Abstract
Epidemiologic interest in particulate matter (PM) is growing particularly because of its impact of respiratory health. It has been elucidated that PM evoked inflammatory signal in pulmonary epithelia. However, it has not been established Ca2+ signaling mechanisms involved in acute PM-derived signaling in pulmonary fibroblasts. In the present study, we explored dust particles PM modulated intracellular Ca2+ signaling and sought to provide a therapeutic strategy by antagonizing PM-induced intracellular Ca2+ signaling in human lung fibroblasts MRC5 cells. We demonstrated that PM10, less than 10 µm, induced intracellular Ca2+ signaling, which was mediated by extracellular Ca2+. The PM10-mediated intracellular Ca2+ signaling was attenuated by antioxidants, phospholipase blockers, polyADPR polymerase 1 inhibitor, and transient receptor potential melastatin 2 (TRPM2) inhibitors. In addition, PM-mediated increases in reactive oxygen species were attenuated by TRPM2 blockers, clotrimazole (CLZ) and N-(p-amylcinnamoyl) anthranilic acid (ACA). Our results showed that PM10 enhanced reactive oxygen species signal by measuring DCF fluorescence and the DCF signal attenuated by both TRPM2 blockers CLZ and ACA. Here, we suggest functional inhibition of TRPM2 channels as a potential therapeutic strategy for modulation of dust particle-mediated signaling and oxidative stress accompanying lung diseases.
Respiratory tract is a primary target because it is the first barrier to inhaled dust particles, which are associated with increased respiratory morbidity and mortality. Available evidence indicates dust particles mediate airway inflammation, the progression of asthmatic diseases [1], pneumonia [23], and inflammation by pathogens, and that they are even linked to brain structural changes and stroke [45]. Lungs provide large surface areas for the exchange of gases, but in so doing are exposed to exogenous particles. Particle size critically determines inhaled particulate uptake [6]. Fine particulates (less than 10 µm) reach the alveolar region and are cleared slowly because of the lack of a clearing mechanism [789]. For this reason, smaller particles and its extended deposition can be used to understand related downstream responses. Fine dust particles prefer respiratory invasion of not only nasal and bronchial epithelial layers but also of deep alveoli and other extra-pulmonary compartments including fibrotic tissue, lymph nodes, and the bloodstream [1011].
Pulmonary health is also threatened by dust particles that induce inflammatory and allergic reactions and cause Ca2+ overloading and the production of reactive oxygen species (ROS) [12]. Dust particles induce inflammatory reactions by inducing the productions of cytokines and chemokines, such as, transforming growth factor β1 (TGF-β1), interleukin-1, IL-6, and IL-8 [13141516]. In addition, particle-induced oxidative stress triggers immune reactions in alveolar macrophages and lung tissues [13171819], and these reactions have been associated with Ca2+, a critical pro-inflammatory mediator signal [19]. Intracellular Ca2+ is known to act as a pivotal intracellular messenger, and has been demonstrated to regulate several pulmonary functions, such as, ciliary beat frequency and mucus secretion [20212223], and mechanical movement of fibroblasts [2425].
Dust particles are known to increase an intracellular Ca2+ concentration ([Ca2+]i), which up-regulates transcription factors and the productions of their downstream mediators in airway epithelial cells [19]. Therefore, dust particles may influence the developments and exacerbations of respiratory diseases directly or indirectly [14]. However, it cannot be ruled out the possible mechanism sustained dust particles is a regulatory factors for development of inflammatory signaling in respiratory fibroblasts.
Although the above-mentioned studies have explored Ca2+ response in airway epithelial cells exposed to several particle types from ambient or chemical sources, the relationship between calcium response and signaling proteins has not been previously examined in bronchial fibroblasts. Accordingly, the goals of this study were to define changes in intracellular Ca2+ and signaling mechanisms in lung fibroblast cells exposed to dust particles PM10 and to explore the therapeutic agents responsible for particles-mediated signaling mechanism.
Human lung fibroblast cell line MRC5 cells were purchased from American Type Culture Collection (Rockville, MD). Fura-2-AM was purchased from Teflabs (Austin, TX). U73122 and its inactive analog, U73343 were from Tocris (Minneapolis, MN). Caffeine, clotrimazole (CLZ), 3-Aminobenzamide (3-AB), N-(p-amylcinnamoyl) anthranilic acid (ACA), 2-aminoethoxydiphenyl borate (2-APB), Chlorpromazine (CLP), N-acetylcysteine (NAC), lanthanum chloride (LaCl3), and all other chemicals were from Sigma. Dulbecco's Modified Eagle's Medium (DMEM), penicillin-streptomycin, trypsin-ethylenediaminetetraacetic acid (EDTA), 5-(and-6)-choloromethyl-2′,7′-dicholorodihydrofluorescin diacetate (CM-H2DCFDA), phosphate-buffered saline (PBS), fetal bovine serum (FBS), Pluronic F-127 (20% in DMSO), and 1,2-bis (2-aminophenoxy) ethane-N,N,N',N'-tetraacetic acid tetrakis, acetoxymethyl ester (BAPTA,AM) were from Invitrogen (Carlsbad, CA). MRC5 cells were incubated at 37℃ in a humidified 5% CO2/95% air atmosphere in DMEM containing 10% FBS, 100 U/mL penicillin, and 100 µg/mL streptomycin. When the cell culture reached 80% confluence, cells were dispersed by adding trypsin-EDTA for 2 min and then transferred to new culture dishes or to glass coverslip-covered dishes for Ca2+ measurements.
