Abstract
Excessive influx and the subsequent rapid cytosolic elevation of Ca2+ in neurons is the major cause to induce hyperexcitability and irreversible cell damage although it is an essential ion for cellular signalings. Therefore, most neurons exhibit several cellular mechanisms to homeostatically regulate cytosolic Ca2+ level in normal as well as pathological conditions. Delayed rectifier K+ channels (IDR channels) play a role to suppress membrane excitability by inducing K+ outflow in various conditions, indicating their potential role in preventing pathogenic conditions and cell damage under Ca2+-mediated excitotoxic conditions. In the present study, we electrophysiologically evaluated the response of IDR channels to hyperexcitable conditions induced by high Ca2+ pretreatment (3.6 mM, for 24 hours) in cultured hippocampal neurons. In results, high Ca2+-treatment significantly increased the amplitude of IDR without changes of gating kinetics. Nimodipine but not APV blocked Ca2+-induced IDR enhancement, confirming that the change of IDR might be targeted by Ca2+ influx through voltage-dependent Ca2+ channels (VDCCs) rather than NMDA receptors (NMDARs). The VDCC-mediated IDR enhancement was not affected by either Ca2+-induced Ca2+ release (CICR) or small conductance Ca2+-activated K+ channels (SK channels). Furthermore, PP2 but not H89 completely abolished IDR enhancement under high Ca2+ condition, indicating that the activation of Src family tyrosine kinases (SFKs) is required for Ca2+-mediated IDR enhancement. Thus, SFKs may be sensitive to excessive Ca2+ influx through VDCCs and enhance IDR to activate a neuroprotective mechanism against Ca2+-mediated hyperexcitability in neurons.
Dynamic changes of cytosolic Ca2+ level under physiological conditions are essential for cellular signalings of protein synthesis and signal transmission of neurons in central nervous system (CNS). However, due to its cytotoxic effects, excessive influx of Ca2+ and subsequent rapid elevation of [Ca2+]i can induce irreversible damage of neurons or result in pathological dysfunctions such as epileptic seizure. Hence, mammalian neurons exhibit several protective mechanisms to homeostatically regulate membrane excitability and cytosolic Ca2+ level. Several types of voltage dependent ion channels are involved in these neuroprotective processes via regulating cation flow. In its original description based on neuronal excitability, intrinsic excitability can be defined as the ability to fire action potentials (APs), which are determined by voltage-dependent K+ and Na+ channels (KV and NaV channels) [1]. Therefore, cation channels especially sensitive to membrane potential are key factors to regulate excitability in most neurons even though they are inhibitory. In particular, several subtypes of Kv channels can determine resting membrane potential and effectively induce cation outflow to downregulate neuronal excitability in many conditions of membrane depolarization [2].
Among the many types of K+ channels expressed in CNS neurons, Kv2.1 is a major component of delayed rectifier K+ channel (IDR channel), exhibiting sustained outward K+ currents [345]. This subtype plays a direct role in lowering membrane potential thus inhibiting AP initiation as well as limiting repetitive APs firing. Thus, the hyperpolarization of membrane potential and blockade of depolarization by Kv2.1 can prevent hyperexcitability that activates cytotoxic cascades and induces neuronal damage [67]. Previous studies showed that Kv2.1 channels are sensitive to changes in cytosolic Ca2+ level. For example, cytosolic Ca2+ increase and subsequent calcineurin activation affects the gating kinetics of IDR channels via dephosphorylating Kv2.1 channels, since calcineurin-dependent dephosphorylation of Kv2.1 decreases the threshold for IDR channel opening and disrupts channel clustering, resulting in changes of activation kinetics [18]. Kv2.1 channels have many serine, threonine, and tyrosine phosphorylation sites. These participate in the regulation of IDR channel kinetics; for e.g., PKA-mediated phosphorylation in serine/threonine sites induces the changes of gating kinetics but not membrane expression of Kv2.1 channels [910]. Dynamic changes of gating kinetics by de- or phosphorylation of Kv2.1 channels seem to be minor but significant for regulating K+ outflow and membrane excitability under physiological conditions. However, other studies have recently demonstrated that under several pathological conditions such as epileptic seizures and ischemic damages, acute increment of cytosolic Ca2+ rapidly potentiates IDR, and thus suppresses hyperexcitability [111213]. These results suggest that rapid changes of cytosolic Ca2+ may stimulate more effective signaling pathways by which IDR channels directly regulate membrane excitability. However, whether excessive Ca2+ influx rapidly and potentially enhances IDR remains unclear.
