test meal in the stomach was calculated by subtraction of the values of successive weighing. The entire small intestine was ligated, removed from the abdomen, and pull straight. Gastric emptying was calculated as [(gavaged test meal–remained test meal in gastric)/gavaged test meal], and intestinal transit was calculated as (the length between where the marker achieved in intestine and pylorus/the length between the pylorus and cecum).
Constipated rats model was induced by administered with atropine-diphenoxylate according to previously described methods with some modifications [10].
For measurement of the effects of ardipusilloside-I on gastric emptying and intestinal transit in normal rats, forty animals were randomly divided into four groups (n=10 per group): normal control group (NC), high, middle and low dose of ardipusilloside-I groups (100, 50, 25 mg/kg), respectively. Ardipusilloside-I was intragastric administration at a dose of 100, 50, 25 mg/kg body weight per day for high, middle, and low dose group rats, respectively, and the rats in the NC group were gavaged with the same amount of distilled water at the same time. After 14 days of administration, rats were fasted for 16 h with free access to water. Each rat was administrated with test meal and then following measurement was performed in accordance with the methods described above.
In constipated rats, the atropine-diphenoxylate was used to induce constipation according to previous reports [11]. Fifty animals were randomly divided into five groups, that is, the remaining rats were the same as the measurement in normal rats except that ten rats were selected as the model control group (MC). Except the NC rats, others were orally administered by atropine-diphenoxylate (diphenoxylate 5 mg/kg; atropine sulfate 0.05 mg/kg) once daily for 14 consecutive days to induce constipation. And then, the ardipusilloside-I was orally administered at a dose of 100, 50, 25 mg/kg body weight per day for high, middle, and low dose group rats for 14 days, respectively. Moreover, the rats in NC and MC group were gavaged with the same amount of distilled water. After 14 days of treatment, rats were fasted for 16 h with free access to water. After that, all animals were placed in small transparent cages individually and administrated with test meal, the following measurement was performed in accordance with the methods described above.
The stomach of rats was opened under urethane anesthesia. The small intestinal were prepared as described earlier [12] and the isolated jejunal segment (IJS) was cut in approximate 2 cm segment in length (tubes) to test the effect of ardipusilloside-I on the contractility of intestinal smooth muscle in rats. The mucosa and submucosa were removed gently with fine tweezers. The IJS was suspended respectively in 20 ml chambers containing 37℃ Kreb's solution with 95% O2 and 5% CO2, equilibrated for 50 min and the bath solution was replaced every 10 min. Contractile response was directly recorded by using RM6240 physiological recording system (Chengdu Instrument Factory, China). Contractile amplitude of the IJS was measured from the baseline to the peak and was expressed as the relative value (the average of the contractile curve recorded over 5 min), and compared to a normal control (Krebs' solution without ardipusilloside-I), which was assigned a value of 100%. The spikes presented simultaneously in both recorded channels were easily identified as movement artifacts which were eliminated from data analysis. Each rat acted as self-control, and recording time was not longer than 6 h. The mean amplitude of contraction was calculated from 6 independent assays.
Myosin and MLCK used in the essay were purified from fresh smooth muscle of chicken gizzard according to the methods reported previously [13]. The purified myosin was unphosphorylated, as determined by 10% glycerol electrophoresis. The method of measuring phosphorylation extents of 20 kDa regulating myosin light chain (MLC20) of myosin and Mg2+-ATPase activity of myosin was performed as described previously [1415]. In brief, Ca2+-calmodulin dependent phosphorylation of MLC20 by MLCK was carried out in a 20 mM Tris-HCl (pH 7.4) buffer containing 1 mM DTT, 5 mM MgCl2, 60 mM KCl, 2 mM EGTA, 4 µM myosin and 2 mM ATP. And the measurement of Mg2+-ATPase activity was carried out in a 20 mM Tris-HCl (pH 7.4) buffer containing 60 mM KCl, 5 mM MgCl2, 1 mM DTT, 0.5 mM ATP, 0.1 mM CaCl2, 0.6 mM calmodulin, and 0.4 µM myosin at 25℃ using the malachite green method. To analyze the percentage of MLC20 phosphorylation, Scion Image Software (Scion Co. Ltd., USA) was applied to scan the density and size of phosphorylated MLC20 and calculate the percentage of phosphorylation MLC20 in total MLC20. Mono-phosphorylation of the fully phosphorylated myosin control was chosen as the control and calculated as 100%.