Ambient air samples were collected in Incheon City, South Korea and analyzed as described previously [2]. Collected outdoor dust particles were sonicated for 3 min at maximal watt and sieved through filters at 10 µm in size (Mitex membrane filters, Millipore). PM10 suspensions consisted of: 48% SiO2, 12% AL2O3, 5% Fe2O3, 5% CaO, 4% K2O, 2.37% MgO, 2% Na2O, and 1% TiO2. PM10 suspensions were heated at 360℃ for 30 min to remove adhered microorganisms or other organic materials and stored at −20℃ until use. No LPS in heated PM10 was detected (below range of 0.005 EU/ml) by a Limulus amebocyte lysate assay kit (BioWhittaker, MD). Particles were diluted in water and its size was measured in a Zeta potential and particle size analyzer (ELSZ-1000, Otsuka Electronics, Japan).
MRC5 cells cultured on cover glasses were incubated with 4 µM fura-2, AM in the presence of 0.05% Pluronic F-127 for 15 min in physiological salt solution (PSS) at room temperature in the dark, and then washed for 10 min with PSS (containing in mM: 10 D-glucose, 140 NaCl, 5 KCl, 1 MgCl2, 1 CaCl2, and 10 HEPES and titrated to pH 7.4). For the 0 Ca2+ extracellular solution, CaCl2 was replaced with 10 mM EGTA. Changes in intracellular Ca2+ concentrations were determined by measuring the fluorescence intensities using dual excitation wavelengths (340 and 380 nm) and an emission wavelength of 510 nm. Results are presented as fluorescence (F) ratios (Ratio=F340/380). Emitted fluorescence was monitored using a CCD camera (Photometrics, AZ) attached to an inverted microscope (Olympus, Japan) and analyzed with a MetaFlour system (Molecular Devices, PA). Fluorescence images were obtained at 1 sec intervals. Background fluorescence at each excitation wavelength was subtracted from raw signals.
MRC5 cells grown on a cover glass in the presence or absence of PM10 were incubated in PSS containing the ROS fluorescence probe 10 µg/mL H2DCFDA for 5 min and then washed with PBS for 5 min at room temperature. Fluorescence images were obtained using a confocal laser-scanning microscope (Leica, Buffalo, NY) using an excitation wavelength of 488 nm for DCF and measuring emitted light intensities at 525 nm. DCF fluorescence was measured in five different regions of selected images and signals were normalized versus baseline. Images were obtained at 10 min intervals.
To characterize the source of Ca2+ induced by PM10, MRC5 cells were stimulated by PM10 in the presence or absence of extracellular Ca2+. The PM10-induced [Ca2+]i signal was dramatically reduced in the absence of extracellular Ca2+ (Fig. 1A and 1C; n=25 cells). When treated with BAPTA,AM as a broad range of Ca2+ chelator, the PM10-induced [Ca2+]i signal was blocked by the chelation of basal and increased Ca2+ (Fig. 1B and 1C; n=28 cells). These observations showed that PM10 induced an increased [Ca2+]i signal which was mediated by extracellular Ca2+ in MRC5 cells. To confirm PM10-induced [Ca2+]i increases were mainly mediated by extracellular media, cells were treated with PM10 in the presence of a non-selective Ca2+ channel blocker La3+. PM10-mediated [Ca2+]i increases were blocked by La3+ (Fig. 1D and 1E, n=60 cells). Collected dust particles were characterized as PM10 (less than 10 µm), which including less than 2.5 µm dust particles PM2.5 (Fig. 1F).
To determine the involvement of the phospholipase C (PLC)/IP3 receptor (IP3R) pathway in PM10-induced [Ca2+]i responses, MRC5 cells were pre-treated with caffeine, which is an antagonist of IP3 receptor (IP3R) in many types of cells [262728]. The PM10-induced [Ca2+]i signal was measured in pretreatment with 20 mM caffeine for 3 min. Caffeine suppressed the [Ca2+]i increase induced by PM10 (Fig. 2A and B, n=64 and 97 cells, respectively). To determine the role of PLC in the effect of PM10, cells were pretreated with U73122 (a specific blocker of PLC) or with its inactive analog U73343. U73122 blocked PM10-induced [Ca2+]i increases, but U73343 did not (Fig. 2B and 2C, n=31 and 60 cells, respectively). These results suggest that PM10-induced [Ca2+]i increases are dependent on the PLC/IP3R pathway in MRC5 cells.
ROS generation by airway epithelia is a characteristic feature of respiratory diseases, such as, chronic obstructive pulmonary disease (COPD) [29], and therefore, the targeting of ROS represents a potential therapeutic strategy in such patients. Furthermore, dust particles have been shown to promote the production of ROS in bronchial epithelial cells [13]. To probe this situation we treated with PM10 in MRC5 cells in the presence of several ROS scavengers. PM10-induced [Ca2+]i signals were modestly attenuated by the antioxidant NAC (Fig. 3A and 3B, n=45 and 65 cells, respectively), indicating that ROS production is involved in Ca2+ signaling mechanism. It is known intracellular ROS increases polyADP-ribose (ADPR) polymerase 1 (PARP-1) activity, that this up-regulation is associated with subsequent DNA damage. PARP is a cardinal factor downstream of ROS production facilitates ADPR synthesis [30]. To determine whether PM10-induced ROS responses influence PARP-1 signaling, we used a PARP-1 inhibitor 3-AB [31]. Pretreatment with 3-AB was found to markedly inhibit PM10-mediated [Ca2+]i increases (Fig. 3C, n=36 cells). Cells were also pretreated with a Ca2+-CaM inhibitor CLP to determine whether CaM was involved in PM10-triggered [Ca2+]i signaling [32]. PM10-induced [Ca2+]i increases were inhibited by CLP (Fig. 3D, n=30 cells), indicating PM10-induced increases in [Ca2+]i in lung fibroblast cells are mediated by oxidative signaling and are dependent on PARP-1 signaling.
Increased PARP activity or [Ca2+]i increases are required for the activation of transient receptor potential melastatin 2 (TRPM2) [3334], and TRPM2 channel is activated by intracellular ADPR and ROS messengers, which leads to excessive Ca2+ influx [230]. To determine whether the PM10-induced [Ca2+]i signal was mediated by TRPM2, MRC5 cells were pretreated with several types of TRPM2 blockers 2-APB, CLZ, or ACA and then treated with PM10. It is known that 2-APB blocks ADPR-induced TRPM2 currents in several cell types [3536]. We found pretreatment with 2-APB or CLZ (Fig. 4A, 4B, and 4C, n=51, 32, and 60 cells, respectively) also significantly decreased PM10-induced Ca2+ influx into lung fibroblast cells. The pretreatment of derivative anthranilic acid, N-(p-amylcinnamoyl) anthranilic acid ACA [36] markedly decreased PM10-mediated influx despite initially increasing [Ca2+]i (Fig. 4D, n=52 cells). These results suggest that the [Ca2+]i response observed in PM10-stimulated bronchial epithelial cells required PARP/ADPR signaling to mediate activation of oxidative stress-dependent channels such as TRPM2 activation.
