Abstract
Vascular reactivity can be influenced by the vascular region, animal age, and pathologies present. Prostaglandins (produced by COX-1 and COX-2) play an important role in the contractile response to phenylephrine in the abdominal aorta of young rats. Although these COXs are found in many tissues, their distribution and role in vascular reactivity are not clear. At a vascular level, they take part in the homeostasis functions involved in many physiological and pathologic processes (e.g., arterial pressure and inflammatory processes). The aim of this study was to analyze changes in the contractile response to phenylephrine of thoracic/abdominal aorta and the coronary artery during aging in rats. Three groups of rats were formed and sacrificed at three distinct ages: prepubescent, young and old adult. The results suggest that there is a higher participation of prostanoids in the contractile effect of phenylephrine in pre-pubescent rats, and a lower participation of the same in old rats. Contrarily, there seems to be a higher participation of prostanoids in the contractile response of the coronary artery of older than pre-pubescent rats. Considering that the changes in the expression of COX-2 were similar for the three age groups and the two tissues tested, and that expression of COX-1 is apparently greater in older rats, COX-1 and COX-2 may lose functionality in relation to their corresponding receptors during aging in rats.
Prostaglandins (PGs) produced by COX-1 and COX-2 play an important role in regulating the contractile effect of phenylephrine in abdominal aorta of young rats [1]. Vascular reactivity to different agonists is influenced by the region in which they are found, the age of the animal, and pathologies that may afflict the organism such as hypertension, diabetes or atherosclerosis [23].
Cyclooxygenase (COX) is the enzyme responsible for the conversion of arachidonic acid (AA) into prostaglandins (PGs). Two isoforms of COX (COX-1 and COX-2) have been identified [45] as the source of prostanoids in the arterial wall smooth muscle of the thoracic and abdominal aorta.
Tissular location of COX-1 and COX-2 differs from one species to another and even between tissues. Both these isoforms have important physiologic activities. At a vascular level, COX-1 reportedly participates in the functions of normal homeostasis by regulating arterial pressure, the formation of atheromas, and inflammatory processes [56789101112]. Connolly et al. [10] confirm that inhibitors of COX, such as nimesulide or indomethacin, reduce the vascular response of alpha adrenergic agonists in the thoracic aorta of rats without endothelium, possibly by preventing the formation of vasoconstrictor PGs. However the effect of alpha1-adrenergic agonists on the vascular tone is affected by many factors, including the regional characteristics of the vascular bed [1910], which could be modified by aging or development [2410].
In addition, different responses have been found when analyzing different regions of a single blood vessel. Gregg et al. [11] described variations along the thoracic aorta of guinea pigs in relation to the endothelium-dependent relaxation caused by acetylcholine, showing it to be less in the proximal than distal region of this artery [1112]. It has been suggested that such variation might be due to the difference in the number or affinity of endothelial muscarinic receptors in the different regions.
Matz et al. [13] describe that the enhanced contractions observed in young hypertensive rats seems to be the result of an increased participation of a cyclooxygenase dependent contracting factor, being this an important key role in the development of hypertension.
On the other hand, quantitative regional differences in the inhibition of the phenylephrine-induced contraction in rat aorta has been described [14]. Indomethacin inhibited this contraction in the abdominal but not thoracic segment, suggesting the participation of prostanoids in this inhibition [1415].
The aim of this work is to improve the information about the relationship of prostaglandins released by COXs in the contractile effect of phenylephrine and analyze if this participation have some changes during the development in the aorta and coronary artery.