In the present study, we confirmed that dissociated hippocampal neurons of rats showed significant increase of IDR after high Ca2+ treatment, which could enhance synaptic activities and membrane excitability. The Ca2+-mediated IDR upregulation was sensitive to activity of voltage-dependent Ca2+ channels (VDCCs) but not NMDA receptors (NMDARs), suggesting a neuroprotective signaling pathway that is not targeted by postsynaptic Ca2+ influx via NMDARs. In the additional experiment, we confirmed that the contribution of Src family tyrosine kinase (SFK) was necessary for upregulating IDR under high Ca2+ condition, suggesting that the increased expression of IDR channels via SFK activation is a protective mechanism against excitotoxicity and pathogenic processes in neurons.
Hippocampal primary cultures were prepared from embryonic 20-day SD rats. After pregnant rats were deeply anesthetized, the embryos were removed and transferred to an ice-cold normal tyrode solution containing the following (in mM): 140 NaCl, 5.4 KCl, 2.3 MgCl2, 10 HEPES, 5 glucose. The pH level was adjusted to 7.4 with NaOH. Isolated hippocampi from embryonic rat brains were transferred to ice-cold minimal essential medium (MEM) containing Earle's salts and glutamine with 10% fetal bovine serum, 0.45% glucose, 1 mM sodium pyruvate, 25 µM glutamate and antibiotics, and the hippocampi were triturated. The cells were counted and seeded on glass coverslips coated with poly-L-lysine (Sigma-Aldrich) at a density of 9×104 cells/ml and transferred to an incubator gassed with 95% O2 and 5% CO2 at 37℃. After 7 hours, the whole plating medium was exchanged to Neurobasal medium (Sigma-Aldrich) containing B-27 (Invitrogen), and a half of the medium was replaced with new media once at DIV 5. Detail protocols for cell preparation and recording techniques were as previously reported [1415]. All experiments and procedures with animals were approved by the Animal Care and Use Committee of Jeju National University.
To electrophysiologically observe the changes of IDR in cultured hippocampal neurons, high Ca2+ condition was induced by doubling Ca2+ concentration of culture media (normal 1.8 mM) up to 3.6 mM (with CaCl2, Sigma-Aldrich) for 24 hours. APV (100 mM, Sigma-Aldrich) and nimodipine (10 mM, Sigma-Aldrich) were used with high Ca2+ treatment to block NMDARs and VDCCs, respectively, in some experiments. H89 was added to block the activation of PKA and ryanodine receptors and IP3 receptors of ER were blocked by Ryanodine and 2AP, respectively. Blockers of kinases and receptors were obtained from Sigma Aldrich. Caffeine or 4CMC (Sigma-Aldrich) were used to test the effects of CICR via ryanodine receptor activation. Details of drugs treatment are mentioned in results and legends of figures.
For patch-clamp recordings of dissociated hippocampal neurons, DIV 6~8 cultured cells were transferred to a recording chamber with a continuous flow of recording solution containing the following (in mM): 145 NaCl, 5 KCl, 2 CaCl2, 1.3 MgCl2, 10 HEPES, 10 glucose. pH level was adjusted to 7.4 with NaOH, and bubbled with 95% O2 and 5% CO2. TTX (0.5 µM, Tocrise) was added to the recording solution to block the Nav channels. The patch pipettes (4~6 MΩ) were filled with an internal solution containing the following (in mM): 20 KCl, 125 K+-gluconate, 4 NaCl, 10 HEPES, 0.5 EGTA, 4 ATP, 0.3 tris-GTP, and 10 phosphocreatin. pH and osmolarity were adjusted to 7.2 and 280~300 mOsm, respectively. In all experiments, neurons showing 7~11 pF whole cell capacitance were used. Whole cell recording parameters were monitored throughout each experiment and recordings where series resistance (6~20 MΩ) varied by more than 10% were rejected.
All recordings were performed at room temperature, low-pass filtered at 5 kHz, and digitized at 10 kHz by a Digidata 1322A convertor. Voltage-clamping whole cell patches, command pulse generation and data recording were performed using Axopatch 200B and pClamp 8 software. Thick-walled, filamented patch electrodes had tip resistance of 3~6 MOhm. For adjusting whole-cell parameters, membrane capacitance and series resistance were manually compensated in Axopatch 200B during applying command pulses. Series resistance varied between 8~30 MOhm, and recordings where series resistance varied by more than 10% were rejected. No electronic compensation for series resistance was employed during currents recording. Pulse commands to record sustained currents in voltage-clamping mode are described in detail in results and figures. Sustained K+ currents were digitally isolated using a prepulse protocol after subtracting leak currents. Peak currents were measured at +60 mV. All experimental data were additionally analyzed using IGOR pro software.