MRC5 cells were loaded with H2DCFDA to determine extents of PM10-induced ROS signal. DCFDA fluorescence, which was used to detect H2O2, was time-dependently increased by PM10 (Fig. 5A; n=7), but pretreatment with both TRPM2 inhibitors CLZ or ACA resulted in the disappearance H2O2 from cells (Fig. 5B and 5C). To avoid the effect of dilution vehicle, PM10 suspension was included with DMSO. These result suggested that PM10 induced ROS signal with time-dependent manner and the ROS signal attenuated by both TRPM2 blockers, CLZ and ACA.
Ambient epidemiologic studies have demonstrated that increases in the morbidities and mortalities of pulmonary diseases are correlated with pollution of the environment by particulate matter (PM). Thus, clarifying the mechanisms of airway diseases induced by PM would support understanding of their pathogeneses. This study demonstrates that PM10 mediates ROS production and [Ca2+]i signaling in a human lung fibroblast cell line. In view of the inflammatory effects of dust particles on respiratory organs such as bronchial epithelia is not surprising; however, the present study shows inflammatory signaling upstream of PM is primarily associated with oxidative stress-dependent Ca2+ channels such as TRPM2, which triggers and promotes an inflammatory stream of extracellular Ca2+ into lung fibroblasts.
Ca2+ homeostasis is related to a variety of cellular functions, which include protein synthesis, transcription factor activation, cell migration, proliferation, and the transduction of extracellular signals into cells [37]. [Ca2+]i is tightly maintained by Ca2+ pump, which hydrolyzes ATP to provide the energy for Ca2+ movement and for store operated Ca2+ influx channels, such as, stromal interaction molecule 1 (STIM1) and Orai. Few studies have addressed the pathological aspects of Ca2+ signaling in pulmonary fibroblasts, although a large number of studies have been undertaken on non-pulmonary fibrotic organs, such as, heart [38], liver [39], and kidneys [40]. In the present study, PM10 evoked a delayed [Ca2+]i signal (Fig. 1), indicating the involvements of other signaling mechanisms prior to Ca2+ influx, and thus, it is important to determine whether dust particles induce ROS-mediated signaling. Although previous report addressed that air pollutants evoke both Ca2+-dependent or -independent mechanisms [19], our findings for the first time suggest that dust particle-mediated ROS production participates in [Ca2+]i signaling events via TRPM2 activation in pulmonary fibroblasts, which further suggests dust particles-mediated TRPM2 activation initiates inflammatory events such as increased proinflammatory cytokines level. On the other hands, TRPM2 activation, which enables excessive Ca2+ influx, phosphorylates and activates the PLCγ1/protein kinase Cα (PKCα) pathway, which subsequently induces airway epithelial tight junction disruption [41]. Particulate stimuli are common events in NLRP3 inflammasome activation [42].
We cannot exclude the possibility that additional [Ca2+]i signaling mechanisms are involved. Our results indicate dust particles elicit [Ca2+]i increases in a IP3/IP3R pathway dependent-manner. Kyung et al. [13] suggested that dust particles may have fibrotic potential in bronchial epithelial cells. This mechanism also allows us to predict and test of potential novel approaches for the treatment of pulmonary fibrotic diseases by long-term exposure of dust particles. However, the mechanism responsible for dysfunctional PLC/IP3/IP3R signaling by dust particles requires additional investigation in pulmonary fibrosis.
PM deposited in alveolar epithelia causes structural modifications in intracellular organelles and pathological changes in alveolar cells [43]. Furthermore, PM-mediated oxidative stresses are closely related to cell toxicity. In one study, PM2.5-mediated DNA damage was abolished by anti-oxidant enzymes or deferoxamine, indicating oxidative mediators are involved in the induction of DNA damage [44]. Oxidative events are also associated with many pathologic conditions, such as, pulmonary fibrosis and COPD [294546]. Indeed, blockade of ROS signaling or TGF-β1 has an anti-fibrotic effect in lung tissue [134647]. In addition, at elevated doses, PM appears to increase ROS levels and subsequent mitochondrial damage, such as mitochondrial swelling and vacuolation, in alveolar type II cells [43].
This study provides the role of [Ca2+]i signaling induced by dust particles in respiratory fibroblast cells. Currently, no therapeutic strategy targets [Ca2+]i signaling in fibroblast-mediated pulmonary diseases [48], but our results suggest potential means of controlling fibrotic pulmonary diseases by manipulating [Ca2+]i signaling events. In particular, we suggest antioxidant, [Ca2+]i signaling pathway inhibitor, and TRPM2 inhibitor treatments should be considered for therapeutic trials in fibrotic diseases. Importantly, this study shows the modulation of dust particles-mediated [Ca2+]i signaling has the potential to generate a new wave of therapies targeting pulmonary fibroblasts.
ACKNOWLEDGEMENTS
This research was supported by Basic Science Research Program through the National Research Foundation of Korea (NRF) funded by the Ministry of Science, ICT & Future Planning (2014R1A1A3049477) by Gachon University, Gil Medical Center Research Fund (FRD2015-13).
Notes
References
1. Kanatani KT, Ito I, Al-Delaimy WK, Adachi Y, Mathews WC, Ramsdell JW. Toyama Asian Desert Dust and Asthma Study Team. Desert dust exposure is associated with increased risk of asthma hospitalization in children. Am J Respir Crit Care Med. 2010; 182:1475–1481. PMID: 20656941.