All procedures and handling of the animals were in accordance with Mexican Federal Regulations for Animal Experimentation and Care (NOM-062-ZOO-1999, Ministry of Agriculture, Mexico City, Mexico) and approved by the Institutional Laboratory Animal Use and Care Committee (CICUAL) of the Escuela Superior de Medicina.
Determination was made of the participation of COX-1 and COX-2 in the contractile effect of phenylephrine in the coronary artery of perfused heart, and the thoracic and abdominal segments of rat aorta. Concentration curves were made for the response to phenylephrine in the presence or absence of indomethacin in the rings of the thoracic and abdominal aorta, as well as in the perfused hearts of rats in the different age groups. By means of the WB technique, the expression of COX-1 and COX-2 was analyzed in the different vessels and segments in the distinct age groups.
Male Wistar rats were housed in cages with a light/dark cycle of 12/12 hours, at a constant temperature (22±2℃) and with food and water available ad libitum. Animals were divided into three groups (n=6) and euthanized by decapitation (previously anaesthetized with pentobarbital at 60 mg/kg IP) at three different ages: 6 weeks (pre-pubescent), 16 weeks (young), and 12 months of age (old).
The thoracic and abdominal portions of the aorta were extracted after animal sacrifice. The thoracic aorta was excised from the diaphragm to the aortic arch, and the abdominal aorta from the diaphragm to the iliac artery. Aortic segments were immediately submerged in cold Krebs solution to remove all adjacent connective tissue. Both thoracic and abdominal segments were cut into aortic rings (4~5 mm long), each mounted on two stainless steel hooks in an isolated organ chamber. One of the hooks was fixed to the bottom of the chamber and the other to a transducer (TSD104) linked to a Biopac System, Inc. (Santa Barbara, Cal. USA) apparatus for registering changes in tension. The isolated organ chamber contained 10 ml of Krebs bicarbonate solution with the following composition (in mM): NaCl, 118; KCl, 4.7; KH2PO4, 1.2; MgSO4·7H2O, 1.2; CaCl2 .2H2O, 2.5; NaHCO3, 25; dextrose, 11.7; and calcium disodium EDTA, 0.026
The chamber was maintained at a constant temperature of 37℃ and pH of 7.4, being continuously bubbled with a mixture of 95% O2 and 5% CO2.
The rings received an initial tension of 1.5 (prepubescent) and 2 (young and older) grams and were allowed to stabilize for a period of 2 hours, during which time they were exposed to phenylephrine (10−6 M) every 30 minutes to test the viability of the tissue through its contractile response. Afterwards, the tissue was washed three times with Krebs solution to recover the basal level of tension. After a pre-contraction with phenylephrine, acetylcholine (10−5 M) was added to verify the integrity of the endothelium (aortic rings with endothelium has more than 80% of relaxation). Concentration-response curves were constructed in response to phenylephrine (1×10−9 to 1×10−5 M) after pre-treatment for 30 minutes with: indomethacin (1×10−5 M) (non-selective COX inhibitor), NS398 (1×10−6 M) (N-[2-(cyclohexyloxy)-4-nitrophenyl]-methanesulfonamide) (COX-2 selective inhibitor) and SC-560 (1×10−6 M) (5-(4-chlorophenyl)-1-(4-methoxyphenyl)-3-(trifluoromethyl)-1H-pyrazole) (COX-1 selective inhibitor).
Within a model of an isolated and perfused heart, the mechanical effects were examined and the perfusion pressure determined. After sacrifice, rats were placed in dorsal decubitus and the ribs were sectioned in two cuts parallel to the sternum's longest axis at the level of the anterior armpit line. The rib cage was held with clamps placed in the cephalic direction, exposing the heart and lungs. Immediately, the pericardium was removed and the connective tissue of the ascendant aorta dissected. The exposed heart was removed and submerged in cold Krebs solution with the following composition (mM): NaCl, 118; KCl, 4.7; KH2PO4, 1.2; MgSO4·7H2O, 1.2; CaCl2 .2H2O, 2.5; NaHCO3, 25; dextrose, 11.7; and calcium disodium EDTA, 0.026.
