Abstract
Angelicae Gigantis Radix (AGR, Angelica gigas) has been used for a long time as a traditional folk medicine in Korea and oriental countries. Decursinol angelate (DCA) is structurally isomeric decursin, one of the major components of AGR. This study was performed to confirm whether DCA augments pentobarbital-induced sleeping behaviors via the activation of GABAA-ergic systems in animals. Oral administration of DCA (10, 25 and 50 mg/kg) markedly suppressed spontaneous locomotor activity. DCA also prolonged sleeping time, and decreased the sleep latency by pentobarbital (42 mg/kg), in a dose-dependent manner, similar to muscimol, both at the hypnotic (42 mg/kg) and sub-hypnotic (28 mg/kg) dosages. Especially, DCA increased the number of sleeping animals in the sub-hypnotic dosage. DCA (50 mg/kg, p.o.) itself modulated sleep architectures; DCA reduced the counts of sleep/wake cycles. At the same time, DCA increased total sleep time, but not non-rapid eye movement (NREM) and rapid eye movement (REM) sleep. In the molecular experiments. DCA (0.001, 0.01 and 0.1 µg/ml) increased intracellular Cl- influx level in hypothalamic primary cultured neuronal cells of rats. In addition, DCA increased the protein expression of glutamic acid decarboxylase (GAD65/67) and GABAA receptors subtypes. Taken together, these results suggest that DCA potentiates pentobarbital-induced sleeping behaviors through the activation of GABAA-ergic systems, and can be useful in the treatment of insomnia.
The research to find alternative compounds for the treatment of insomnia has progressed in the past decades. Many kinds of herbs such as St, John's wort, Kava Kava, Valerian and Passion flower have been introduced in European countries [1]. Herbs as sleep aids are becoming more popular as alternative medicines.
γ-Aminobutyric acid (GABA)A-ergic transmission plays an important role in the mediation of sleep behaviors. GABA is synthesized from glutamate by glutamic acid decarboxylase (GAD) and higher levels of GABA contains in the inhibitory neurons [2]. GABA is the main inhibitory neurotransmitter in the central nervous systems (CNS) of mammalians [3]. GABA binds the ionotropic and metabotropic GABA receptors on the pre- and post-synaptic nerves, resulting in hyperpolarization of neuronal cells in CNS [456]. Depending on the configuration of the structural subunits, it determines the pharmacological properties of the GABA receptors [7]. α1-Subunits in GABAA receptors mediate sedation, amnesia, and ataxic effects of benzodiazepine (BZ), whereas α2-and α3-subunits are involved in their anxiolytic-like and muscle-relaxing actions. α5-Subunits are involved in at least some of the memory impairment caused by BZ [8].
The dried root of Korean Angelicae Gigantis Radix (AGR, Angelica gigas), known by the Korean name as ‘Cham-dang-gui’ has been used as a medicinal herb in Korea [9]. This herb been used traditionally for the treatment of psychosomatic diseases such as excess stress, anxiety, depression, pain and insomnia [1011121314]. So far, recent studies have been shown that the essential oil components of AGR exhibited anxiolytic-like effects in rodents tests [15]. It was reported that Japanese Angelica root extract reversed stress-induced sleep-reduction in pentobarbital sleeping animals through the inhibition of the central noradrenergic or the activation of GABAA receptors in mice [16]. Decursinol angelate (DCA, one of coumarin derivatives from AGR, Fig. 1) has been shown similar pharmacological activities of the extract of AGR such as and anti-amyloid β protein aggregation, anti-amnesic activity, anti-nociceptive activity and protective activity of neurotoxicity [17181920]. Therefore, we investigated whether DCA augments pentobarbital-induced sleeping behaviors via the activation of GABAA-ergic systems, and the sleep architectures of electroencephalogram (EEG) induced by DCA were analyzed. These experiments have been more focused on whether DCA could be a candidate of insomnia treatment.
DCA was gifted from the professor Jae-Seon Kang who works at the College of Pharmacy, Kyungsung University of Pharmacy. Diazepam and pentobarbital sodium were purchased from Hanlim Pharm. Co., Ltd. (Seoul, Korea) and Samjin Pharm. (Seoul, Korea), respectively. Fetal bovine serum (FBS), Dulbecco's Modified Eagle Medium (DMEM), Neurobasal A Medium, Penicillin–Streptomycin and Trypsin–EDTA were obtained from GIBCO (GrandIsland, NY, USA). The Cl- sensitive fluorescence probe N-(ethoxycarbonylmethyl)-6-methoxyquinolinium bromide (MQAE) and cytosine β-D-arabinofuranoside were obtained from Sigma-Aldrich Co. (St. Louis, MO, USA). Muscimol (Tocris Bioscience, Bristol, UK) and Dimethyl sulfoxide (Amresco Solon, Ohio, USA) were purchased, respectively. The specific rabbit polyclonal antibodies against GABAA receptors subunits or GAD65/67 and the corresponding conjugated anti-rabbit immunoglobulin G-horseradish peroxidase were purchased from Abcam Inc. (Cambridge, UK). Chemiluminescent HRP substrate was obtained from Millipore Co. (Billerica, MA, USA).
All animals (ICR mice weighing 20~24 g and Sprague-Dawley rats weighing 220~250 g) were purchased from Samtako (Osan, Korea). All animals were housed in acrylic cages (45×60×23 cm) and were kept at least 1 week for acclimation time. The room temperature was maintained at 22±2℃ and at the relative humidity (50~52%) with a 12 h light/dark cycle (lights on at 7:00) in the animal room. Food and water were available ad libitum. All the behavioral experiments were performed between 10:00 and 17:00. Experiments were carried out in accordance with the Principle of Laboratory Animal Care (NIH publication No. 85-23, revised 1985) and the Animal Care and Use Guidelines of Chungbuk National University, Korea.
Spontaneous locomotor activity was measured automatically with a tilting-type ambulometer (AMB-10, O'Hara, Tokyo, Japan). Each mouse was placed in the activity cage (20 cm in diameter and 18 cm in height) and after an adaptation period of 10 min. DCA (10, 25 and 50 mg/kg). Diazepam (2.0 mg/kg) were dissolved in 0.9% physiological saline. DCA (10, 25 and 50 mg/kg) and diazepam (2.0 mg/kg) were orally administered before 60 min and 30 min of the locomotor measurement, respectively. The ambulatory of activity was measured automatically for 1 h after the administration of the agents [21].
Animals were fasted for a day. Pentobarbital sodium (Hanlim Pharm. Co., Ltd., Korea) was diluted in 0.9% physiological saline, and administered to each mouse intraperitoneally to induce sleep. Muscimol and DCA were suspended in 0.01% DMSO. All experiments were carried out between 13:00 and 17:00. DCA (10, 25 and 50 mg/kg) and muscimol (0.2 mg/kg) were orally administered before 1 h and 30 min of pentobarbital (42 mg/kg, i.p.) administration, respectively, to the mice. The animals that stopped the movement after pentobarbital injection was moved carefully to another cage. The sleep latency was recorded as elapse time between pentobarbital injection and loss of the reflex. The sleep was recorded as elapse time between the loss and recovery righting movement. The mice which failed to induce sleep within 15 min after administration of pentobarbital were except from the experiments [2223]. The awakening behaviors characterized by righting of the animal, were observed constantly.
Pentobarbital (50 mg/kg) was i.p. injected to the rats of the surgical anesthesia. The animals were then placed on a pad, and then secured in the stereotaxic apparatus under aseptic conditions. An incision was made in the part of the abdomen's midline, caudal to the cartilage. One hole for positioning the electrodes was drilled in the skull surface (A: 2.0 [Bregma], L: 1.5; P: 7.0 [Bregma], L: 1.5 contra-lateral) [24]. The periosteum was removed by sterile cotton. Lines from the transmitter were connected subcutaneously to the hole that made in the skull. The electrodes lines were anchored to the skull with screws and dental cement. The transmitter (Data Sciences International TA11CTA-F40, MN, USA) was placed in the skin. It was attached to the skin with 3 sutures for stabilization. It was fixed by a silk 4–0 suture. The antibiotic (i.p.) (5 million unit potassium penicillin-G Injection, Kunwha, KOREA) was given to the rats for a better recovery.
Following 7 days post-surgical recovery, rats were divided into control and DCA, with 8 rats in each group. DCA (50 mg/kg) was suspended, dissolved in 0.01% DMSO, and administered orally 1 hour prior to the measurement. Telemetric recording of cortical EEG activity were designed with a slight modification as previous reports [25]. The setting of EEG signal was amplified and arranged of 0.5~20.0 Hz in the transmitters. It was additionally set up −0.5/+0.5 volts per/units ×2, and was controlled by a Data Sciences International analog converter. The signals were passed over to an AD converter (Eagle PC30, USA) and then saved by a computer. The signals digitize the collected data to the rate of 128 Hz by an AD converter. The recordings marked graphically can be expressed by the computer. An on-line fast Fourier transformation (FFT) was performed on the EEG data at 2 sec intervals during data acquisition (256 samples) after a Hanning window treatment. The FFT analysis generated power density values from 0.0 to 20.0 Hz. The FFT data were further averaged in the range of 0 to 20 Hz for every 10 sec. The sleep data and FFT results were saved to the hard disk every 10 sec for additional offline analysis. Movement of the animal in relation to the telemetry receiver generated transistor-transistor logic (TTL) pulses that were collected and counted as a measure of activity. Data were recorded between 11:00 and 15:00. The activity of the each rat was recorded simultaneously.
The amount of time in wakefulness, NREM sleep and REM sleep were determined from the digitized data at 10 sec intervals using professional animal sleep analysis software SleepSign2.1 (KISSEI Comtec Co Ltd, Matsumoto, Japan). The amount of time in wakefulness, non-rapid eye movement (NREM) sleep, and rapid eye movement (REM) sleep were classified every 10 sec period [26]. EEG signals according to the followings were classified by the software: Wakefulness was identified by a high-frequency, and recoding of NREM was focused on the presence of spindles interspersed with slow waves. EEG power during REM sleep is significantly reduced in lower frequency δ-wave (0.75~4.0 Hz), and is increased in the range of theta-wave activity (5.0~9.0 Hz, peak at 7.5 Hz) for 6 hours period. We further calculated the time of each recording spent in each sleep–wake states (wakefulness, NREM sleep and REM sleep). The absolute EEG power was calculated during wakefulness, NREM, and REM in 0.5 Hz bins from 0.5 to 20 Hz for the entire 6 h reading. EEG power density in 3 selected frequency bands for wakefulness, NREM sleep and REM sleep δ-wave (0.75~4.0 Hz), θ-wave (4.0~8.0 Hz) and α-wave (8.0~13.0 Hz) were subsequently evaluated.
Primary cultures of hypothalamus cells were prepared from 8 days old SD rats [2728]. Hypothalamus cells were plated (1.0×105 cells per well) in 96-well microplates dishes coated with poly-L-lysine (50 mg/mL; Sigma, St. Louis, MO, USA). and were cultured DMEM and neurobasal A media 10% heat-inactivated fetal bovine serum, gentamicin (100 mg/mL), glutamine (2.0 mM), antibiotic-antimycotic solution (10 mg/mL; Sigma), and potassium chloride (25 mM). After seeding the cells, incubated for 6~9 days in a humidified 5% CO2/95% air atmosphere at 37℃. Cytosine arabinofuranoside (final concentration, 10 mM; Sigma, USA) was added after 18~24 h of culture to inhibit the replication of non-neuronal cells. The intracellular chloride ion ([Cl–]i) of hypothalamic neuronal cells of rats was estimated using Cl– sensitive fluorescence probe N-(ethoxy-carbonylmethyl)-6-methyoxyquionolinium bromide (MQAE) according to the method of West and Molloy, with a slight modification [29]. After overnight incubated, the primary cultured cells were washed three times in the buffer (pH 7.4) which contained 2.4 mM HPO42–, 0.6 mM H2PO4–, 10 mM HEPES, 10 mM D-glucose and 1 mM MgSO4. Fluorescence was monitored using excitation wavelength 320 nm and emission wavelength 460 nm, respectively, in a microplate reader (Spectra Max M2e Multi-Mode, PA, USA) as described by Wagner [30]. The data is presented as the relative fluorescence F0/F, where F0 is the fluorescence without Cl– ions and F is the fluorescence as a function of each sample. The F0/F values were directly proportional to [Cl–]i.