2. He M, Ichinose T, Yoshida S, Yamamoto S, Inoue K, Takano H, Yanagisawa R, Nishikawa M, Mori I, Sun G, Shibamoto T. Asian sand dust enhances murine lung inflammation caused by Klebsiella pneumoniae. Toxicol Appl Pharmacol. 2012; 258:237–247. PMID: 22118940.


3. Yeo NK, Hwang YJ, Kim ST, Kwon HJ, Jang YJ. Asian sand dust enhances rhinovirus-induced cytokine secretion and viral replication in human nasal epithelial cells. Inhal Toxicol. 2010; 22:1038–1045. PMID: 20879958.


4. Wilker EH, Preis SR, Beiser AS, Wolf PA, Au R, Kloog I, Li W, Schwartz J, Koutrakis P, DeCarli C, Seshadri S, Mittleman MA. Long-term exposure to fine particulate matter, residential proximity to major roads and measures of brain structure. Stroke. 2015; 46:1161–1166. PMID: 25908455.


5. Shah AS, Lee KK, McAllister DA, Hunter A, Nair H, Whiteley W, Langrish JP, Newby DE, Mills NL. Short term exposure to air pollution and stroke: systematic review and meta-analysis. BMJ. 2015; 350:h1295. PMID: 25810496.


6. Blank F, Stumbles PA, Seydoux E, Holt PG, Fink A, Rothen-Rutishauser B, Strickland DH, von Garnier C. Size-dependent uptake of particles by pulmonary antigen-presenting cell populations and trafficking to regional lymph nodes. Am J Respir Cell Mol Biol. 2013; 49:67–77. PMID: 23492193.