In physiological regulation of neuronal excitability, the dominant role of small but sustained K+ outflow through IDR channels is to determine and stabilize sub- and suprathreshold membrane potentials. However, signaling-mediated changes of its kinetics suggest a possibility that IDR channels may also be targeted by hyperexcitable conditions. In the present study, we tested K+ outflow through IDR channels in response to hyperexcitable condition induced by high Ca2+ overnight treatment (3.6 mM CaCl2, for 24 hours) in cultured hippocampal neurons. This experimental condition not only induces the downregulation of A-type K+ channels (IA channels) but also enhances synaptic Ca2+ influx by remodeling NMDARs composition, resulting in the hyperexcitability of hippocampal neurons [1416]. After treating high Ca2+ to culture media for 24 hours, neurons were transferred to a recording chamber and washed in normal ACSF solution for electrophysiological measurement. In results, the current density of IDR channels was significantly increased by high Ca2+ treatment, as shown in Fig. 1 (Control=112.62±10.28 pA/pF, n=13; high Ca2+=171.05±18.30 pA/pF, n=12, p=0.02). This indicates that IDR channels may be upregulated under hyperexcitable conditions.
Depolarization of membrane potential activates two major Ca2+ influx pathways: VDCC and NMDAR. High Ca2+-enhanced neuronal excitability is reportedly dependent on Ca2+ influx via NMDARs, hence it was necessary to confirm if the increase of IDR was dependent on NMDARs [16]. APV (100 µM) or nimodipine (10 µM) with high Ca2+ treatment was applied to culture media for 24 hours before recording. APV-induced NMDARs block showed no effects on the high Ca2+-enhanced IDR in this experiment; whereas, nimodipine, a VDCCs antagonist, significantly inhibited the effect of high Ca2+ treatment on IDR (Fig. 1, APV=151.55±12.56 pA/pF, Nimodipine=96.03±9.34 pA/pF, p=0.48, 0.008 respectively, compared with high Ca2+). Thus, IDR upregulation may be triggered by Ca2+ influx through VDCCs rather than NMDARs. Furthermore, because it is well known that Ca2+-induced Ca2+ release (CICR) is responsive to Ca2+ influx, we also tested if it might participate in IDR upregulation resulted from Ca2+ influx via VDCCs. In Table 1, blocking of two major receptors of endoplasmic reticulum, ryanodine receptors (Ryanodine) and IP3 receptors (2APB), showed no effects on enhanced IDR under high Ca2+ condition. Additionally, caffeine or 4CMC, agonists of ryanodine receptors, showed no enhancement effects on IDR, unlike high Ca2+ treatment. These results strongly indicated that Ca2+ influx through VDCCs may directly regulate IDR channels regardless of CICR activation.
Although the current density of IDR was increased, the gating kinetics of IDR channels was not affected under high Ca2+ condition. In Fig. 2, the increase rate of IDR positively reflected the increase rates of command pulse potentials in all experimental groups. At +80 mV injection, significant differences of IDR amplitudes were observed between control and high Ca2+ treated neurons (Fig. 2A and B, Control=139.82±15.62 pA/pF; High Ca2+=226.79±17.34 pA/pF, p=0.02). The current density of IDR under high Ca2+ condition was enhanced up to 60% in each injection range from −40 to 80 mV compared with the control group. However, in the analysis of conductance compensated by each membrane potential, activation properties of IDR channels were consistent in all groups, indicating that the gating kinetics of IDR channels may not be subjected to high Ca2+ condition (Fig. 2C and D, Vh of activation: Control=12.38±2.62 mV; High Ca2+=22.21±4.4 mV, p=0.1). This result suggests that the upregulation of IDR channels under high Ca2+ condition may not be dependent on their dephosphorylation by calcineurin activated by Ca2+ signaling. Additionally, both antagonists, APV and nimodipine, showed no effects on the activation kinetics of IDR channels (Vh of activation; APV group=10.11±1.91; Nimodipine group=14.92±1.63, p=0.55 and 0.45, respectively).
Previously, Ledoux et al. [17] reported that the enhancement of intracellular Ca2+ concentration induced the elevation of K+ efflux through small conductance Ca2+ channels (SK channels). Because of possible change in intracellular Ca2+ concentration under the experimental condition, it was necessary to confirm whether the enhanced density of IDR by high Ca2+ was contaminated by the flow of SK channels even though the two channels exhibit totally different gating mechanisms. In Fig. 3, the effect of 100 nM apamin with high Ca2+ was tested by adding to culture media for 24 hours to block SK channels. The addition of apamin did not influence the increased density of IDR by high Ca2+ treatment, indicating that upregulating IDR is not via the activation of SK channels (179.37±26.56 pA/pF, p=0.04 and 0.81, as compared with control and high Ca2+, respectively).