A cannula, inserted in the heart through the aorta, was connected to a constant-flow perfusion system consisting of a coil and an isolating chamber. A pressure transducer in this chamber was connected to the cannula to record the coronary perfusion pressure (linked to a Biopac System, Inc. (Santa Barbara, Cal. USA) apparatus). The perfusion solution was kept in a reservoir at a controlled temperature (37℃), subject to bubbling with carbogen (95% O2 and 5% CO2) at pH 7.4. This solution was supplied to the heart by means of a peristaltic pump at a constant flow miniplus 3, Gilson France (3~4 ml/min for prepubescents, 10~12 ml/min for young rats, and 10–14 ml/min for elderly rats). After allowing the system to stabilize for a period of 30 minutes, a pair of stimulating electrodes, made of fine stainless steel wire (vascular clamps/fine surgical instruments) were placed 2 mm apart in the apex of the right atrium. Pacing was achieved by applying electrical square pulses of 2 ms duration and twice the electrical threshold at a rate of 4.5±0.3 Hz. Concentration-response curves to phenylephrine were constructed for all three groups, using increasing concentrations from 1×10−9 to 1×10−5 M, pumped inside the aorta by means of continuous perfusion at 50 µl/min.
Approximately 20 mg of tissue from either heart, thoracic aorta or abdominal aorta were homogenized with a polytron device (PT 1200 E, Kinematica Technology, INC, NY, USA) in 200 µl of denaturing lysis buffer (1% triton X-100, 20 mM Tris, 140 mM NaCl, 2 mM EDTA, and 0.1% SDS) with protease and phosphatase inhibitor cocktails (P2714 and P2850, Sigma-Aldrich, St. Louis, MO, USA) supplemented with 0.15 mM PMSF, 5 mM Na3VO4 and 3 mM NaF. Homogenates were sonicated for 30 min at 4℃ and centrifuged (12,000 g) for 15 min. The total protein content was measured in the supernatant using the Bradford method. A total of 40 µg of protein was loaded into a denaturing 4%~15% TGX precast polyacrylamide gel (SDS-PAGE) (Bio-rad), electrotransferred into a polyvidinylidene fluoride membrane (PVDF Immobilon-P, Millipore) at 12 V for 50 minutes using a Trans-Blot Semidry system from Bio-rad. It was then incubated for 1 h in blocking solution (5% nonfat dry milk in TBS plus 0.1% Tween 20 [TBS-T]), followed by overnight incubation at 4℃ with primary antibodies. Mouse monoclonal anti-COX-1 (Abcam Cat. # 695), rabbit polyclonal anti-COX-2 Cat. # 15191) and anti-β-actin rabbit polyclonal (Abcam Cat. # ab129348) primary antibodies were diluted in TBS-T plus 5% nonfat dry milk. Membranes were washed (3X for 5 min) in TBS-T and incubated for 1 h at room temperature in the presence of HRP-conjugated secondary antibodies (anti-mouse IgG or anti-rabbit IgG HRP-linked antibodies, Cell Signaling Cat # 7076 and Cat # 7074, respectively) diluted 1:10,000 in blocking solution. Membranes were again washed 3 times in TBS-T, and the immunoblots were developed using an ECL Plus detection kit (Amersham-GE). The band intensities were digitally quantified using ImageJ software (http://www.nih.gov).
All values obtained represent the mean±SEM. In all the experiments, n equals the number of rats from which the aortic segments and hearts were obtained. The statistical comparisons were made by one-way ANOVA and a Tukey post-hoc test in order to determine significant differences. In all cases, a p value <0.05 was considered statistically significant.
In both aortic segments (thoracic and abdominal), the contractile effect of phenylephrine was similar in young rats and old rats (1.55 and 1.27 g for thoracic, 1.58 and 1.56 g for abdominal, respectively). However, it was significantly lower in both segments of pre-pubescent rats (1.12 g for thoracic, 0.85 g for abdominal) (Fig. 1).
The contractile effect in the abdominal segment decreased after pre-treatment with indomethacin in the three age groups (1.03 g, old; 0.95 g, young; 0.94 g, pre-pubescent). Unlike the significant differences between the three groups in regard to the contractile effect of the thoracic segment without pretreatment, there was significant difference in the contractile response of the thoracic aorta from the older rats compare with the other groups after pre-treatment with indomethacin (Fig. 2). Contrarily to that results, in the abdominal segment there was a higher indomethacin-induced inhibition of the phenylephrine contractile effect in pre-pubescent rats (0.16 g) and a much lower inhibition in old rats (0.98 g) (Fig. 3).