7. Tsuda A, Rogers RA, Hydon PE, Butler JP. Chaotic mixing deep in the lung. Proc Natl Acad Sci U S A. 2002; 99:10173–10178. PMID: 12119385.


8. Oberdörster G. Pulmonary effects of inhaled ultrafine particles. Int Arch Occup Environ Health. 2001; 74:1–8. PMID: 11196075.
9. Philipson K, Falk R, Svartengren M, Jarvis N, Bailey M, Bergmann R, Hofmann W, Camner P. Does lung retention of inhaled particles depend on their geometric diameter? Exp Lung Res. 2000; 26:437–455. PMID: 11033767.
10. Choi HS, Ashitate Y, Lee JH, Kim SH, Matsui A, Insin N, Bawendi MG, Semmler-Behnke M, Frangioni JV, Tsuda A. Rapid translocation of nanoparticles from the lung airspaces to the body. Nat Biotechnol. 2010; 28:1300–1303. PMID: 21057497.


11. Mohammad AK, Amayreh LK, Mazzara JM, Reineke JJ. Rapid lymph accumulation of polystyrene nanoparticles following pulmonary administration. Pharm Res. 2013; 30:424–434. PMID: 22992832.


12. Ichinose T, Yoshida S, Hiyoshi K, Sadakane K, Takano H, Nishikawa M, Mori I, Yanagisawa R, Kawazato H, Yasuda A, Shibamoto T. The effects of microbial materials adhered to Asian sand dust on allergic lung inflammation. Arch Environ Contam Toxicol. 2008; 55:348–357. PMID: 18227959.


13. Kyung SY, Yoon JY, Kim YJ, Lee SP, Park JW, Jeong SH. Asian dust particles induce TGF-β(1) via reactive oxygen species in bronchial epithelial cells. Tuberc Respir Dis (Seoul). 2012; 73:84–92. PMID: 23166540.
14. Honda A, Matsuda Y, Murayama R, Tsuji K, Nishikawa M, Koike E, Yoshida S, Ichinose T, Takano H. Effects of Asian sand dust particles on the respiratory and immune system. J Appl Toxicol. 2014; 34:250–257. PMID: 23576315.


15. Kim ST, Ye MK, Shin SH. Effects of Asian sand dust on mucin gene expression and activation of nasal polyp epithelial cells. Am J Rhinol Allergy. 2011; 25:303–306. PMID: 22186242.


16. Shin SH, Ye MK, Hwang YJ, Kim ST. The effect of Asian sand dust-activated respiratory epithelial cells on activation and migration of eosinophils. Inhal Toxicol. 2013; 25:633–639. PMID: 24044679.


17. Cohen MD, Vaughan JM, Garrett B, Prophete C, Horton L, Sisco M, Kodavanti UP, Ward WO, Peltier RE, Zelikoff J, Chen LC. Acute high-level exposure to WTC particles alters expression of genes associated with oxidative stress and immune function in the lung. J Immunotoxicol. 2015; 12:140–153. PMID: 24911330.


18. Higashisaka K, Fujimura M, Taira M, Yoshida T, Tsunoda S, Baba T, Yamaguchi N, Nabeshi H, Yoshikawa T, Nasu M, Yoshioka Y, Tsutsumi Y. Asian dust particles induce macrophage inflammatory responses via mitogen-activated protein kinase activation and reactive oxygen species production. J Immunol Res. 2014; 2014:856154. DOI: 10.1155/2014/856154. PMID: 24987712.


19. Sakamoto N, Hayashi S, Gosselink J, Ishii H, Ishimatsu Y, Mukae H, Hogg JC, van Eeden SF. Calcium dependent and independent cytokine synthesis by air pollution particle-exposed human bronchial epithelial cells. Toxicol Appl Pharmacol. 2007; 225:134–141. PMID: 17720209.


20. Lansley AB, Sanderson MJ, Dirksen ER. Control of the beat cycle of respiratory tract cilia by Ca2+ and cAMP. Am J Physiol. 1992; 263:L232–L242. PMID: 1325130.
21. Hayashi T, Kawakami M, Sasaki S, Katsumata T, Mori H, Yoshida H, Nakahari T. ATP regulation of ciliary beat frequency in rat tracheal and distal airway epithelium. Exp Physiol. 2005; 90:535–544. PMID: 15769883.


22. Abdullah LH, Conway JD, Cohn JA, Davis CW. Protein kinase C and Ca2+ activation of mucin secretion in airway goblet cells. Am J Physiol. 1997; 273:L201–L210. PMID: 9252557.
23. Davis CW. Regulation of mucin secretion from in vitro cellular models. Novartis Found Symp. 2002; 248:113–125. discussion 125-131, 277-282. PMID: 12568491.