Ca2+ signaling cascades activated by Ca2+ influx through synaptic NMDARs induce the phosphorylation of protein kinase A (PKA) resulting in the downregulation of IA in hippocampal neurons [1518]. Although it is still under argument, it is possible that PKA regulates the turnover rate of proteins from plasma membrane and currents through various channels. In this study, PKA was not involved in the cellular processing to enhance IDR under high Ca2+ condition (Table 1, +H89). Rather, we confirmed that SFKs have a potentially important role in IDR upregulation under high Ca2+ condition (Fig. 3). Adding SFK blocker, PP2 (1 µM), to culture media with high Ca2+ showed no enhancement of IDR, with similar levels as in control neurons that were not treated with any drugs (Ca2++PP2=99.01±10.52 pA/pF, p=0.43 and 0.02 compared with the control and high Ca2+, respectively). Furthermore, PP2 alone showed no effect on the density of IDR, indicating that high Ca2+ condition possibly activate SFKs to prevent neuronal excitotoxicity or damage (123.46±20.23 pA/pF, p=0.63 compared with the control).
Neurons in CNS exhibit dynamic regulatory signalings to protect from hyperexcitable conditions that result in neuronal damage. Herein, we reported upregulation of IDR channels, as a neuroprotective regulator, which increased K+ outflow under hyperexcitable condition induced by high Ca2+ overnight treatment in cultured hippocampal neurons. The high Ca2+-induced enhancement of IDR was dependent on Ca2+ influx through VDCCs but not NMDARs and was not affected by CICRs that synergistically increase intracellular Ca2+ level (Fig. 1 and Table 1). These results indicate that hippocampal neurons may homeostatically modulate IDR channels in response to Ca2+ influx through VDCCs. Thus, neuronal excitability is consequently downregulated by the enhancement of K+ outflow via IDR channels. Furthermore, IDR enhancement was dependent on tyrosine kinases of Src family, indicating the possibility of an unique signaling cascade for the downregulation of excitability to prevent neuronal damages under hyperexcitable or pathogenic conditions.
High Ca2+ treatment for 24 hours used in this study (i.e. doubling Ca2+ concentration in culture media), is reported to mediate changes in the subunit composition of synaptic NMDARs, IA density and Ca2+-calmodulin dependent kinase activity [141619]. Increased Ca2+ influx through NMDARs and subsequent IA downregulation critically increases neuronal excitability due to changes in membrane resistance, firing rates of APs and after-hyperpolarizing potentials resulting from direct effects of the cationic flow through channels or receptors [1619]. These effects of high Ca2+ condition possibly result in irreversible damage to hippocampal neurons. However, hippocampal neurons showing Ca2+-induced downregulation of Kv4.2 channels had no effect on the resting membrane potential even though suprathreshold properties of membrane excitability were significantly changed [19]. This phenomenon is consistent with previous results in hippocampal neurons pretreated with high Ca2+, indicating that membrane potentials may also be targeted by a regulatory mechanism to prevent excitotoxicity [14]. In this study, the enhancement of IDR strongly suggested a neuroprotective mechanism related with IDR channels and Ca2+ influx through VDCCs (Fig. 1). IDR channels contribute to the restoration of depolarized membrane potential to resting membrane potential in cardiac cells, determining refractory period as well as heart rate. In nervous system, these channels seem to contribute to the regulation of somatodendritic excitability. Kv2 family mediated with IDR acts as a dominant regulatory factor for membrane excitability in hippocampal and cortical pyramidal neurons [202122]. Experimental evidence indicates that the genetic manipulation of Kv2.1 directly affects intrinsic excitability, showing epileptiform activity, and that Kv2.1 knock-out mice exhibit defects in spatial learning [23]. These pathogenic outcomes are likely due to the hyperexcitability of neurons during development, as IDR channels are targeted by cellular signaling pathways of various growth factors in young brain [24]. Therefore, enhanced IDR under high Ca2+ condition observed in this study may be a rapid but effective neuroprotective modulation to prevent entry into pathogenic stages.