The groups were administered orally to DCA (10, 25 and 50 mg/kg) and diazepam (0.2 mg/kg) before 1 h and 30 min respectively injected to all mice (0.1 ml/10 g). The mice were decapitated and separated hypothalamus from brain on ice. Mouse hypothalamic tissue was homogenized with 4℃ lysis buffer. The extracts were centrifuged at 13,000 × g for 20 min at 4℃. Then, supernatant was recovered, the samples were stored at –20℃. Equal amounts of protein were loaded and electrophoresed on 10% SDS–polyacrylamide gel. Proteins were then transferred to PVDF membranes (Hybond-P, GE Healthcare, Amersham, UK) using a semidry transfer system. The blots were blocked for 1 h at room temperature with 5% (w/v) BSA [applied to all primary antibodies except for glyceraldehyde 3-phosphate dehydrogenase (GAPDH)] in tris-buffered saline solution (TBS) containing 0.1% Tween-20. Both specific rabbit polyclonal antibodies against GABAA receptors subunits and rabbit anti-GAD65/67 polyclonal antibody at the appropriate dilution in TBST and 5.0% BSA (1:2,500 for all the primary antibodies used) were incubated for overnight at 4℃. Blots were washed and incubated with the horseradish peroxidase-conjugated (HRP) secondary antibody (1:3,000 for goat anti-rabbit IgG) in TBST for 4 h at room temperature. The chemiluminescent substrate for the secondary antibody was developed using the ECL detection system (Roche Diagnostics, Mannheim, Germany) [31].
All statistical analyses were calculated by Sigma Stat software (SPSS Inc., Chicago, IL, USA). The results were expressed as mean±S.E.M for the number of experiments. The significance of the effects of the compounds was assessed using analysis of variance (ANOVA). Statistical significances were compared between treated group and control group by Holm-Sidak method. For the sub-hypnotic pentobarbital dosage experiment, Chi-square test was used to compare the control. Each experiment was repeated at least three times and yielded comparable results. Values with p<0.05 were considered significant.
DCA (10, 25 and 50 mg/kg) was administered to the mice. Locomotor activity was decreased, in a dose-dependent manner, by DCA. Locomotor activities were decreased by approximately 61.8% at 50 mg/kg, 44.3% at 25 mg/kg, and 30.0% at 10 mg/kg, respectively. The locomotor activity by diazepam (2.0 mg/kg) as a positive control was also decreased by approximately 27.2%, compared with that of the control (Fig. 2).
DCA (25 and 50 mg/kg) significantly decreased the sleep latency of sleep and increased dose-dependently total sleep time in pentobarbital induced sleeping mice. Muscimol (0.2 mg/kg, p.o.) as a positive control also augmented the increase of the sleeping time and the decrease of the sleep latency in pentobarbital (42 mg/kg)-induced sleeping mice. The time of sleep onset to oral administration with DCA at 10, 25 and 50 mg/kg were decreased by approximately 97.4%, 86.1% and 77.9%, respectively. Muscimol also decreased by approximately 77.3%, compared with that of the control (Fig. 3A). The time of sleep duration by oral administration with DCA at 10, 25 and 50 mg/kg were increased by approximately 113.0%, 121.1% and 129.8%, respectively. Muscimol showed similar effects and increased sleeping time by approximately 137.3%, compared with that of the control (Fig. 3B).
Oral administration of DCA increased the rate of sleep onset and the duration of sleep time induced by sub-hypnotic dosage of pentobarbital (28 mg/kg. i.p.). Muscimol also increased the rate of sleep onset and prolonged the sleep time significantly (Table 1). It was found that DCA at all dose treated to the mice showed significant increase in sleeping behaviors induced by pentobarbital. The time of sleep duration to sub-hypnotics dosage with DCA at 25 and 50 mg/kg were increased by approximately 152.3% and 195.0%, respectively. Muscimol increased sleep duration by approximately 157.5%, compared with that of the control (Table 1).
Sleep-wake cycles were observed in rats during 6 h after administration of DCA (50 mg/kg, p.o.), DCA decreased the count of sleep-wake cycles approximately 75.6%, compared with that of the control (Fig. 4).
The oral administration of DCA (50 mg/kg) significantly decreased wakefulness, and increased total sleep during 6 hours in EEG recording. DCA decreased wakeful time by approximately 68.5%, and increased total sleep time by approximately 108.2%, compared with that of the control (Fig. 5). However, REM and NREM sleep was not increased.
DCA (50 mg/kg) decreased δ-wave and increased θ-wave by approximately 83.2% and 134.6%, respectively, in wakefulness. DCA increased δ-waves in REM and NREM sleep by approximately 109.9% and 106.5%. In addition, DCA decreased θ-waves in REM and NREM sleep, by approximately 79.7% and 78.9%, respectively, compared with that of the control (Fig. 6). It is suggested that slow waves such as δ-waves were increased during sleep.
Intracellular chloride ion influx in primary cultured hypothalamic neuronal cells was measured. The data is represented as the relative fluorescence F0/F, where F0 is the fluorescence without chloride ions and F is the fluorescence as a function of each sample. The F0/F values were directly proportional to intracellular chloride ion concentration. DCA (0.01 and 0.1 µg/ml) significantly increased chloride ion influx in primary cultured cerebellar granule cells by approximately 115.3%, compared with that of the control (Fig. 7). Pentobarbital (10 µM) also increased the influx of Cl–(Fig. 7).
Mice were sacrificed to examine the effect of these drugs on the abundance of GAD65/67 in the hypothalamic tissue. DCA (50 mg/kg) overexpressed GAD65/67 in the plasma membrane of hypothalamic tissue by approximately 164.8%, compared with that of the controls tissue (Fig. 8).
The DCA increased the expression of GABAA subunit (α4, α5, β1, β2 and γ3) in hypothalamic sample treated with 50 mg/kg were approximately 111.1%, 120.9%, 129.6%, 123.7% and 110.6% respectively. The protein overexpressions (α1, α4, β1, β2, γ3) by PENT as a positive control were also increased by approximately 124.8%, 135.6%, 112.9%, 109.9% and 145.1% respectively, compared with that of the control. DCA did not influence the amounts of α1 and α3 subunit in the GABAA receptor (Fig. 9). Pentobarbital showed increased all protein levels except α3 and α5 subunits.
DCA is one of major components of AGR, have been reported as anti-amnesic [32], analgesic, anti-cancer, anti-bacterial, neuroprotective and protein kinase C (PKC) activating activities from in vivo and in vitro experiment [3334353637]. From these experiments, oral administration of DCA suppressed spontaneous locomotor activity. We considered that DCA would be inhibitory in the CNS. DCA also prolonged sleeping time, and decreased the sleep latency by pentobarbital (42 mg/kg), in a dose-dependent manner, similar to muscimol, both at the hypnotic (42 mg/kg) and sub-hypnotic (28 mg/kg) dosages. Especially, DCA increased the number of sleeping animals in the sub-hypnotic dosage. It was confirmed that DCA potentiated pentobarbital-induced sleeping behaviors.
From the independent experiments using rats, DCA (50 mg/kg, p.o.) itself modulated sleep architectures; DCA reduced the counts of sleep/wake cycles in rats. Spectral EEG analysis technique of sleep/wake cycles can be used as a tool for insomnia treatment. At the same time, DCA increased total sleep time and non-rapid eye movement (NREM) and rapid eye movement (REM) sleep. Sleep stages are generally divided into three stages including wake, non-rapid eye movement (NREM) sleep and rapid eye movement (REM) sleep. The NREM phase is further divided into several stages according to deeper sleep. These stages include N1, N2 and N3. During N3, it is considered to be in the deep or delta wave sleep. The sleep cycle first starts with NREM sleep and then followed by REM sleep [38]. About 25 percent of sleep is spent in an REM sleep and the remaining 75 percent is for the NREM sleep. During an 8 h sleep, REM sleep repeated about 4 to 6 times. Each stage of sleep possess different frequency. Especially α-wave appears most in wakefulness and δ-wave appears most in sleep stage. DCA itself reduced sleep/wakefulness cycles, increased total sleep time, non-rapid eye movement (NREM) and rapid eye movement (REM) sleep in EEG. In the power density of NREM sleep and REM sleep, δ-wave increased and θ-wave decreased. It has been known that several GABAA agonists decrease EEG δ activity in NREM [3940]. Diazepam (DZ) is known as benzodiazepines acting on GABAA receptors and increasing in NREM sleep without change of REM sleep [41], but it decreases in δ wave activity [42]. Indeed, remedies for the treatment of insomnia should increase both REM and NREM sleep. From these experiments, it is suggested that DCA could enhance total sleep and NREM sleep in rats, reducing sleep/wake cycles.
Based on in vivo experiments, we are more interested in whether sleep potentiation of DCA in pentobarbital induced sleep animals is mediated by the activation of GABAA-ergic systems. From the first in vitro experiments, we found that DCA increased intracellular Cl- influx in primary cultured hypothalamic cells of rats, resulting in hyperpolarization of neuronal cells in the CNS. These effects can be caused by the increased levels of GABA in the neuronal cells. We found that DCA activated GAD65/67, an enzyme synthesizing GABA. In more detail, when the released GABA is coupled to the postsynaptic GABAA receptors, Cl– ion channels open and the intracellular influx of Cl– ion increases, and the cells become hyperpolarized state. GABA receptors is remarkable because it is the site of action for anxiolytic benzodiazepines and other sedative hypnotics which are generally used in the world. GABAA receptors are blocked by the convulsive bicuculline, a competitive antagonist at the GABA binding site. In addition, also plays a crucial role in sleep [43]. GAD65/67 is the rate-limiting enzyme in GABA biosynthesis which also plays an important role in maintaining GABA level in the brain [44]. GABA is released to the synapse which is the extracellular space existing between the neurons. The amount of endogenous GABA in CNS may activates GABAA-ergic transmission. DCA increased protein expression of GAD65/67 and intracellular Cl- influx, and then GABAA-ergic transmission would be activated. GABAA receptors lead to induce sleep by increasing the intracellular influx of chloride ion and occurring a hyperpolarization of cell membrane through binding site of GABA.
It has been well known that the activation of GABAA receptors shows sedative, hypnotic and anticonvulsant effects. Notably, pharmacological manipulation of GABAA receptors has differential effects on sleep onset, sleep maintenance and insomnia. DCA induced over-expression of GABAA receptors subunits in the hypothalamic neuronal tissues. Especially, benzodiazepine drugs have been used extensively for the treatment of insomnia. These drugs act through potentiating of the effects of the inhibitory neurotransmitter of GABA, binding to a specific site on the GABAA receptors to produce all.osteric enhancement of anion flux through this ligand-gated chloride channel [45].
All subunits which we have tested except for α3- subunits were over-expressed by DCA. Previous studies have shown α1 subunit associated with sedation [4647], the α2/3 subunits associated with anxiety [4849], and the α5 subunit associated with temporal and spatial memory [5051]. DCA, non-selectively activated the subunits of GABAA receptors in these experiments. According to previous reports, the expression levels vary throughout the brain and the composition of subunits influences the functional and pharmacological properties of the receptor, as well as its trafficking and synaptic localization [52]. We suggest that DCA may non-selectively activate the subunits of GABAA receptors. The activation of GABAA receptors by DCA seem unlikely with those of diazepam, as diazepam showed high protein expression in all subunits except α3 and α5.
DCA prolonged pentobarbital-induced sleep behaviors, and modulated sleep architectures in animals. These effects could be caused by the increased of Cl– influx in the primary cerebellar cell culture and activation of GAD65/67. In addition, DCA activated GABAA receptors subunits. Our in vivo and in vitro experiments are suggested that DCA prolonged sleep behaviors via GABAA-ergic systems DCA would be useful for the treatment of insomnia.
ACKNOWLEDGEMENTS
This work was supported by the research grant of the Chungbuk National University in 2014.
Notes
References
1. Kim CS, Han JY, Kim SH, Hong JT, Oh KW. Herbs for the treatment of insomnia. Biomol Ther. 2011; 19:274–281.