24. Sakamoto Y, Ishijima M, Kaneko H, Kurebayashi N, Ichikawa N, Futami I, Kurosawa H, Arikawa-Hirasawa E. Distinct mechanosensitive Ca2+ influx mechanisms in human primary synovial fibroblasts. J Orthop Res. 2010; 28:859–864. PMID: 20108315.
25. Murata N, Ito S, Furuya K, Takahara N, Naruse K, Aso H, Kondo M, Sokabe M, Hasegawa Y. Ca2+ influx and ATP release mediated by mechanical stretch in human lung fibroblasts. Biochem Biophys Res Commun. 2014; 453:101–105. PMID: 25256743.
26. Wakui M, Osipchuk YV, Petersen OH. Receptor-activated cytoplasmic Ca2+ spiking mediated by inositol trisphosphate is due to Ca2+-induced Ca2+ release. Cell. 1990; 63:1025–1032. PMID: 1701691.
27. Ehrlich BE, Kaftan E, Bezprozvannaya S, Bezprozvanny I. The pharmacology of intracellular Ca2+-release channels. Trends Pharmacol Sci. 1994; 15:145–149. PMID: 7754532.
28. Criddle DN, Sutton R, Petersen OH. Role of Ca2+ in pancreatic cell death induced by alcohol metabolites. J Gastroenterol Hepatol. 2006; 21(Suppl 3):S14–S17. PMID: 16958662.
29. Wiegman CH, Michaeloudes C, Haji G, Narang P, Clarke CJ, Russell KE, Bao W, Pavlidis S, Barnes PJ, Kanerva J, Bittner A, Rao N, Murphy MP, Kirkham PA, Chung KF, Adcock IM. COPDMAP. Oxidative stress-induced mitochondrial dysfunction drives inflammation and airway smooth muscle remodeling in patients with chronic obstructive pulmonary disease. J Allergy Clin Immunol. 2015; 136:769–780. PMID: 25828268.


30. Park L, Wang G, Moore J, Girouard H, Zhou P, Anrather J, Iadecola C. The key role of transient receptor potential melastatin-2 channels in amyloid-β-induced neurovascular dysfunction. Nat Commun. 2014; 5:5318. PMID: 25351853.


31. Shekh K, Khan S, Jena G, Kansara BR, Kushwaha S. 3-Aminobenzamide--a PARP inhibitor enhances the sensitivity of peripheral blood micronucleus and comet assays in mice. Toxicol Mech Methods. 2014; 24:332–341. PMID: 24568202.
32. Choi SY, Kim YH, Lee YK, Kim KT. Chlorpromazine inhibits store-operated calcium entry and subsequent noradrenaline secretion in PC12 cells. Br J Pharmacol. 2001; 132:411–418. PMID: 11159689.


33. Mori Y, Kajimoto T, Nakao A, Takahashi N, Kiyonaka S. Receptor signaling integration by TRP channelsomes. Adv Exp Med Biol. 2011; 704:373–389. PMID: 21290307.


34. Takahashi N, Kozai D, Kobayashi R, Ebert M, Mori Y. Roles of TRPM2 in oxidative stress. Cell Calcium. 2011; 50:279–287. PMID: 21616534.


35. Nazıroğlu M, Özgül C, Çelik Ö, Çiğ B, Sözbir E. Aminoethoxydiphenyl borate and flufenamic acid inhibit Ca2+ influx through TRPM2 channels in rat dorsal root ganglion neurons activated by ADP-ribose and rotenone. J Membr Biol. 2011; 241:69–75. PMID: 21509529.
36. Roberge S, Roussel J, Andersson DC, Meli AC, Vidal B, Blandel F, Lanner JT, Le Guennec JY, Katz A, Westerblad H, Lacampagne A, Fauconnier J. TNF-α-mediated caspase-8 activation induces ROS production and TRPM2 activation in adult ventricular myocytes. Cardiovasc Res. 2014; 103:90–99. PMID: 24802330.


37. Berridge MJ, Bootman MD, Roderick HL. Calcium signalling: dynamics, homeostasis and remodelling. Nat Rev Mol Cell Biol. 2003; 4:517–529. PMID: 12838335.


38. Lei B, Hitomi H, Mori T, Nagai Y, Deguchi K, Mori H, Masaki T, Nakano D, Kobori H, Kitaura Y, Nishiyama A. Effect of efonidipine on TGF-β1-induced cardiac fibrosis through Smad2-dependent pathway in rat cardiac fibroblasts. J Pharmacol Sci. 2011; 117:98–105. PMID: 21897055.


39. Ohyama T, Sato K, Kishimoto K, Yamazaki Y, Horiguchi N, Ichikawa T, Kakizaki S, Takagi H, Izumi T, Mori M. Azelnidipine is a calcium blocker that attenuates liver fibrosis and may increase antioxidant defence. Br J Pharmacol. 2012; 165:1173–1187. PMID: 21790536.