As a critical regulator of neuronal excitability, IDR channels are dominantly targeted by Ca2+-mediated signaling in the manner of activity- and use-dependence. Even though IDR channels are highly phosphorylated in neurons, the expression and gating kinetics of these channels are bidirectionally subjected to activity-dependent dephosphorylation, which is mediated by glutamatergic transmission [111325]. The dephosphorylation of these channels can rapidly and homeostatically suppress neuronal excitability under pathological conditions via the enhancement of K+ outflow. Dephosphorylation of IDR channels under anoxic stress is dependent on Ca2+ influx through NMDARs that are activated by excess glutamate release in synapses [25]. Thus, overexcitability of glutamatergic synapses in various pathological conditions possibly activates neuroprotective signalings to dephosphorylate and upregulate IDR channels. However, we observed that enhanced IDR under high Ca2+ condition was clearly dependent on Ca2+ influx through VDCCs but not NMDARs. This is evidenced by the effect of nimodipine to abolish the enhancement of IDR (Fig. 1). Despite previous reports on the effects of VDCC blockers to inhibit IDR, nimodipine, one of the dihydropyridines, showed no direct inhibition of IDR in this study (data not shown) [262728]. Because IA downregulation is dependent on NMDARs under high Ca2+ condition, IDR enhancement may be a possible mechanism of compensatory regulation in hippocampal neurons that are chronically exposed to high Ca2+ [14]. IDR channels are predominantly regulated by calcineurin-dependent dephosphorylation, which is accompanied by changes of threshold as well as activation kinetics [18]. In this study, however, we confirmed that high Ca2+-induced upregulation of IDR involved only the increase of IDR peak but not the alteration of gating kinetics, suggesting the possibility of another signaling mechanism distinctive to calcineurin-dependent dephosphorylation. Moreover, the bidirectional and homeostatic upregulation of IDR observed in neurons is due to the overexcitation of glutamatergic synapses, hence, the enhancement of IDR peak without a kinetic change may be originated from Ca2+ influx via VDCCs rather than NMDARs [111325].
The upregulation of IDR peak without changes of gating kinetics suggests the involvement of specific modulatory signalings of kinases, which can be activated under high Ca2+ condition (Fig. 1 and 2). Previous reports focusing on regulatory mechanisms of KV2.1 channel indicate that these channels possess a large number of kinase binding sites such as serine, threonine, and tyrosine, and are usually affected by all except threonine [910]. In particular, tyrosine kinases, such as Src and Fyn, seem to play an important role in the regulation of KV2.1 channel expression. Although, tyrosine 124 phosphorylated by src regulates channels activities, tyrosine 810 phosphorylation influences the intracellular trafficking, regulating Kv2.1 channels expression [293031]. Furthermore, growth differentiation factor 15 (GDF15)-induced upregulation of Kv2.1 channels expression was also abolished by the inhibition of Src-mediated phosphorylation of TGFβ receptors [24]. These previous reports indicate that SFKs are dominantly mediated with the cellular regulation of Kv2.1 channel expression, while the gating kinetics of IDR channels is specifically targeted by serine kinases. Thus, the upregulation of IDR by high Ca2+ treatment observed in this study may be originated from the modulatory function of tyrosine kinases. Fig. 2 clearly shows that PP2 blocked the enhancement of IDR under high Ca2+ condition, indicative of SFKs' roles in IDR channels for preventing hyperexcitability.
Because excessive Ca2+ influx is sufficient to cause hyper-excitability and irreversible damage to hippocampal neurons, resulting in pathological conditions, a number of subcellular signalings in neurons target the expression and gating kinetics of Kv channels which actively regulate membrane excitability. The current study confirmed that a neuroprotective regulatory mechanism involving IDR channels in hippocampal neurons possibly plays a role to suppress neuronal excitability under various pathogenic conditions. Although further study is needed to clarify the relationship(s) between Ca2+ influx via VDCCs and SFK signaling in IDR upregulation, VDCC-mediated upregulation of IDR suggests another signaling pathway to actively regulate membrane excitability of hippocampal neurons.
ACKNOWLEDGEMENTS
This work was supported by the Academic Research Foundation of Jeju National University Institute of medical science in 2014.
Notes
References
1. Misonou H, Mohapatra DP, Trimmer JS. Kv2.1: a voltage-gated K+ channel critical to dynamic control of neuronal excitability. Neurotoxicology. 2005; 26:743–752. PMID: 15950285.
2. Oliver D, Lien CC, Soom M, Baukrowitz T, Jonas P, Fakler B. Functional conversion between A-type and delayed rectifier K+ channels by membrane lipids. Science. 2004; 304:265–270. PMID: 15031437.
3. Du J, Haak LL, Phillips-Tansey E, Russell JT, McBain CJ. Frequency-dependent regulation of rat hippocampal somato-dendritic excitability by the K+ channel subunit Kv2.1. J Physiol. 2000; 522:19–31. PMID: 10618149.
4. Malin SA, Nerbonne JM. Delayed rectifier K+ currents, IK, are encoded by Kv2 alpha-subunits and regulate tonic firing in mammalian sympathetic neurons. J Neurosci. 2002; 22:10094–10105. PMID: 12451110.
5. Pal S, Hartnett KA, Nerbonne JM, Levitan ES, Aizenman E. Mediation of neuronal apoptosis by Kv2.1-encoded potassium channels. J Neurosci. 2003; 23:4798–4802. PMID: 12832499.