After exposure to phenylephrine, the perfusion pressure decreased in the hearts of old rats (17.917 mmHg) compared to the groups of young and pre-pubescent rats (23.250 and 20.556 mmHg, respectively) (Fig. 4). When administering the COX inhibitor, the only modification found was in the perfusion pressure of old rats, which decreased to 4 mmHg.
When applying SC560 (a selective COX-1 inhibitor), there was a significant inhibition of phenylephrine-induced contractile effect in the abdominal aorta of prepuber but not in old rats (Fig. 5). When applying NS398 (a selective inhibitor of COX-2), we observed the contrary effect, a significant increase in the phenylephrine-induced contractile effect in the aorta of old but not prepubescent rats. Indeed, in the latter animals an increase was found in this contractile effect (Fig. 6). In the thoracic aorta, on the other hand, there were no significant differences between the prepubescent and young treated groups after exposure of aortic rings to either of these COX inhibitors, however the older group shows an inhibitory effect after the pretreatment with SC560.
Protein density of COX-1 and COX-2 was measured by means of WB in thoracic and abdominal aortic rings as well as perfused hearts of the different age groups. There was an age-dependent increase in the expression of COX-1, but no significant differences were found in the level of COX-2 between age groups (Figs. 7 and 8).
There were no qualitative differences found in the phenylephrine-induced contractile effect when comparing the thoracic and abdominal segments in any of the groups of rats (prepubescent, young and old), being the maximal effect similar between the two segments.
Importantly, there were indeed qualitative differences between the two segments in the inhibition of the phenylephrine-induced contractile effect by indomethacin, This suggests a different participation of COXs and contractile prostanoids in both segments. These results are similar to those described in literature, where indomethacin (a non-selective inhibitor of COX) inhibited the contractile response to phenylephrine only in the abdominal but not the thoracic segment of the aorta. showing a significative inhibition in the abdominal segment but not iin the thoracic, there are similar reports that describes a similar inhibition in the abdominal [1131416].
On the other hand, the presence of COX-1 in the hearts of young adults has been described previously [512]. However, there have been no reports on the changes in the expression of COXs during development or the participation of these isoforms in the contractile effect during different stages. In this study we observed differences in the perfusion pressure to phenylephrine in all groups after perfusion of phenylephrine into the hearts. The lowest pressure was found in the group of old rats, results that are consistent with several reports [151617]. On the other hand, after the administration of indomethacin there was a decrease in the perfusion pressure in hearts of old rats previously perfused with phenylephrine. Similar results were found in the abdominal aorta, where the inhibition of the contractile response to phenylephrine was higher in the group of pre-pubescent rats and lower in the group of old rats This inhibition of the contractile effect in the abdominal segment and the decrease in perfusion pressure suggest that the contractile effect of phenylephrine was being mediated in both cases by a contractile prostanoid [18].
We considered that the regional difference should be associated with a variation in the density or particular isoform of COX present in each aortic segment. or to a difference in the coupling between the enzyme and the transduction system of α1-adrenoceptors. Therefore, we measured these factors [18].
In order to detect possible changes in the expression of COX-1 and COX-2 during development, their presence in different tissues and age groups was measured by Western Blot. We found that the expression of COX-1 increased during development, which may mean that COX-1 plays an important role at a cardiovascular level during development. Meanwhile, the expression of COX-2 was similar in the different age groups, which may indicate that this isoform does not participate in changes at the cardiovascular level that take place with growth and development [1920212223].