MLCK protein expression in jejuna of constipated rats was examined using western blot as described previously [16]. Ardipusilloside-I at doses of 25, 50, 100 mg/kg was gavaged to constipated rats daily respectively as mentioned above. After 2-week of ardipusilloside-I administration, rats were sacrificed and jejunal segments were isolated. Total protein was isolated from the full thickness of jejunal wall using a Total Protein Extraction Kit (KeyGen Bio TECH, China). Anti-MLCK mAb (1:1000 dilution) (No. ab76092 abcam (Hong Kong) Ltd., UK) was incubated overnight at 4℃, followed by incubation with 1:1000 diluted HRP-conjugated goat antibody against rabbit IgG and stained with an enhanced chemiluminescence kit (KyeGen Bio TECH, China). The bands were detected and quantified using a Multispectral Imaging System (UVP, Cambridge, UK).
The results showed that the gastric emptying and the intestinal transit in ardipusilloside-I treated normal rats were faster than those in rats of NC group without receiving administration, particularly at the dose of 100 mg/kg and 50 mg/kg (p<0.01) (Fig. 1B). Fig. 1C showed that, the gastric emptying and intestinal transit in constipated rats of MC group were slower than those in normal rats of NC group (p<0.01). However, ardipusilloside-I (25, 50, and 100 mg/kg) could significantly promote the gastric emptying and intestinal transit in constipation-predominated rats (p<0.01). The results in both normal and constipationpredominated rats indicated that, ardipusilloside-I exerted obviously stimulatory effects on the gastrointestinal motility in a dose-dependent manner.
Ardipusilloside-I- induced effects on IJS contractility in normal contractile state were shown by its dose-response relationship. At concentration of 6.25–100 µM, ardipusilloside-I exerted stimulatory effects on the contractility of IJS in a concentration-dependent manner, especially at the doses greater than 12.5 µM (p<0.01).
To characterize ardipusilloside-I-induced stimulatory effects, 3 different low contractile states and high contractile states of IJS were established and used in the study, respectively. The results indicated that ardipusilloside-I increased the contractility of IJS in low contractile states induced by low concentration of Ca2+, adrenaline and atropine respectively. Additionally, for the contractility of IJS in high contractile states, the synergistic effects were showed between ardipusilloside-I and acetylcholine (ACh), high Ca2+ and histamine respectively.
Now that MLCK phosphorylates the MLC20 of myosin, which triggers cycling of myosin cross-bridges along actin filaments, resulting in motive force [17], the changes in myosin phosphorylation and Mg2+-ATPase activity reflect the catalytic activity of MLCK. The following assays were designed to reveal the potential mechanisms related to ardipusilloside-I-induced stimulatory effects, including MLCK expression in jejuna of constipation-predominated rats. In addition, myosin and MLCK purified from chicken gizzard smooth muscle were used to determine the MLCK catalytic activity. Due to the high sensitivity of MLC20 phosphorylation by MLCK in the presence of Ca2+ and Calmodulin [18], only 0.002 mM MLCK was added to the assay for MLC20 phosphorylation. Besides, the different incubation times (5, 10 and 20 min) were chosen to examine the myosin Mg2+-ATPase activities.
Fig. 4A indicates that ardipusilloside-I at concentrations from 12.5 µM to 100 µM induced a dose-dependent stimulation of MLC20 phosphorylation by MLCK. Fig. 4B showed that, the Mg2+-ATPase activities of Ca2+-dependent phosphorylated myosin increased gradually with incubation time. However, at different incubation time, the highest Mg2+-ATPase activities of phosphorylated myosin was observed in the group of 100 µM ardipusilloside-I, the second one in the 50 µM ardipusilloside-I, the third one in the 25 µM ardipusilloside-I, the fourth one in the 12.5 µM ardipusilloside-I, and the lowest in the negative control. The differences between the Mg2+-ATPase activities in different groups became more and more obvious along with the extension of incubation. So, ardipusilloside-I could stimulate Mg2+-ATPase activities of Ca2+-dependent phosphorylated myosin both in the absence and in the presence of actin, in the dose-dependent and time-dependent manners. Fig. 4C showed that, the MLCK expression in the jejuna was significantly decreased in constipated rats in comparison with that in normal control, and ardipusilloside-I significantly increased MLCK protein content in constipated rats.