6. Lien CC, Jonas P. Kv3 potassium conductance is necessary and kinetically optimized for high-frequency action potential generation in hippocampal interneurons. J Neurosci. 2003; 23:2058–2068. PMID: 12657664.


7. Surmeier DJ, Foehring R. A mechanism for homeostatic plasticity. Nat Neurosci. 2004; 7:691–692. PMID: 15220926.


8. Mohapatra DP, Trimmer JS. The Kv2.1 C terminus can autonomously transfer Kv2.1-like phosphorylation-dependent localization, voltage-dependent gating, and muscarinic modulation to diverse Kv channels. J Neurosci. 2006; 26:685–695. PMID: 16407566.


9. Jonas EA, Kaczmarek LK. Regulation of potassium channels by protein kinases. Curr Opin Neurobiol. 1996; 6:318–323. PMID: 8794088.


10. Murakoshi H, Shi G, Scannevin RH, Trimmer JS. Phosphorylation of the Kv2.1 K+ channel alters voltage-dependent activation. Mol Pharmacol. 1997; 52:821–828. PMID: 9351973.
11. Misonou H, Mohapatra DP, Menegola M, Trimmer JS. Calcium- and metabolic state-dependent modulation of the voltage-dependent Kv2.1 channel regulates neuronal excitability in response to ischemia. J Neurosci. 2005; 25:11184–11193. PMID: 16319318.


12. Misonou H, Mohapatra DP, Park EW, Leung V, Zhen D, Misonou K, Anderson AE, Trimmer JS. Regulation of ion channel localization and phosphorylation by neuronal activity. Nat Neurosci. 2004; 7:711–718. PMID: 15195093.


13. Park KS, Mohapatra DP, Misonou H, Trimmer JS. Graded regulation of the Kv2.1 potassium channel by variable phosphorylation. Science. 2006; 313:976–979. PMID: 16917065.