2. Jiang X, Yu J, Shi Q, Xiao Y, Wang W, Chen G, Zhao Z, Wang R, Xiao H, Hou C, Feng J, Ma Y, Shen B, Wang L, Li Y, Han G. Tim-3 promotes intestinal homeostasis in DSS colitis by inhibiting M1 polarization of macrophages. Clin Immunol. 2015; 160:328–335. PMID: 26208474.


3. Pu L, Xu N, Xia P, Gu Q, Ren S, Fucke T, Pei G, Schwarz W. Inhibition of activity of GABA transporter GAT1 by δ-opioid receptor. Evid Based Complement Alternat Med. 2012; 2012:818451. PMID: 23365600.
4. Hajak G, Rodenbeck A, Voderholzer U, Riemann D, Cohrs S, Hohagen F, Berger M, Rüther E. Doxepin in the treatment of primary insomnia: a placebo-controlled, double-blind, polysomnographic study. J Clin Psychiatry. 2001; 62:453–463. PMID: 11465523.
5. Barnard EA, Skolnick P, Olsen RW, Mohler H, Sieghart W, Biggio G, Braestrup C, Bateson AN, Langer SZ. International Union of Pharmacology. XV. Subtypes of gamma-aminobutyric acidA receptors: classification on the basis of subunit structure and receptor function. Pharmacol Rev. 1998; 50:291–313. PMID: 9647870.
6. McKernan RM, Whiting PJ. Which GABAA-receptor subtypes really occur in the brain? Trends Neurosci. 1996; 19:139–143. PMID: 8658597.