Lamb et al. [15] argue that prostanoids other than thromboxane are involved in the contraction induced by adrenergic amines in the abdominal but not the thoracic segment of the aorta. This is consistent with the idea that the intracellular signaling system of α1 adrenoceptors is complex and variable, including the participation of PGs, at least in certain cases [14].
There are studies demonstrating that prolonged inhibition of COX-2 is associated with an increase in blood pressure in young adults [20]. However, the present results indicate that the contractile response to phenylephrine in the thoracic segment was not modified by the presence of NS398, a selective antagonist of COX-2, in prepuber, otherwise we observed an increase in the contractile effect of phenylephrine in both segments (thoracic and abdominal) in old rats. Contrarily, the contractile response to phenylephrine in the abdominal segment was indeed modified by the presence of NS398 in prepuber rats, leading to an increase in the contractile response. This could be associated with the increase in blood pressure of young adults reported by Qi et al. [21]. In old rats, a decrease was found in the phenylephrine-induced contraction in abdominal aorta after pretreatment with NS398.
These results suggest that during prepuberty a greater expression of receptors to COX-2 may exist. However, the changes in COX-2 expression remained within the same levels during the development of the rats of the present study. These results lead us to conclude that the activity of these receptors is possibly greater during prepuberty than old age, taking into consideration that the values of expression were similar in the different age groups of the study.
Similarly, the current results indicate that the expression of COX-1 is greater in old than pre-pubescent rats. Hence, during prepuberty there may be a greater sensibility of COX-1, which would be gradually lost with age. This would explain how a greater expression of COX-1 in old rats could be accompanied by a lesser phenylephrine-induced contractile effect in this age group [21].
These results lead us to conclude that both COX-1 and COX-2 lose functionality in relation to their corresponding receptors during aging in rats. Likewise, considering that the changes in the expression of COX-2 are similar for the three age groups and the two tissues tested, the participation of PGs derived from COX-2 during prepuberty could lose its effect during development. Thus, we suggest that during the developmental stage corresponding to pre-puberty, there is a greater inhibition of the contractile effect in the abdominal segment, apparently due to a greater participation of contractile prostanoids mediated by COXs. This takes place in spite of the fact that there is a lower expression of COX-1 and a lower production of PGF2 alpha.
On the other hand, in old rats there was a lower inhibition of the contractile effect in the abdominal segment, which was associated with a lower participation of phenylephrine-mediated contractile prostanoids. However, there was a higher expression of COX-1 and higher PGF2 alpha levels in this stage of life. On the contrary, in the perfused heart there was a decrease in perfusion pressure in old rats, which indicates a higher participation of contractile prostanoids, effect that could be associate with the absence of endothelium, and this was releated with an increase in the oxidative stress (due to the age) that leading in an upregulation of COX1 expression and synthesis of prostanoids that modulates the contractile response [2223].
ACKNOWLEDGEMENTS
This work was supported by the SIP project (Escuela Superior de Medicina, IPN) and COFAA.
Notes
References
1. Castillo-Hernandez MC, Guevara-Balcazar G, Lopez-Sanchez P, Asbun-Bojalil J, Lopez RM, Castillo EF, Castillo-Henkel C. The influence of constitutive COX-2 in smooth muscle tissue on the contractile effect of phenylephrine in the rat abdominal aorta. Front Biosci (Elite Ed). 2010; 2:441–448. PMID: 20036891.
2. Kähönen M, Tolvanen JP, Sallinen K, Wu X, Pörsti I. Influence of gender on control of arterial tone in experimental hypertension. Am J Physiol. 1998; 275:H15–H22. PMID: 9688891.
3. Pairet M, Engelhardt G. Distinct isoforms (COX-1 and COX-2) of cyclooxygenase: possible physiological and therapeutic implications. Fundam Clin Pharmacol. 1996; 10:1–17. PMID: 8900495.