14. Kang MS, Yang YS, Kim SH, Park JM, Eun SY, Jung SC. The down-regulation of somatic a-type K+ channels requires the activation of synaptic nmda receptors in young hippocampal neurons of rats. Korean J Physiol Pharmacol. 2014; 18:135–141. PMID: 24757375.
15. Kim J, Jung SC, Clemens AM, Petralia RS, Hoffman DA. Regulation of dendritic excitability by activity-dependent trafficking of the A-type K+ channel subunit Kv4.2 in hippocampal neurons. Neuron. 2007; 54:933–947. PMID: 17582333.
16. Jung SC, Kim J, Hoffman DA. Rapid, bidirectional remodeling of synaptic NMDA receptor subunit composition by A-type K+ channel activity in hippocampal CA1 pyramidal neurons. Neuron. 2008; 60:657–671. PMID: 19038222.
17. Ledoux J, Werner ME, Brayden JE, Nelson MT. Calcium-activated potassium channels and the regulation of vascular tone. Physiology (Bethesda). 2006; 21:69–78. PMID: 16443824.


18. Hammond RS, Lin L, Sidorov MS, Wikenheiser AM, Hoffman DA. Protein kinase a mediates activity-dependent Kv4.2 channel trafficking. J Neurosci. 2008; 28:7513–7519. PMID: 18650329.


19. Kim J, Wei DS, Hoffman DA. Kv4 potassium channel subunits control action potential repolarization and frequency-dependent broadening in rat hippocampal CA1 pyramidal neurones. J Physiol. 2005; 569:41–57. PMID: 16141270.


20. Bekkers JM. Distribution and activation of voltage-gated potassium channels in cell-attached and outside-out patches from large layer 5 cortical pyramidal neurons of the rat. J Physiol. 2000; 525:611–620. PMID: 10856116.


21. Guan D, Tkatch T, Surmeier DJ, Armstrong WE, Foehring RC. Kv2 subunits underlie slowly inactivating potassium current in rat neocortical pyramidal neurons. J Physiol. 2007; 581:941–960. PMID: 17379638.


22. Mohapatra DP, Misonou H, Pan SJ, Held JE, Surmeier DJ, Trimmer JS. Regulation of intrinsic excitability in hippocampal neurons by activity-dependent modulation of the KV2.1 potassium channel. Channels (Austin). 2009; 3:46–56. PMID: 19276663.


23. Speca DJ, Ogata G, Mandikian D, Bishop HI, Wiler SW, Eum K, Wenzel HJ, Doisy ET, Matt L, Campi KL, Golub MS, Nerbonne JM, Hell JW, Trainor BC, Sack JT, Schwartzkroin PA, Trimmer JS. Deletion of the Kv2.1 delayed rectifier potassium channel leads to neuronal and behavioral hyperexcitability. Genes Brain Behav. 2014; 13:394–408. PMID: 24494598.


24. Wang CY, Huang AQ, Zhou MH, Mei YA. GDF15 regulates Kv2.1-mediated outward K+ current through the Akt/mTOR signalling pathway in rat cerebellar granule cells. Biochem J. 2014; 460:35–47. PMID: 24597762.
25. Ito T, Nuriya M, Yasui M. Regulation of Kv2.1 phosphorylation in an animal model of anoxia. Neurobiol Dis. 2010; 38:85–91. PMID: 20079839.


26. Choe H, Lee YK, Lee YT, Choe H, Ko SH, Joo CU, Kim MH, Kim GS, Eun JS, Kim JH, Chae SW, Kwak YG. Papaverine blocks hKv1.5 channel current and human atrial ultrarapid delayed rectifier K+ currents. J Pharmacol Exp Ther. 2003; 304:706–712. PMID: 12538825.
27. Perchenet L, Clément-Chomienne O. Characterization of mibefradil block of the human heart delayed rectifier hKv1.5. J Pharmacol Exp Ther. 2000; 295:771–778. PMID: 11046117.
28. Rampe D, Wible B, Fedida D, Dage RC, Brown AM. Verapamil blocks a rapidly activating delayed rectifier K+ channel cloned from human heart. Mol Pharmacol. 1993; 44:642–648. PMID: 8371716.
29. Tiran Z, Peretz A, Attali B, Elson A. Phosphorylation-dependent regulation of Kv2.1 Channel activity at tyrosine 124 by Src and by protein-tyrosine phosphatase epsilon. J Biol Chem. 2003; 278:17509–17514. PMID: 12615930.
30. Sobko A, Peretz A, Attali B. Constitutive activation of delayed-rectifier potassium channels by a src family tyrosine kinase in Schwann cells. EMBO J. 1998; 17:4723–4734. PMID: 9707431.