7. Verdoorn TA, Draguhn A, Ymer S, Seeburg PH, Sakmann B. Functional properties of recombinant rat GABAA receptors depend upon subunit composition. Neuron. 1990; 4:919–928. PMID: 1694446.


8. Macdonald RL, Kelly KM. Mechanisms of action of currently prescribed and newly developed antiepileptic drugs. Epilepsia. 1994; 35(Suppl 4):S41–S50.


9. Konoshima M, Chi HJ, Hata K. Coumarins from the root of Angelica gigas Nakai. Chem Pharm Bull (Tokyo). 1968; 16:1139–1140. PMID: 5706829.


10. Lam HW, Lin HC, Lao SC, Gao JL, Hong SJ, Leong CW, Yue PY, Kwan YW, Leung AY, Wang YT, Lee SM. The angiogenic effects of Angelica sinensis extract on HUVEC in vitro and zebrafish in vivo. J Cell Biochem. 2008; 103:195–211. PMID: 17497682.
11. Zhao RJ, Koo BS, Kim GW, Jang EY, Lee JR, Kim MR, Kim SC, Kwon YK, Kim KJ, Huh TL, Kim DH, Shim I, Yang CH. The essential oil from Angelica gigas NAKAI suppresses nicotine sensitization. Biol Pharm Bull. 2005; 28:2323–2326. PMID: 16327174.


12. Deng S, Chen SN, Yao P, Nikolic D, van Breemen RB, Bolton JL, Fong HH, Farnsworth NR, Pauli GF. Serotonergic activity-guided phytochemical investigation of the roots of Angelica sinensis. J Nat Prod. 2006; 69:536–541. PMID: 16643021.


13. Han DS. Pharmacognosy. Seoul: Dongmyungsa Press;1992.
14. Yeung WF, Chung KF, Poon MM, Ho FY, Zhang SP, Zhang ZJ, Ziea ET, Wong Taam V. Prescription of chinese herbal medicine and selection of acupoints in pattern-based traditional chinese medicine treatment for insomnia: a systematic review. Evid Based Complement Alternat Med. 2012; 2012:902578. PMID: 23259001.