In this study, ardipusilloside-I increased contractility of IJS in a dose-dependent manner and reversed the LCS of IJS induced by low Ca2+, adrenaline, and atropine respectively. Meanwhile, there was a synergistic effect between ardipusilloside-I and ACh, high Ca2+, and histamine, respectively. In accordance with the stimulatory effects on jejunal contractility, ardipusilloside-I stimulated the gastric emptying and intestinal transit in normal and constipated rats in vivo, respectively. All in all, the aforementioned results have pointed out that, ardipusilloside-I had the definite activation effects on the gastrointestinal motility in rats.
The MLC20 phosphorylation of myosin by Ca2+/calmodulindependent MLCK has been long-established to play a key role in regulation the contraction of smooth muscle [19]. Upon stimulation, smooth muscle cells release Ca2+ from the sarcoplasmic reticulum and extracellular Ca2+ influx into cells. The rise in intracellular Ca2+ results in rapid binding of Ca2+ to calmodulin and then activates MLCK. The activated MLCK phosphorylates the 20 kDa regulatory unit of myosin light chains (MLC20), which then activates myosin ATPase and triggers cross-bridges cycling and the development of contractile force [20]. Since the changes in myosin phosphorylation and Mg2+-ATPase activity loyally reflect the regulation of myosin function, we focused on these changes as important indexes to determine the effects of ardipusilloside-I on myosin in these experiments. Studies also indicate that increased MLCK expression and content rather than the change of myosin heavy chain content may be potential mechanisms for enhanced contractile reactivity in sensitized airways [21]. Targeted deletion of MLCK induced a significant loss of myosin phosphorylation, suggesting an essential role for MLCK in the contraction of smooth muscle and for gastrointestinal motility in vivo [22].
Our study was designed based on these previously investigated evidences. In accordance with the stimulatory effects on jejunal contractility, ardipusilloside-I up-regulated MLCK expression in jejuna of constipated rats and increased both phosphorylation and Mg2+-ATPase activity of purified smooth muscle myosin. These evidences imply that the increase of MLCK contents and stimulation of MLCK activity are involved in the ardipusilloside-I-induced stimulatory effects.
Chronic constipation is a worldwide public health issue, which is usually defined as infrequent or difficult passage of stool in both developed and developing countries. Epidemiologic studies showed that the median prevalence of constipation was 16% (range, 0.7%~79%) in adults overall and 33.5% in adults aged 60 to 101 years [23]. It significantly affects the quality of life because constipation can cause not only discomfort and restlessness but also abdominal distension, vomiting, restlessness, gut obstruction, and perforation and even is associated with fatal pulmonary embolism [24]. Most patients with constipation used laxative medicines, such as phenolphthalein, magnesium sulfate, liquid paraffin, etc. However, these drugs are not so ideal in clinical use because of their potential adverse side effects such as inducing tolerance, melanosis coli, or cathartic colon [25]. Therefore, it is still greatly necessary to develop a safe and effective agent for relieving the constipation.
Constipation is characterized by decreased gastrointestinal motility, and the gastro emptying, the transit time and the MLCK expression were decreased in patients with constipation [26]. Our studies indicated that ardipusilloside-I could prompt the gastrointestine movement and MLCK expression in constipation-predominant rats, which indicated that ardipusilloside-I possessed obvious therapeutic effects on constipation and may be developed as a drug for clinical treatment of constipation. In summary, the present results provide some valuable information for understanding the action mechanism of ardipusilloside-I on gastrointestinal function and the further clinical researching on ardipusilloside-I.
Figures and Tables
Fig. 1
Effects of ardipusilloside-I on gastrointestinal motility (X±s, n=10).
(A) The chemical structure of ardipusilloside-I. (B, C) The effects of ardipusilloside-I on gastric emptying and intestinal transit in normal rats and in constipated rats respectively. **p<0.01 compared with NC group. ##p<0.01 compared with MC group.
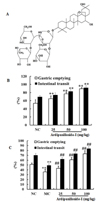
Fig. 2
Effects of ardipusilloside-I on the contractility of isolated jejunal segment (IJS) in normal contractile state (X±s, n=6).
(A) Representative traces and (B) statistical analysis of concentration- response relation of ardipusilloside-I on the contractility of IJS. The contractile amplitude of IJS in normal contractile state was set as 100% (NC). **p<0.01 compared with the NC.
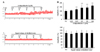
Fig. 3
Effects of ardipusilloside-I on the contractility of isolated jejunal segment (IJS) in different contractile states (X±s, n=6).