31. Song MY, Hong C, Bae SH, So I, Park KS. Dynamic modulation of the kv2.1 channel by SRC-dependent tyrosine phosphorylation. J Proteome Res. 2012; 11:1018–1026. PMID: 22106938.


Fig. 1
IDR is enhanced under high Ca2+ condition in cultured hippocampal neurons, showing the dependence on VDCCs but not NMDARs.
(A) The experimental protocol of voltage clamping to record IDR in hippocampal neurons and example traces of IDR. High Ca2+ condition was induced by doubling Ca2+ concentration (CaCl2, 3.6 mM) of culture media for 24 hours (High Ca2+) and then neurons were washed in normal ACSF during recording. Either APV (100 µM, APV+High Ca2+) or nimodipine (10 µM, Nimodipine + High Ca2+) was added to culture media for 24 hours under high Ca2+ condition. Scale bars 500 pA, 100 ms. (B) Individuals (circles) and averaged (square bars) densities of IDR. Currents were adjusted with whole cell capacitance for measuring current densities of each group. Error bars represent SEM.
*p<0.05.

Fig. 2
The enhancement of IDR under high Ca2+ condition does not involve the significant alteration of activation properties of IDR channels.
The activation property was measured at −60 to 80 mV with 20 steps after prepulse injection (−20 mV, 200 ms duration). (A) The experimental protocol of voltage clamping to measure the activation kinetics of IDR channels and example traces of IDR. The amplitude of IDR is increased by steps of voltage clamping. However, the constant rate of increment indicates no changes of IDR activating kinetics. Scale bars 500 pA, 100 ms. (B) Averaged densities of IDR at each clamping voltage (−40 to 80 mV). (C) and (D) Boltzmann fitted curves of gating kinetics of IDR activation and averaged values of voltage half (Vh, dotted line in C). The significant change of activation curves was not observed. Error bars represent SEM.

Fig. 3
SFKs are participated in IDR enhancement under high Ca2+ condition.
(A) Example traces of IDR in each group. To confirm the contribution of SK channels, apamin (100 nM, Ca2++Apamin) was added to culture media. PP2 (1 µM) abolished the Ca2+-induced enhancement of IDR, indicating the involvement of SFK in IDR upregulation. (B) Individual (open circles) and averaged values (square bars) of IDR densities. Error bars represent SEM. *p<0.05 compared with control and †p<0.05 compared with High Ca2+. (C) Averaged densities of IDR at each clamping voltage (−40 to 80 mV). Error bars represent SEM.
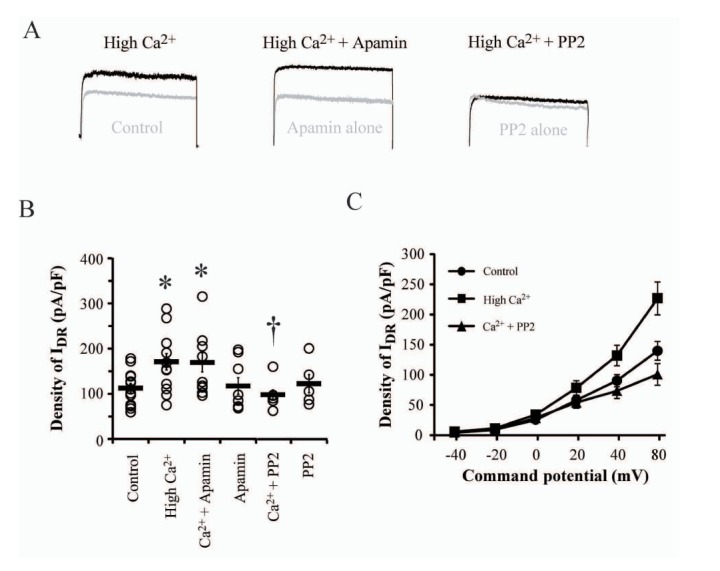