15. Chen SW, Min L, Li WJ, Kong WX, Li JF, Zhang YJ. The effects of angelica essential oil in three murine tests of anxiety. Pharmacol Biochem Behav. 2004; 79:377–382. PMID: 15501315.


16. Matsumoto K, Kohno S, Ojima K, Tezuka Y, Kadota S, Watanabe H. Effects of methylenechloride-soluble fraction of Japanese angelica root extract, ligustilide and butylidenephthalide, on pentobarbital sleep in group-housed and socially isolated mice. Life Sci. 1998; 62:2073–2082. PMID: 9627086.


17. Dugovic C, Maccari S, Weibel L, Turek FW, Van Reeth O. High corticosterone levels in prenatally stressed rats predict persistent paradoxical sleep alterations. J Neurosci. 1999; 19:8656–8664. PMID: 10493766.


18. Edéll-Gustafsson UM, Kritz EI, Bogren IK. Self-reported sleep quality, strain and health in relation to perceived working conditions in females. Scand J Caring Sci. 2002; 16:179–187. PMID: 12000672.


19. Ford DE, Kamerow DB. Epidemiologic study of sleep disturbances and psychiatric disorders. An opportunity for prevention? JAMA. 1989; 262:1479–1484. PMID: 2769898.


20. Kang SY, Lee KY, Park MJ, Kim YC, Markelonis GJ, Oh TH, Kim YC. Decursin from Angelica gigas mitigates amnesia induced by scopolamine in mice. Neurobiol Learn Mem. 2003; 79:11–18. PMID: 12482674.


21. Morton SK, Whitehead JR, Brinkert RH, Caine DJ. Resistance training vs. static stretching: effects on flexibility and strength. J Strength Cond Res. 2011; 25:3391–3398. PMID: 21969080.


22. Wolfman C, Viola H, Marder M, Wasowski C, Ardenghi P, Izquierdo I, Paladini AC, Medina JH. Anxioselective properties of 6,3'-dinitroflavone, a high-affinity benzodiazepine receptor ligand. Eur J Pharmacol. 1996; 318:23–30. PMID: 9007508.


23. Darias V, Abdala S, Martin-Herrera D, Tello ML, Vega S. CNS effects of a series of 1,2,4-triazolyl heterocarboxylic derivatives. Pharmazie. 1998; 53:477–481. PMID: 9699225.
24. Paxinos G, Watson C, Pennisi M, Topple A. Bregma, lambda and the interaural midpoint in stereotaxic surgery with rats of different sex, strain and weight. J Neurosci Methods. 1985; 13:139–143. PMID: 3889509.


25. Sanford LD, Yang L, Liu X, Tang X. Effects of tetrodotoxin (TTX) inactivation of the central nucleus of the amygdala (CNA) on dark period sleep and activity. Brain Res. 2006; 1084:80–88. PMID: 16546144.


26. Tokunaga S, Takeda Y, Niimoto T, Nishida N, Kubo T, Ohno T, Matsuura Y, Kawahara Y, Shinomiya K, Kamei C. Effect of valerian extract preparation (BIM) on the sleep-wake cycle in rats. Biol Pharm Bull. 2007; 30:363–366. PMID: 17268081.


27. Houston CM, Smart TG. CaMK-II modulation of GABA(A) receptors expressed in HEK293, NG108-15 and rat cerebellar granule neurons. Eur J Neurosci. 2006; 24:2504–2514. PMID: 17100839.


28. Ma Y, Han H, Eun JS, Kim HC, Hong JT, Oh KW. Sanjoinine A isolated from Zizyphi Spinosi Semen augments pentobarbital-induced sleeping behaviors through the modification of GABA-ergic systems. Biol Pharm Bull. 2007; 30:1748–1753. PMID: 17827733.


29. West MR, Molloy CR. A microplate assay measuring chloride ion channel activity. Anal Biochem. 1996; 241:51–58. PMID: 8921165.


30. Wagner M, Weber P, Bruns T, Strauss WS, Wittig R, Schneckenburger H. Light dose is a limiting factor to maintain cell viability in fluorescence microscopy and single molecule detection. Int J Mol Sci. 2010; 11:956–966. PMID: 20479994.


31. Han S, Niu W, Li H, Hu L, Yuan Y, Xu G. Effect of hydroxyl and amino groups on electrochemiluminescence activity of tertiary amines at low tris(2,2'-bipyridyl)ruthenium(II) concentrations. Talanta. 2010; 81:44–47. PMID: 20188885.


32. Yan JJ, Kim DH, Moon YS, Jung JS, Ahn EM, Baek NI, Song DK. Protection against beta-amyloid peptide-induced memory impairment with long-term administration of extract of Angelica gigas or decursinol in mice. Prog Neuropsychopharmacol Biol Psychiatry. 2004; 28:25–30. PMID: 14687853.
33. Ahn KS, Sim WS, Kim IH. Decursin: a cytotoxic agent and protein kinase C activator from the root of Angelica gigas. Planta Med. 1996; 62:7–9. PMID: 8720379.
34. Huang SH, Lin CM, Chiang BH. Protective effects of Angelica sinensis extract on amyloid beta-peptide-induced neurotoxicity. Phytomedicine. 2008; 15:710–721. PMID: 18448320.
35. Lee S, Shin DS, Kim JS, Oh KB, Kang SS. Antibacterial coumarins from Angelica gigas roots. Arch Pharm Res. 2003; 26:449–452. PMID: 12877552.
36. Kim JH, Jung JH, Kim SH, Jeong SJ. Decursin exerts anti-cancer activity in MDA-MB-231 breast cancer cells via inhibition of the Pin1 activity and enhancement of the Pin1/p53 association. Phytother Res. 2014; 28:238–244. PMID: 23580332.


37. Seo YJ, Kwon MS, Park SH, Sim YB, Choi SM, Huh GH, Lee JK, Suh HW. The analgesic effect of decursinol. Arch Pharm Res. 2009; 32:937–943. PMID: 19557373.


38. Jang HO, Huh MH, Lee SW, Lee YH, Lee JH, Seo JB, Koo KI, Jin SD, Jeong JH, Lim JS, Bae MK, Yun I. The effect of dimyristoylphosphatidylethanol on the lateral and rotational mobilities of liposome lipid bilayers. Arch Pharm Res. 2005; 28:839–847. PMID: 16116676.


39. Feinberg I, Maloney T, Campbell IG. Effects of hypnotics on the sleep EEG of healthy young adults: new data and psychopharmacologic implications. J Psychiatr Res. 2000; 34:423–438. PMID: 11165310.


40. Tobler I, Kopp C, Deboer T, Rudolph U. Diazepam-induced changes in sleep: role of the alpha 1 GABA(A) receptor subtype. Proc Natl Acad Sci U S A. 2001; 98:6464–6469. PMID: 11353839.
41. Qiu MH, Qu WM, Xu XH, Yan MM, Urade Y, Huang ZL. D(1)/D(2) receptor-targeting L-stepholidine, an active ingredient of the Chinese herb Stephonia, induces non-rapid eye movement sleep in mice. Pharmacol Biochem Behav. 2009; 94:16–23. PMID: 19604496.