(A1) Representative traces and (A2) statistical analysis of ardipusilloside-I on the contractility of IJS in 3 low contractile states pre-induced with low Ca2+ (1.25 mM), adrenaline (1 µM), and atropine (1 µM), respectively. (B1) Representative traces and (B2) statistical analysis of ardipusilloside-I on the contractility of IJS in 3 high contractile states pre-induced with ACh (acetylcholine, 1 µM), high Ca2+ (5 mM), and histamine (5 µM) respectively. **p<0.01 compared with the NC, ##p<0.01 compared with the contractile amplitudes in low contractile states (LCS) and in high contractile states (HCS) before ardipusilloside-I treatment, respectively.

Fig. 4
Effects of ardipusilloside-I on phosphorylation and Mg2+-ATPase activity of myosin in vitro and content of MLCK in vivo (X±s, n=6).
The assays were performed in the assay as described in Materials and Methods. (A1) was glycerol electrophoresis results of ardipusilloside-I on the extent of myosin phosphorylation in a Ca2+-dependent way. LC20, unphosphorylated MLC20; p-LC20, mono-phosphorylated MLC20; LC17, 17 kDa myosin essential light chain. Lane 0 was unphosphorylation control, lane 1 was phosphorylation of myosin without ardipusilloside-I administered, lane 2~5 represented phosphorylation of myosin after 12.5, 25, 50, 100 µM ardipusilloside-I administered. (A2) was the extent of myosin phosphorylation, which was analyzed by using Scion Image Software. Mono-phosphorylation was chosen as the control and calculated as 100%, and other data were the relative values compared to the control. **p<0.01 compared with phosphorylation without ardipusilloside-I. (B1) and (B2) were the Mg2+- ATPase activities of Ca2+-dependent phosphorylated myosin in the absence and in the presence of actin, respectively. The Mg2+-ATPase activity of unphosphorylated myosin was calculated as 100% in the absence of ardipusilloside-I and actin. Other data obtained were the relative values compared to it. *p<0.05, **p<0.01 compared with the corresponding controls without ardipusilloside-I. (C1) and (C2) Representative bands and statistical analysis (columns) of MLCK protein contents in the absence and presence of ardipusilloside-I (25, 50, 100 mg/kg). Data obtained from normal control (NC) was set to 100%. **p< 0.01 compared with the NC, ##p< 0.01 compared with the constipated rats of MC group.

ACKNOWLEDGEMENTS
This study was supported by China Postdoctoral Science Foundation (Grant No. 2012M510834) and the National Key Basic Research Program of China (973 Program, Grant No. 2013CB531800).
Notes
References
1. Zhang QH, Huang SL, Wang XJ. Studies on the sapogenins and prosapogenins in Ardisia pusilla A. DC. Zhongguo Zhong Yao Za Zhi. 1993; 18:545–547.
2. Cao WY, Wang YN, Wang PY, Lei W, Feng B, Wang XJ. Ardipusilloside-I metabolites from human intestinal bacteria and their antitumor activity. Molecules. 2015; 20:20569–20581.
3. Xu XF, Zhang TL, Jin S, Wang R, Xiao X, Zhang WD, Wang PY, Wang XJ. Ardipusilloside I induces apoptosis by regulating Bcl-2 family proteins in human mucoepidermoid carcinoma Mc3 cells. BMC Complement Altern Med. 2013; 13:322.
4. Tao XJ, Long JW, He JY, Yao HP, Liu J, Cao YX. Antitumor and immunological regulation effects of ardipusilloside-I. Chinese Pharmacological Bulletin. 2005; 21:1070–1073.
5. Vale RD, Milligan RA. The way things move: looking under the hood of molecular motor proteins. Science. 2000; 288:88–95.
6. Olson NJ, Pearson RB, Needleman DS, Hurwitz MY, Kemp BE, Means AR. Regulatory and structural motifs of chicken gizzard myosin light chain kinase. Proc Natl Acad Sci U S A. 1990; 87:2284–2288.
7. Francis J, Dourish CT, Cooper SJ. Devazepide attenuates dlfenfluramine-induced suppression of gastric emptying but not food intake in the 17 h food-deprived rat. Physiol Behav. 1997; 62:545–550.
8. Francis J, Critchley D, Dourish CT, Cooper SJ. Comparisons between the effects of 5-HT and DL-fenfluramine on food intake and gastric emptying in the rat. Pharmacol Biochem Behav. 1995; 50:581–585.