4. Smith WL, Dewitt DL. Prostaglandin endoperoxide H synthases-1 and -2. Adv Immunol. 1996; 62:167–215. PMID: 8781269.


5. Zidar N, Dolenc-Strazar Z, Jeruc J, Jerse M, Balazic J, Gartner U, Jermol U, Zupanc T, Stajer D. Expression of cyclooxygenase-1 and cyclooxygenase-2 in the normal human heart and in myocardial infarction. Cardiovasc Pathol. 2007; 16:300–304. PMID: 17868881.


6. Feng L, Sun W, Xia Y, Tang WW, Chanmugam P, Soyoola E, Wilson CB, Hwang D. Cloning two isoforms of rat cyclooxygenase: differential regulation of their expression. Arch Biochem Biophys. 1993; 307:361–368. PMID: 8274023.


7. O'Neill GP, Ford-Hutchinson AW. Expression of mRNA for cyclooxygenase-1 and cyclooxygenase-2 in human tissues. FEBS Lett. 1993; 330:156–160. PMID: 8365485.
8. Iseki S. Immunocytochemical localization of cyclooxygenase-1 and cyclooxygenase-2 in the rat stomach. Histochem J. 1995; 27:323–328. PMID: 7635765.


9. Luksha L, Poston L, Gustafsson JA, Aghajanova L, Kublickiene K. Gender-specific alteration of adrenergic responses in small femoral arteries from estrogen receptor-beta knockout mice. Hypertension. 2005; 46:1163–1168. PMID: 16216990.
10. Connolly C, McCormick PA, Docherty JR. Effects of the selective cyclooxygenase-2 inhibitor nimesulide on vascular contractions in endothelium-denuded rat aorta. Eur J Pharmacol. 1998; 352:53–58. PMID: 9718267.


11. Gregg AR, Thompson LP, Herrig JE, Weiner CP. Regionalization of endothelium-dependent relaxation in the thoracic aorta of pregnant and nonpregnant guinea pigs. J Vasc Res. 1995; 32:106–111. PMID: 7734656.


12. Perrone MG, Scilimati A, Simone L, Vitale P. Selective COX-1 inhibition: A therapeutic target to be reconsidered. Curr Med Chem. 2010; 17:3769–3805. PMID: 20858219.
13. Matz RL, Van Overloop B, Gaubert E, Gairard A. Maturation reveals a decrease in endothelium-dependent contraction induced by depolarization in the aorta of spontaneously hypertensive rats. Life Sci. 2001; 69:1791–1804. PMID: 11665841.


14. Asbún-Bojalil J, Castillo EF, Escalante BA, Castillo C. Does segmental difference in alpha 1-adrenoceptor subtype explain contractile difference in rat abdominal and thoracic aortae? Vascul Pharmacol. 2002; 38:169–175. PMID: 12402516.
15. Lamb VL, Schwartz AJ, Rohn WR, Kaiser L. Cyclooxygenase inhibitors depress norepinephrine constriction of rat abdominal, but not thoracic, aorta. Eur J Pharmacol. 1994; 256:221–226. PMID: 7914169.


16. Asano K, Lilly CM, Drazen JM. Prostaglandin G/H synthase-2 is the constitutive and dominant isoform in cultured human lung epithelial cells. Am J Physiol. 1996; 271:L126–L131. PMID: 8760142.


17. Hennan JK, Huang J, Barrett TD, Driscoll EM, Willens DE, Park AM, Crofford LJ, Lucchesi BR. Effects of selective cyclooxygenase-2 inhibition on vascular responses and thrombosis in canine coronary arteries. Circulation. 2001; 104:820–825. PMID: 11502709.


18. Vane JR, Bakhle YS, Botting RM. Cyclooxygenases 1 and 2. Annu Rev Pharmacol Toxicol. 1998; 38:97–120. PMID: 9597150.


19. Morita I. Distinct functions of COX-1 and COX-2. Prostaglandins Other Lipid Mediat. 2002; 68-69:165–175. PMID: 12432916.


20. LaBelle EF, Polyak E. Norepinephrine stimulates arachidonic acid release from vascular smooth muscle via activation of cPLA2. Am J Physiol. 1998; 274:C1129–C1137. PMID: 9575810.
21. Qi Z, Cai H, Morrow JD, Breyer MD. Differentiation of cyclooxygenase 1- and 2-derived prostanoids in mouse kidney and aorta. Hypertension. 2006; 48:323–328. PMID: 16801485.


22. Kangussu LM, Olivon VC, Arifa RD, Araújo N, Reis D, Assis MT, Soriani FM, de Souza Dda G, Bendhack LM, Bonaventura D. Enhancement on reactive oxygen species and COX-1 mRNA levels modulate the vascular relaxation induced by sodium nitroprusside in denuded mice aorta. Fundam Clin Pharmacol. 2015; 29:150–163. PMID: 25619310.