42. Bastien CH, LeBlanc M, Carrier J, Morin CM. Sleep EEG power spectra, insomnia, and chronic use of benzodiazepines. Sleep. 2003; 26:313–317. PMID: 12749551.


43. Liang SL, Carlson GC, Coulter DA. Dynamic regulation of synaptic GABA release by the glutamate-glutamine cycle in hippocampal area CA1. J Neurosci. 2006; 26:8537–8548. PMID: 16914680.


44. Tillakaratne NJ, Medina-Kauwe L, Gibson KM. gamma-Aminobutyric acid (GABA) metabolism in mammalian neural and nonneural tissues. Comp Biochem Physiol A Physiol. 1995; 112:247–263. PMID: 7584821.


45. Möhler H. GABA(A) receptor diversity and pharmacology. Cell Tissue Res. 2006; 326:505–516. PMID: 16937111.


46. Rudolph U, Crestani F, Benke D, Brünig I, Benson JA, Fritschy JM, Martin JR, Bluethmann H, Möhler H. Benzodiazepine actions mediated by specific gamma-aminobutyric acid(A) receptor subtypes. Nature. 1999; 401:796–800. PMID: 10548105.
47. McKernan RM, Rosahl TW, Reynolds DS, Sur C, Wafford KA, Atack JR, Farrar S, Myers J, Cook G, Ferris P, Garrett L, Bristow L, Marshall G, Macaulay A, Brown N, Howell O, Moore KW, Carling RW, Street LJ, Castro JL, Ragan CI, Dawson GR, Whiting PJ. Sedative but not anxiolytic properties of benzodiazepines are mediated by the GABA(A) receptor alpha1 subtype. Nat Neurosci. 2000; 3:587–592. PMID: 10816315.
48. Löw K, Crestani F, Keist R, Benke D, Brünig I, Benson JA, Fritschy JM, Rülicke T, Bluethmann H, Möhler H, Rudolph U. Molecular and neuronal substrate for the selective attenuation of anxiety. Science. 2000; 290:131–134. PMID: 11021797.


49. Crestani F, Löw K, Keist R, Mandelli M, Möhler H, Rudolph U. Molecular targets for the myorelaxant action of diazepam. Mol Pharmacol. 2001; 59:442–445. PMID: 11179437.


50. Crestani F, Keist R, Fritschy JM, Benke D, Vogt K, Prut L, Blüthmann H, Möhler H, Rudolph U. Trace fear conditioning involves hippocampal alpha5 GABA(A) receptors. Proc Natl Acad Sci U S A. 2002; 99:8980–8985. PMID: 12084936.
51. Collinson N, Kuenzi FM, Jarolimek W, Maubach KA, Cothliff R, Sur C, Smith A, Otu FM, Howell O, Atack JR, McKernan RM, Seabrook GR, Dawson GR, Whiting PJ, Rosahl TW. Enhanced learning and memory and altered GABAergic synaptic transmission in mice lacking the alpha 5 subunit of the GABAA receptor. J Neurosci. 2002; 22:5572–5580. PMID: 12097508.
52. Korpi ER, Gründer G, Lüddens H. Drug interactions at GABA(A) receptors. Prog Neurobiol. 2002; 67:113–159. PMID: 12126658.