9. Trudel L, Tomasetto C, Rio MC, Bouin M, Plourde V, Eberling P, Poitras P. Ghrelin/motilin-related peptide is a potent prokinetic to reverse gastric postoperative ileus in rat. Am J Physiol Gastrointest Liver Physiol. 2002; 282:G948–G952.
10. Xu J, Zhou X, Chen C, Deng Q, Huang Q, Yang J, Yang N, Huang F. Laxative effects of partially defatted flaxseed meal on normal and experimental constipated mice. BMC Complement Altern Med. 2012; 12:14.
11. Zhu F, Xu S, Zhang Y, Chen F, Ji J, Xie G. Total glucosides of paeony promote intestinal motility in slow transit constipation rats through amelioration of interstitial cells of cajal. PLoS One. 2016; 11:e0160398.
12. Yu C, Xiong Y, Chen D, Li Y, Xu B, Lin Y, Tang Z, Jiang C, Wang L. Ameliorative effects of atractylodin on intestinal inflammation and co-occurring dysmotility in both constipation and diarrhea prominent rats. Korean J Physiol Pharmacol. 2017; 21:1–9.
13. Lin Y, Ishikawa R, Okagaki T, Ye LH, Kohama K. Stimulation of the ATP-dependent interaction between actin and myosin by a myosin-binding fragment of smooth muscle caldesmon. Cell Motil Cytoskeleton. 1994; 29:250–258.
14. Tang ZY, Liu ZN, Fu L, Chen DP, Ai QD, Lin Y. Effect of lithium on smooth muscle contraction and phosphorylation of myosin light chain by MLCK. Physiol Res. 2010; 59:919–926.
15. Yang JX, Wang XM, Tang ZY, Chen H, Xu H, Lin Y. The characterization of Ca2+-calmodulin independent phosphorylation of myosin light chains by a fragment from myosin light chain kinase. Sheng Wu Hua Xue Yu Sheng Wu Wu Li Xue Bao (Shanghai). 2003; 35:793–800.
16. Xiong YJ, Chu HW, Lin Y, Han F, Li YC, Wang AG, Wang FJ, Chen DP, Wang JY. Hesperidin alleviates rat postoperative ileus through anti-inflammation and stimulation of Ca2+-dependent myosin phosphorylation. Acta Pharmacol Sin. 2016; 37:1091–1100.
17. Webb RC. Smooth muscle contraction and relaxation. Adv Physiol Educ. 2003; 27:201–206.
18. Tansey MG, Luby-Phelps K, Kamm KE, Stull JT. Ca2+-dependent phosphorylation of myosin light chain kinase decreases the Ca2+ sensitivity of light chain phosphorylation within smooth muscle cells. J Biol Chem. 1994; 269:9912–9920.
19. Gao N, Huang J, He W, Zhu M, Kamm KE, Stull JT. Signaling through myosin light chain kinase in smooth muscles. J Biol Chem. 2013; 288:7596–7605.
20. Lin G, Fandel TM, Shindel AW, Wang G, Banie L, Ning H, Lue TF, Lin CS. Modulation of smooth muscle tonus in the lower urinary tract: interplay of myosin light-chain kinase (MLCK) and MLC phosphatase (MLCP). BJU Int. 2011; 108:E66–E70.
21. Ammit AJ, Armour CL, Black JL. Smooth-muscle myosin light-chain kinase content is increased in human sensitized airways. Am J Respir Crit Care Med. 2000; 161:257–263.
22. He WQ, Peng YJ, Zhang WC, Lv N, Tang J, Chen C, Zhang CH, Gao S, Chen HQ, Zhi G, Feil R, Kamm KE, Stull JT, Gao X, Zhu MS. Myosin light chain kinase is central to smooth muscle contraction and required for gastrointestinal motility in mice. Gastroenterology. 2008; 135:610–620.
23. Wang J, Huang JH, Cheng YF, Yang GM. Banana resistant starch and its effects on constipation model mice. J Med Food. 2014; 17:902–907.
24. Mostafa SM, Bhandari S, Ritchie G, Gratton N, Wenstone R. Constipation and its implications in the critically ill patient. Br J Anaesth. 2003; 91:815–819.
25. Wald A. Chronic constipation: advances in management. Neurogastroenterol Motil. 2007; 19:4–10.
26. Xiong YJ, Chen DP, Lv BC, Liu FF, Wang L, Lin Y. Characteristics of nobiletin-induced effects on jejunal contractility. Fitoterapia. 2014; 94:1–9.