40. Mishima K, Maeshima A, Miya M, Sakurai N, Ikeuchi H, Hiromura K, Nojima Y. Involvement of N-type Ca2+ channels in the fibrotic process of the kidney in rats. Am J Physiol Renal Physiol. 2013; 304:F665–F673. PMID: 23324177.
41. Xu R, Li Q, Zhou XD, Perelman JM, Kolosov VP. Oxidative stress mediates the disruption of airway epithelial tight junctions through a TRPM2-PLCγ1-PKCα signaling pathway. Int J Mol Sci. 2013; 14:9475–9486. PMID: 23629676.
42. Horng T. Calcium signaling and mitochondrial destabilization in the triggering of the NLRP3 inflammasome. Trends Immunol. 2014; 35:253–261. PMID: 24646829.


43. Li R, Kou X, Geng H, Xie J, Yang Z, Zhang Y, Cai Z, Dong C. Effect of ambient PM(2.5) on lung mitochondrial damage and fusion/fission gene expression in rats. Chem Res Toxicol. 2015; 28:408–418. PMID: 25560372.


44. Dellinger B, Pryor WA, Cueto R, Squadrito GL, Hegde V, Deutsch WA. Role of free radicals in the toxicity of airborne fine particulate matter. Chem Res Toxicol. 2001; 14:1371–1377. PMID: 11599928.


45. Kinnula VL, Fattman CL, Tan RJ, Oury TD. Oxidative stress in pulmonary fibrosis: a possible role for redox modulatory therapy. Am J Respir Crit Care Med. 2005; 172:417–422. PMID: 15894605.
46. Park JW, Yoon JY, Kim YJ, Kyung SY, Lee SP, Jeong SH, Moon C. Extracellular signal-regulated kinase (ERK) inhibition attenuates cigarette smoke extract (CSE) induced-death inducing signaling complex (DISC) formation in human lung fibroblasts (MRC-5) cells. J Toxicol Sci. 2010; 35:33–39. PMID: 20118622.


47. Park SA, Kim MJ, Park SY, Kim JS, Lee SJ, Woo HA, Kim DK, Nam JS, Sheen YY. EW-7197 inhibits hepatic, renal, and pulmonary fibrosis by blocking TGF-β/Smad and ROS signaling. Cell Mol Life Sci. 2015; 72:2023–2039. PMID: 25487606.


48. Janssen LJ, Mukherjee S, Ask K. Calcium homeostasis and ionic mechanisms in pulmonary fibroblasts. Am J Respir Cell Mol Biol. 2015; 53:135–148. PMID: 25785898.