Fig. 2
Decreased effects of decursinol angelate (DA) and diazepam (DZ) on locomotor activity test in mice.
Ambulation activity was measured for 1 h, 30 min after oral administration of diazepam and 1 h after the administration of DCA. Each column represents the mean with S.E.M (n=10). The statistical significance was assessed using analysis of variance (ANOVA) followed by Holm-sidak test. **p<0.01, ***p<0.001 compared with that of the control.
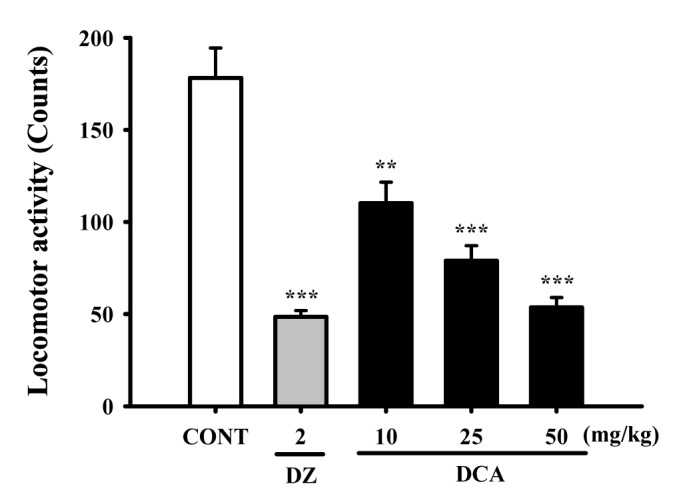
Fig. 3
Decreased effects of DCA on onset and duration of sleep in pentobarbital-treated mice.
Mice were food deprived for 24 h prior to the experiment. Pentobarbital (42 mg/kg, i.p.) was injected to mice following administration of muscimol (MUSC) or DCA. The sleep latency time (A) and total sleeping time (B) were recorded. Each column represents the mean with S.E.M (n=12~14). The significance of the effects of the compounds was assessed using analysis of variance (ANOVA). Where there was significant variability, the individual values were compared using Holm-sidak test. *p<0.05, **p<0.01, ***p<0.001, compared with that of the control.
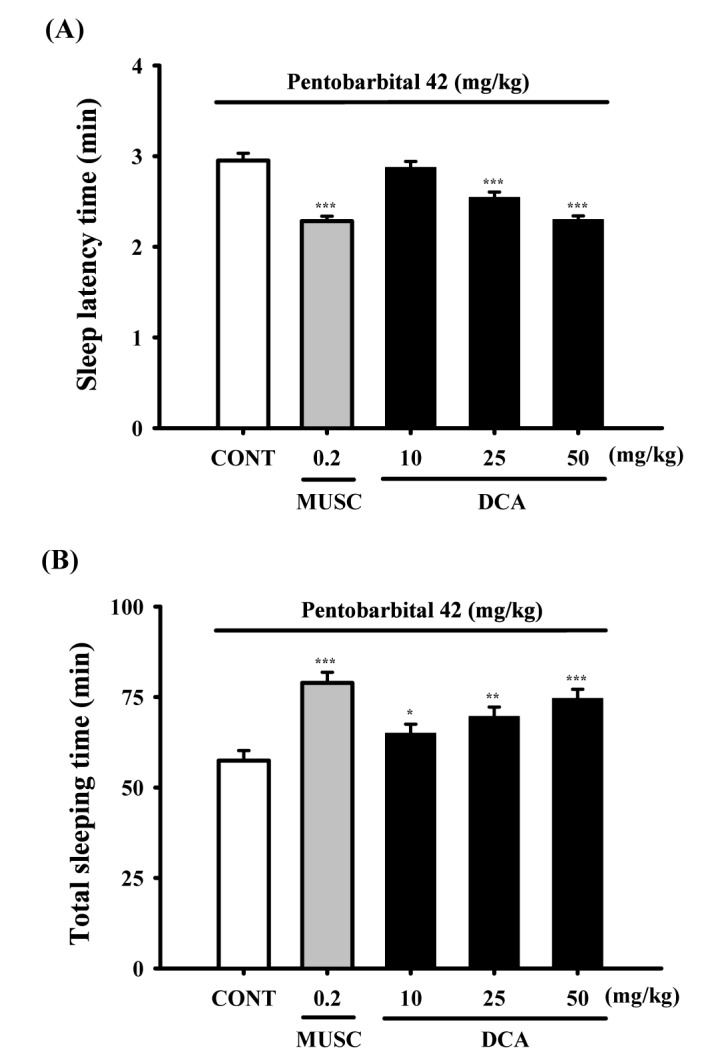
Fig. 4
Decreased effects of DCA (50 mg/kg) on the counts of sleep-wake cycles.
Where there was significant variability, the individual values were calculated as mean with S.E.M (n=8) were compared using Holm-sidak test. *p<0.05, compared with that of the naïve control.
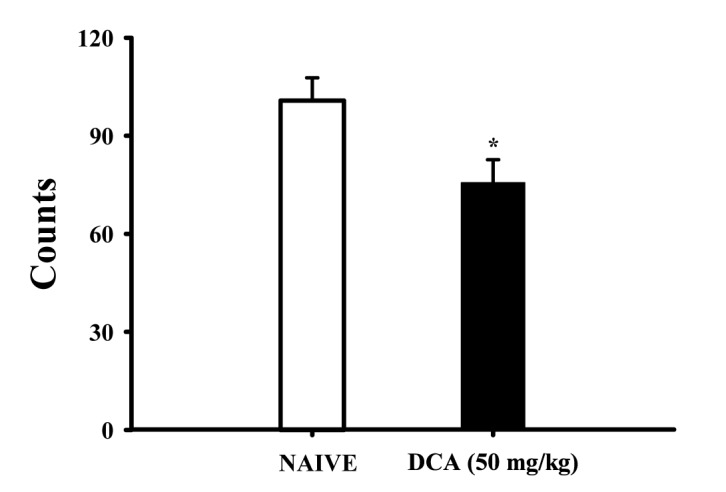
Fig. 5
Effects of DCA on sleep architectures in rats.
The data represent the mean with S.E.M (n=8) of time spent, which separated the wakefulness/sleep (NREM and REM sleep) state. The significance of the effects of the compounds was assessed using analysis of variance (ANOVA). Where there was significant variability, the individual values were compared using Holm-sidak test. *p<0.05, Compared with that of the naïve control.
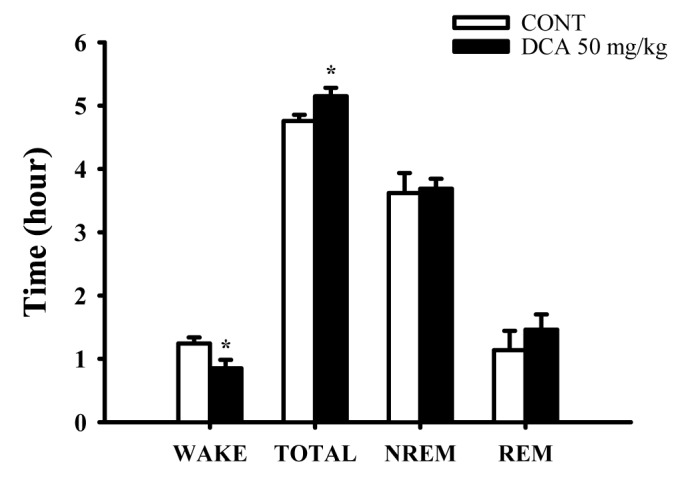
Fig. 6
Effects of DCA on EEG power density of wakefulness (A), REM sleep (B) and NREM sleep (C).