23. Kumar P, Padi SS, Naidu PS, Kumar A. Cyclooxygenase inhibition attenuates 3-nitropropionic acid-induced neurotoxicity in rats: possible antioxidant mechanisms. Fundam Clin Pharmacol. 2007; 21:297–306. PMID: 17521299.


Fig. 1
Contractile effects of phenylephrine in thoracic and abdominal aorta.
Contractile effect of phenylephrine (1×10−9 to 1×10−5 M) in thoracic aorta (A) and abdominal (B) from pre-pubescent, young and old. Each point represent the mean±S.E.M. n=5. *p≤0.05.

Fig. 2
Contractile effect of phenylephrine with indomethacin.
Contractile effect of phenylephrine (1×10−9 to 1×10−5 M) in thoracic aorta from 6 weeks old (A), 16 weeks old (B) and 1 year old (C), with and without indomethacin (1×10−5 M). Each point represent the mean±S.E.M. n=5. *p≤0.05.

Fig. 3
Effects of indomethacin on the phenylephrine-induced contraction in abdominal aorta.
Contractile effect of phenylephrine (1×10−9 to 1×10−5 M) in abdominal aorta from 6 weeks old (A), 16 weeks old (B) and 1 year old (C), with and without indomethacin (1×10−5 M). Each point represent the mean±S.E.M. n=5. *p≤0.05.
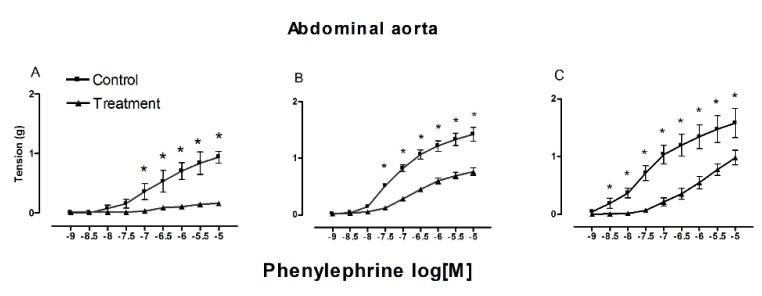
Fig. 4
Contractile effect of phenylephrine in isolated heart.
Contractile effect of phenylephrine (1×10−9 to 1×10−5 M) in isolated heart from 6 weeks old (A), 16 weeks old (B) and 1 year old (C), with and without indomethacin (1×10−5 M). Each point represent the mean±S.E.M. n=5. *p≤0.05.
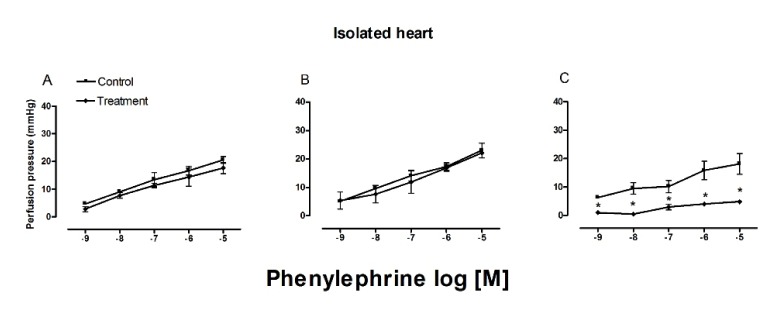
Fig. 5
Effect of SC560 on the phenylephrine-induced contraction.
Contractile effect of phenylephrine (1×10−9 to 1×10−5 M) in thoracic and abdominal aorta from 6 weeks old (A) and 1 year old (B), with and without SC560 (1×10−6 M). Each point represent the mean±S.E.M. n=5. *p≤0.05.

Fig. 6
Effect of NS398 on the phenylephrine-induced contraction.
Contractile effect of phenylephrine (1×10−9 to 1×10−5 M) in thoracic and abdominal aorta from 6 weeks old (A) and 1 year old (B), with and without NS398 (1×10−6 M). Each point represent the mean±S.E.M. n=5. *p≤0.05.