Fig. 1
PM10-induced [Ca2+]i signal by extracellular Ca2+ in human lung fibroblast MRC5 cells.
(A) Changes in [Ca2+]i induced by 50 µg/mL PM10 in 1 mM Ca2+ medium (black line) and in Ca2+-free medium (gray line). Top bars indicate the extracellular solutions applied to MRC5 cells. (B) 50 µg/mL PM10-induced [Ca2+]i signals were completely abolished by 10 µM BAPTA-AM. Traces shown were obtained from average signal except the stimulation with PM10 only. (C) ΔCa2+ was calculated at the indicated dotted line. Results are presented as mean±SEM. *p values of <0.01 were considered significant. (D) Cells were stimulated with the presence of La3+. Traces shown were obtained from average signal except the stimulation with PM10 only. (E) Results are presented as mean±SEM and *p values of <0.01 were considered significant. (F) Analysis of size distribution of dust particles.
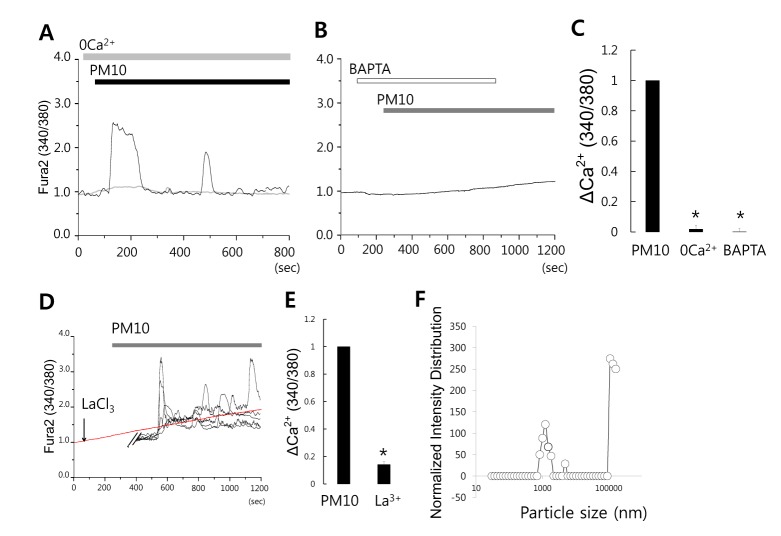
Fig. 2
PM10-induced [Ca2+]i signal is dependent on the PLC/IP3 receptor pathway.
(A) Changes in [Ca2+]i induced by 50 µg/mL PM10 in 1 mM Ca2+ medium. (B) 50 µg/mL PM10-induced [Ca2+]i signals were prevented in MRC5 cells by 20 mM caffeine (an IP3R antagonist). 50 µg/mL (C) PM10-induced [Ca2+]i signaling in the presence of PLC inhibitor 5 µM U73122 or its inactive analog 5 µM U73343. The top bars indicate the types of extracellular solutions applied to cells. Traces shown were obtained from average signal except the co-stimulation with PM10 and U73343. (D) ΔCa2+ was calculated at the indicated dotted line. Results are presented as mean±SEM. *p values of <0.01 were considered significant.
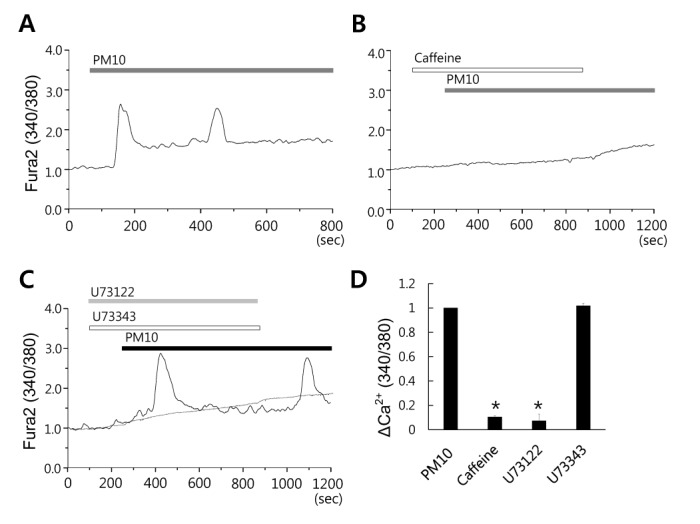
Fig. 3
PM10-induced [Ca2+]i signal is attenuated by inhibition of oxidative pathways.
(A) Changes in [Ca2+]i induced by 50 µg/mL PM10. (B) 50 µg/mL PM10 induced [Ca2+]i signaling in the presence of (A) 5 mM NAC (an anti-oxidant), (C) 10 µM 3-AB (a PARP-1 inhibitor), or (D) 10 µg/mL CLP. Top bars indicate the extracellular solution type applied. Traces shown were obtained from average signal. (E) ΔCa2+ was calculated at the indicated dotted line. Results are presented as mean±SEM. *p values of <0.01 were considered significant.

Fig. 4
PM10-induced [Ca2+]i signal is required for the involvement of TRPM2 activation.
(A) Changes in [Ca2+]i induced by 50 µg/mL PM10. 50 µg/mL PM10 induced [Ca2+]i signaling in the presence of TRPM2 inhibitors: (B) 75 µM 2-APB, (C) 10 µM CLZ, or (D) 10 µM ACA. Top bars indicate the extracellular solution type applied to cells. Traces shown were obtained from average signal. (E) ΔCa2+ was calculated at the indicated dotted line. Results are presented as mean±SEM, and *p values of <0.01 were considered significant.
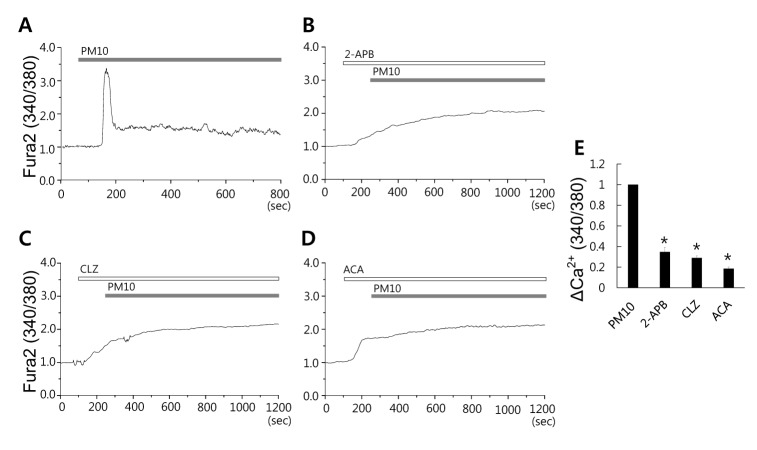
Fig. 5
PM10 induced ROS production and ROS levels were reduced in the presence of TRPM2 blockers.
The fluorescence of H2DCFDA was visualized by confocal laser scanning microscopy and quantified. (A) ROS production induced by 50 µg/mL PM10 and the effects of pretreating the TRPM2 blockers, 10 µM CLZ and 10 µM ACA. ROS images were taken within 60 min. (B) Results are presented as mean±SEM and *p values of <0.01 were considered significant. (C) ROS production in the presence of TRPM2 blockers CLZ and ACA. *p values of <0.01 were considered significant.