The power density was departmentalized into δ-wave, θ-wave, and α-wave. Each wave represents the mean with S.E.M (n=8). The significance of the effects of the compounds was assessed using analysis of variance (ANOVA). Where there was significant variability, the individual values were compared using Holm-sidak test. *p<0.05, **p<0.01, ***p<0.001, compared with that of the naïve control.
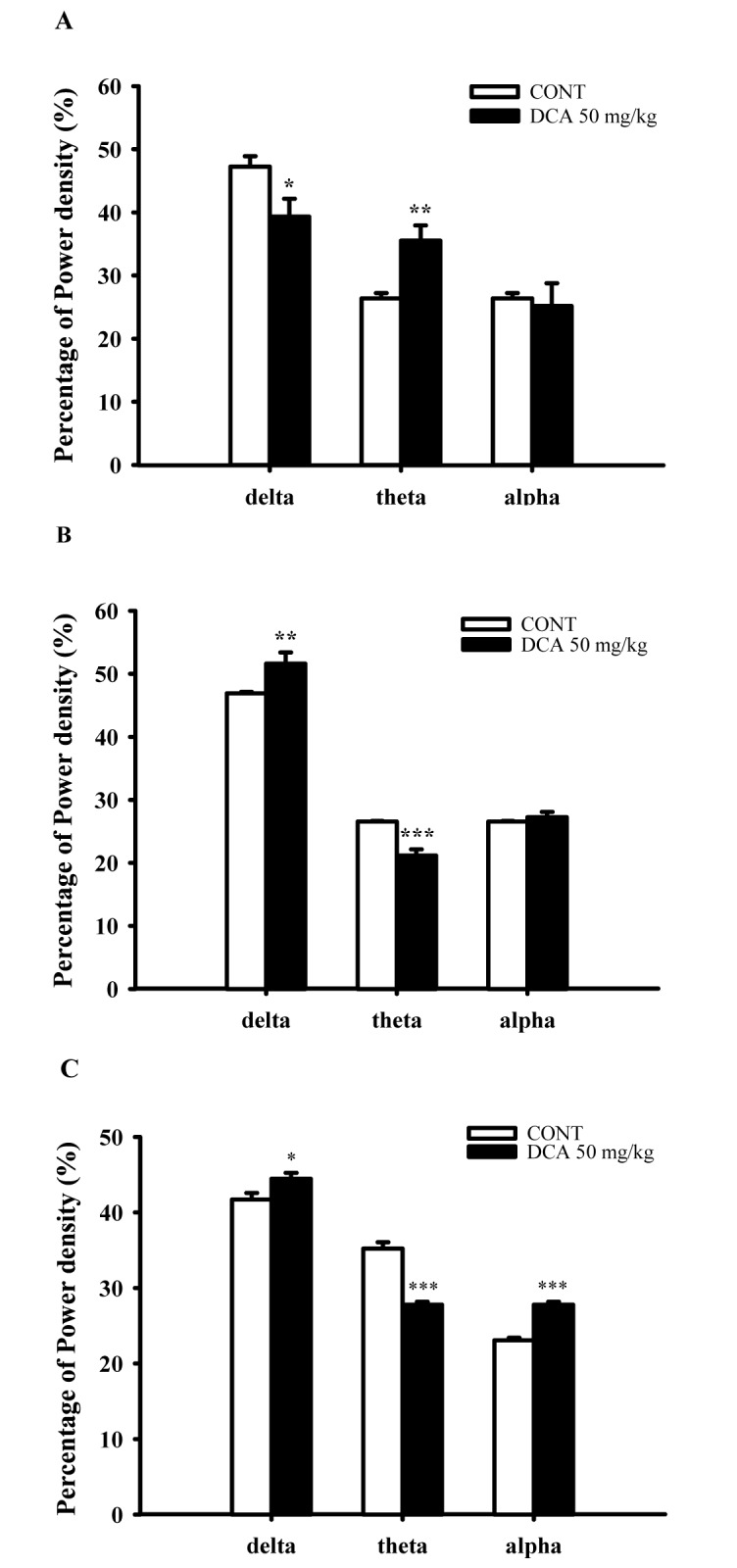
Fig. 7
Decreased effects of DCA on Cl– influx in primary cultured hypothalamic neuronal cells.
The cells were incubated with MQAE overnight, after the culture of hypothalamic neuronal cells for 8 days, and then DCA (0.001, 0.01 and 0.1 µg/ml) and pentobarbital (PENT 10 µm) were added 1 h prior to measurement. Each column represents the mean with S.E.M (n=3). The significance of the effects of the compounds was assessed using analysis of variance (ANOVA). Where there was significant variability, the individual values were compared using Holm-sidak test. **p<0.01, ***p<0.005, compared with that of the naïve control.
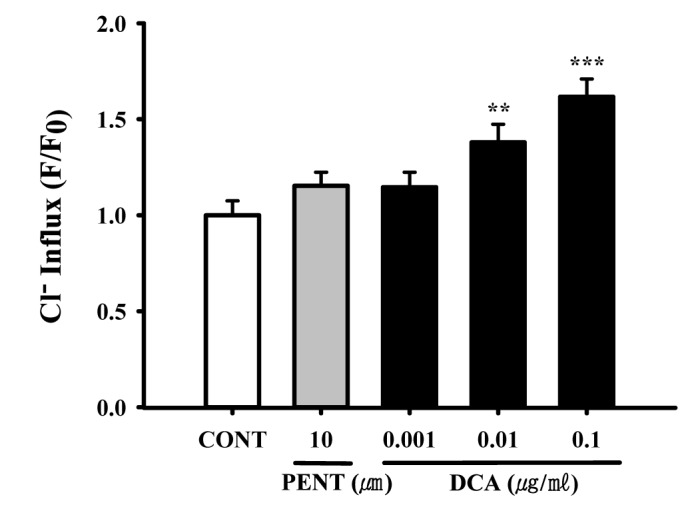
Fig. 8
Increased effects of DCA on the expression of GAD.
Immunoblots of lysed hypothalamus tissues which were treated for 1 h following DCA are shown. GAPDH levels were need for the normalization of the protein expression. Each column represents the mean with S.E.M (n=3). The significance of the effects of the compounds was assessed using analysis of variance (ANOVA). Where there was significant variability, the individual values were compared using Holm-sidak t-test. **p<0.01, compared with that of the control. CONT means the control group and GAD means glutamic acid decarboxylase.
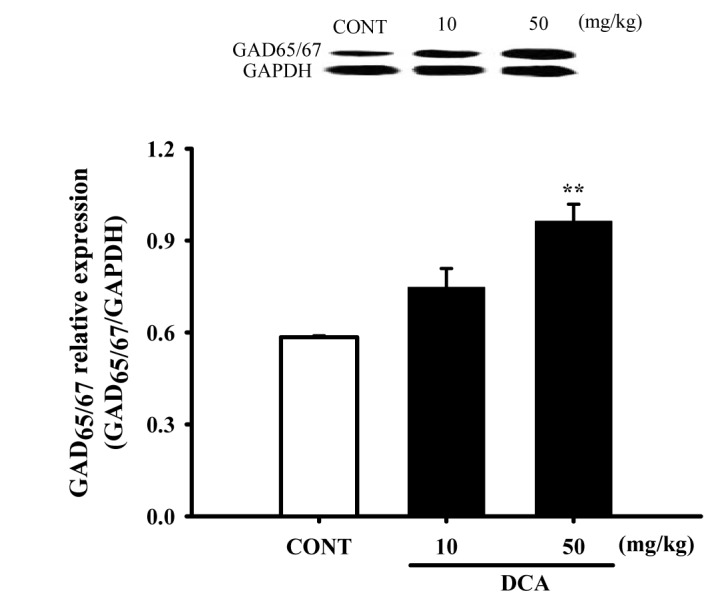
Fig. 9
Increased effects of DCA on expression of GABAA receptors subunits.
Immunobblots of lysed hypothalamus tissue which were treated for 1 h following DCA are shown. GAPDH levels were need for the normalization of the protein expression. Each column represents the mean with S.E.M (n=3). The significance of the effects of the compounds was assessed using analysis of variance (ANOVA). Where there was significant variability, the individual values were compared using Holm-sidak test. *p<0.05, **p<0.01, ***p<0.005 compared with that control. CONT means the control group, and DZ means the diazepam group.
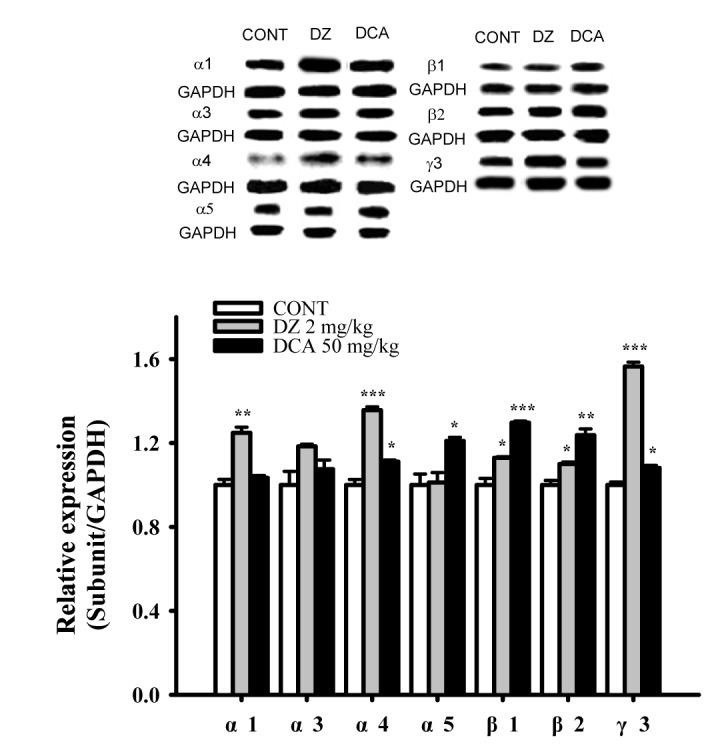